Abstract
Avian influenza (AI) vaccines should be used as part of a whole comprehensive AI control programme. Vectored vaccines based on Newcastle disease virus (NDV) are very promising, but are so far licensed in only a few countries. In the present study, the immunogenicity and protection against a highly pathogenic H5N1 influenza challenge were evaluated after vaccination with an enterotropic NDV vector expressing an H5 haemagglutinin (rNDV-H5) in 1-day-old specific pathogen free chickens inoculated once, twice or once followed by a heterologous boost with an inactivated H5N9 vaccine (iH5N9). The heterologous prime/boost rNDV-H5/iH5N9 combination afforded the best level of protection against the H5N1 challenge performed at 6 weeks of age. Two rNDV-H5 administrations conferred a good level of protection after challenge, although only a cellular H5-specific response could be detected. Interestingly, a single administration of rNDV-H5 gave the same level of protection as the double administration but without any detectable H5-specific immune response. In contrast to AI immunity, a high humoral, mucosal and cellular NDV-specific immunity could be detected up to 6 weeks post vaccination after using the three different vaccination schedules. NDV-specific mucosal and cellular immune responses were slightly higher after double rNDV-H5 vaccination when compared with single inoculation. Finally, the heterologous prime/boost rNDV-H5/iH5N9 combination induced a broader detectable immunity including systemic, mucosal and cellular AI and NDV-specific responses.
Introduction
The current H5N1 highly pathogenic (HP) avian influenza (AI) outbreak was first detected in Hong Kong in 1997 (Shortridge et al., Citation1998) and spread to Asia, Europe, and Africa. In some circumstances, well-monitored vaccination can be an additional tool for an effective control programme of AI in combination with good biosecurity practices, education about prevention, preventive culling, early diagnosis, and surveillance to detect the disease and infection. Several AI vaccines, such as inactivated (Bublot et al., Citation2007), live attenuated vectored (Bublot et al., Citation2007; Ge et al., Citation2007; Römer-Oberdörfer et al., Citation2008; Nayak et al., Citation2009; Pavlova et al., Citation2009; Ramp et al., Citation2011; Lardinois et al., Citation2012), subunit (Swayne et al., Citation2001), reassortant (Lee et al., Citation2013) or DNA vaccines (Torrieri-Dramard et al., Citation2011), have been shown to protect against HPAI, but only inactivated whole AI virus vaccines and viral vectored vaccines based on fowlpox virus or Newcastle disease virus (NDV) vectors (Swayne & Kapczynski, Citation2008) have so far been licensed in some countries.
Protection against HPAI is mainly the result of the immune response against the haemagglutinin (HA) protein, while immune responses to the neuraminidase or the internal viral proteins, such as nucleoprotein or matrix protein, are usually insufficient to provide field protection (Swayne, Citation2006). The protection is frequently assessed after experimental challenge by the prevention of death and clinical signs, the reduction in the quantity of challenge virus shed from respiratory and digestive tracts and in the percentage of shedding birds, as well as the prevention of contact transmission. It can also be evaluated indirectly through the measurement of the HA-specific antibody after vaccination and challenge (Swayne & Kapczynski, Citation2008).
The selection of AI virus (AIV) strains for inactivated vaccine manufacturing is mostly based on low-pathogenic (LP) AIV obtained from field outbreaks that have a homologous HA protein. LPAI inactivated vaccine can protect multiple species of poultry and against various HPAI viruses within the same HA subtype (Swayne, Citation2006; Bublot et al., Citation2007). A high level of HA-specific antibodies can be elicited, allowing reduced virus shedding and transmission after challenge (Bublot et al., Citation2007). Due to their widespread use, live-Newcastle disease (ND) vaccine strains have been used to generate, by reverse genetics, vectored ND vaccines expressing the HA of AIV (rNDV-HA). The potential advantages of these NDV vectored vaccines are the following: they induce a broad immunity, including mucosal, cellular and humoral immunity; they do not express the NP and M proteins, and therefore polyvalent tests such as anti-NP-based enzyme-linked immunosorbent assay (ELISA) or agar gel precipitation can be used to detect AIV infection in vaccinated animals (differentiate between infected and vaccinated animals test); they induce a rapid onset of immunity; they are bivalent (AI and ND); they can be administered by mucosal routes (spray or drinking water) and are compatible with mass administration; and their production poses less risk for the environment than inactivated vaccines in case of accidental release (Huang et al., Citation2003; van den Berg et al., Citation2008; Rauw et al., Citation2009). Several studies have demonstrated that the rNDV-AI vaccine can protect against both NDV and HPAIV challenges (Swayne et al., Citation2003; Park et al., Citation2006; Ge et al., Citation2007; Veits et al., Citation2008; Sarfati-Mizrahi et al., Citation2010). Indeed, similar to live NDV vaccines, rNDV-AI vaccines induce NDV-specific antibodies and provide excellent clinical and virological protection against velogenic NDV challenges. Most of these vaccines afforded good clinical and virological protection against homologous H5N1 HPAI challenges with minimal (Veits et al., Citation2008; Nayak et al., Citation2009; Lardinois et al., Citation2012) or high (Ge et al., Citation2007) AIV-specific antibodies. However, a lower level of protection could be observed after heterologous challenge, underlining the importance of the homology between the H5 insert vaccine and the HPAI challenge strain (Ramp et al., Citation2011; Lardinois et al., Citation2012). Finally, in all of these studies, tracheotropic genotype II NDV strains were used as the vaccinal vector with the H5 gene inserted between the P and M genes; moreover, most of the studies performed the vaccination at least at 1 week of age. Currently, as all recent NDV outbreaks declared worldwide are due to viscerotropic strains (Miller et al., Citation2007; OIE, Citation2012) and ND vaccination is frequently performed by spray at the hatchery at 1 day old, it was of great interest to investigate AI vaccination at the hatchery with an enterotropic vectored NDV vaccine expressing the AIV H5. This asymptomatic enteric strain has a great advantage to be used as a vector because this form usually consists of a subclinical enteric infection (OIE, Citation2012) and thus vaccination with an enterotropic strain should be better fitted to the field situation. Therefore, in this study, the clinical and virological protection against H5N1 HPAI strain conferred by different vaccination schedules using an enterotropic rNDV-H5 candidate inoculated at 1 day old in specific pathogen free (SPF) chickens with or without inactivated H5N9 vaccine boost at 2 weeks of age has been evaluated. Specific humoral, mucosal and cellular immune responses elicited by these different vaccination regimens were investigated and correlated to the induced AI protection levels.
Materials and Methods
Chickens
SPF White Leghorn chickens were hatched from eggs provided by Lohmann Valo (Cuxhaven, Germany). After hatching, birds were kept in biosecurity level 3 isolators and the experiments were conducted under the authorization and supervision of the Biosafety and Bioethics Committees at the Veterinary and Agrochemical Research Centre, following National and European regulations.
Vaccines and challenge strain
The rNDV-AI vectored vaccine was generated by reverse genetics from an enterotropic AVINEW® (Merial, Lyon, France) vaccine and encoding an optimized synthetic HA gene from HPAIV clade 2.2. We used the H5N1 A/turkey/Turkey/1/05 strain whose cleavage site PQGERRRKKR/GLF (HP) was mutated into PQGETR/GLF (LP) as described previously (Ferreira et al., Citation2012).
The inactivated H5N9 vaccine was an experimental vaccine as described earlier (Bublot et al., Citation2007) based on the A/Chicken/Italy/22A/98 H5N9 LPAI (cleavage site: PRNVPQKETR/GLF) inactivated antigen formulated in a water-in-oil emulsion used for commercial inactivated poultry vaccines.
The clade 2.2 H5N1 HPAI (A/Duck/Hungary/1180/2006) was kindly provided by Dr Vilmos Palfi (CVI Budapest, Hungary) and was used for challenge at the dose of 106 median embryo infective dose (EID50) per bird.
Mitogens and antigens
The mitogens phorbol 12-myristate 13-acetate (PMA) and ionomycin (Iono) were purchased from Sigma (Diegem, Belgium). NDV and H5N2 recall antigens were prepared from NDV La Sota and H5N2 LPAI (A/Chicken/Belgium/150VB/99) strains, respectively, as described previously (Lambrecht et al., Citation2004; Rauw et al., Citation2011) and were named NDV and H5N2 all proteins (prot-NDV and prot-H5N2). The NDV La Sota strain, the H5N3 LPAI (A/Teal/England/7394-2805/06) and the H5N1 HPAI (A/Duck/Hungary/1180/2006) were used to perform haemagglutination inhibition (HI) tests. The purified La Sota NDV and H5N2 LPAI (A/Chicken/Belgium/150VB/99) strains were used as coating antigens for NDV-specific and AIV-specific IgG ELISA, respectively.
It is important to note that only a small amount of HPAIV antigen (H5N1) was detected after inactivation and purification, which was not enough to be used in ELISA tests, whereas a large quantity of purified LPAI virus (H5N2) without inactivation was obtained using the same protocol (Rauw et al., Citation2011). In order to complete the characterization of H5 humoral response, the challenge strain (H5N1) and a reference strain (H5N3; Laboratory of European Union, VLA, Weybridge, UK) were used to perform the HI tests because of their high antigenic similarity. This approach, presenting two complementary tests, has previously been reported to characterize the H5 humoral response after vaccination and challenge experiments (Rauw et al., Citation2011, Citation2012).
As for analysis of NDV response, the La Sota strain is commonly used as a reference antigen in accredited tests. This strain was previously used to characterize the humoral response specific to NDV after vaccination and challenge experiments (Rauw et al., Citation2009, Citation2010).
Measurement of NDV-specific and AIV-specific humoral immunity
NDV-specific humoral immunity was evaluated by HI test as described previously (Ferreira et al., Citation2012) using 4 haemagglutination units of NDV La Sota strain per well. AIV-specific humoral immunity was evaluated using 4 haemagglutination units of H5N3 LPAI (A/Teal/England/7394-2805/06) and of H5N1 HPAI (A/Duck/Hungary/1180/2006) strains.
Measurement of NDV-specific and AIV-specific local antibody-mediated immunity in the digestive tract
The culture of intestinal tissue was conducted as described previously (Rauw et al., Citation2009). Several two-fold dilutions of supernatants were processed for testing by NDV-specific IgG ELISA (Rauw et al., Citation2009). Samples were also tested by AIV-specific IgG and IgA detection. Briefly, MaxiSorp Nunc-Immuno F96 microwell plates (International Medical, Watermaal, Belgium) were coated overnight at 4°C with purified H5N2 LPAI virus diluted at 1 µg/ml in carbonate/bicarbonate pH 9.6 buffer. After washing and blocking steps, samples were added and AIV-specific IgG and IgA was revealed using biotin-labelled mouse antibody directed against chicken IgG or IgA (SouthernBiotech, Brussels, Belgium), respectively, as used previously (Rauw et al., Citation2009).
Measurement of NDV-specific and AIV-specific cell-mediated immunity by ex vivo antigenic recall on splenic lymphocytes
The induction of NDV-specific and AIV-specific cell-mediated immunity (CMI) was evaluated by the production of ChIFNγ after ex vivo antigen activation of splenocytes as described previously (Emery et al., Citation1988). Briefly, splenic lymphocytes were activated by mitogens (PMA/Iono, 0.1 µg/ml), as positive control of ex vivo activability of lymphocytes, and by NDV and AIV recall antigens (prot-NDV and prot-H5N2, 1 µg/ml). ChIFNγ production was measured by capture ELISA as described previously (Lambrecht et al., Citation2004) and using a commercially available assay (catalogue number #CAC1233; Biosource Europe, Nivelles, Belgium). Cellular immune responses were expressed as stimulation indices (SIs) that were calculated for each bird by dividing the optical density (OD) values of mitogen-activated and antigen-activated lymphocytes by the OD of non-activated lymphocytes (Rauw et al., Citation2009), and the SIs per group were calculated. The chicken having OD < 0.1 or SI < 2 for mitogen activation was excluded from further antigen-activation analysis. OD ≥ 0.1 and SI ≥ 2 for antigen activation were considered positive for NDV/AIV-specific CMI (Emery et al., Citation1988).
Measurement of virus shedding by the oropharyngeal and cloacal routes after challenge
The oropharyngeal and cloacal swabs were harvested and RNA was extracted as described previously (Ferreira et al., Citation2012). The H5N1 HPAI RNA was detected by real-time reverse transcriptase polymerase chain reaction targeting the matrix (M) gene of avian influenza (Spackman et al., Citation2002) and quantification was done relative to a standard curve based on 10-fold dilutions of an in vitro transcribed RNA template (Steensels et al., Citation2009). The results were expressed as the number of M gene copies per millilitre of swab samples. Moreover, the quality of the sample and the RNA extraction procedure were validated using avian β-actin as described previously (Van Borm et al., Citation2007).
Experimental design
Two studies with different experimental designs were conducted using SPF chickens. The summary of the two studies is shown in . In the first trial, 40 1-day-old chicks were assigned to two groups of 20 birds each. One group, identified as the rNDV-H5/– group, was vaccinated with 105 EID50/bird of rNDV-AI by the oculonasal route using 50 µl. The second group was left untreated to be used as unvaccinated negative controls and was identified as the –/– group. Four chickens per group were randomly euthanized on a weekly basis during 3 weeks, namely at 2, 3, and 4 weeks post vaccination (p.v.). The blood, spleen and duodenum were collected to evaluate the systemic, cellular and mucosal immune responses. At 4 weeks p.v., eight chickens from each group were individually identified and challenged with 106 EID50 of H5N1 HPAI (A/Duck/Hungary/1180/2006) via the oculonasal route. Birds were monitored daily for clinical signs of disease and mortality was recorded over a 2-week period. Oropharyngeal and cloacal swabs were taken at 2, 4 and 7 days post challenge (p.c.) and blood was taken from the surviving chickens at 2 weeks p.c.
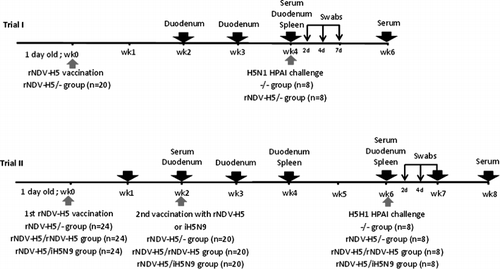
In the second trial, two vaccination schemes involving two successive vaccine administrations were tested. Ninety-six SPF 1-day-old chickens were split into four groups of 24 birds. Three groups were vaccinated with 105 EID50 of rNDV-H5. After 2 weeks, chickens were boosted with the rNDV-H5 at the same dose by the oculonasal route (homologous prime/boost) or with one dose of the H5N9 (A/Chicken/Italy/22A/1998) inactivated vaccine (heterologous prime/boost) by the subcutaneous route and identified as the rNDV-H5/rNDV-H5 group and the rNDV-H5/iH5N9 group, respectively. Chickens of the third group, namely the rNDV-H5/– group, were not boosted at 2 weeks, while unvaccinated chickens were used as the control group (–/–group). Four birds per group were randomly euthanized on a weekly basis during 4 weeks, namely at 2, 3, 4 and 6 weeks after the first vaccination.
In both trials, the blood, spleen and duodenum were collected to evaluate the immune responses. The mucosal and cellular responses were not evaluated in birds from the rNDV-H5/– group (Trial II) due to the limited number of birds obtained for the experiment and also as these responses were evaluated in Trial I, 2, 3 and 4 weeks after prime vaccination. At 6 weeks p.v., eight chickens per group were individually identified with rings numbered 1 to 8 and challenged with 106 EID50 of H5N1 HPAI (A/Duck/Hungary/1180/2006) by the oculonasal route. Protection against mortality and clinical signs, virus shedding and serology were evaluated as described above.
Statistical analysis
One-way analysis of variance and the Student t test were carried out to compare the different groups, and the differences were considered significant at P < 0.05.
Accession numbers
The GenBank accession number for the complete genome sequence of enterotropic AVINEW® vaccine is KC906188. The GenBank accession number of the NDV La Sota strain is AJ629062. The GenBank accession number of the HA sequence from clade 2.2 H5N1 A/turkey/Turkey/1/05 strain is HB416506. The GISAID accession numbers of clade 2.2 H5N1 A/Duck/Hungary/1180/2006 strain are EPI_ISL_64353 and EPI_ISL_63778. The GISAID accession number of the H5N2 A/Chicken/Belgium/150VB/99 strain is EPI_ISL_26340. The AHVLA scientific accession number of the H5N3 A/Teal/England/7394-2805/06 strain is RAA7008.
Results
Protection against morbidity and mortality
The efficacy of the rNDV-H5 vaccines used alone or included in a homologous or heterologous prime/boost vaccination scheme was evaluated and compared for protection against mortality and clinical signs after challenge with the H5N1 HPAI (A/Duck/Hungary/1180/2006) strain. The unvaccinated birds showed clinical signs such as depression and conjunctivitis at 2 days p.c. and all birds died by days 3 and 4 p.c. when challenged at 4 and 6 weeks, respectively, validating the trial (). Single rNDV-H5 vaccination resulted in full (8/8) protection against mortality and clinical signs after challenge at 4 weeks p.v., while 7/8 (88%) of the chickens of the rNDV-H5/– group were protected when the challenge was performed at 6 weeks of age. A second rNDV-H5 administration at 2 weeks of age did not improve the protection against mortality (7/8 birds), while a boost with the H5N9 inactivated vaccine induced full (8/8 birds) protection against mortality. No clinical signs were observed among vaccinated birds in all groups, and an even mortality was reported.
Table 1. Protection against morbidity and mortality after challenge with the H5N1 HPAI (A/Duck/Hungary/1180/2006) strain in vaccinated SPF chickens.
Virus excretion after challenge
Viral shedding was monitored by real-time reverse transcriptase polymerase chain reaction in the oropharyngeal and cloacal swabs and observed at 2 days p.c. in the unvaccinated group (). The levels of viral RNA detected in the oropharynx were significantly (P < 0.05) reduced in the three vaccinated groups, regardless of the time of challenge. Interestingly, at 2 days p.c. a booster vaccination with the H5N9 inactivated vaccine reduced the virus excretion significantly (P < 0.05), when compared with the single rNDV-H5 vaccination. However, the level of oropharyngeal shedding after challenge was similar after single or double rNDV-H5 vaccination. No virus was excreted by the cloacal route in any vaccinated group regardless of the time of challenge.
Table 2. Virus shedding after challenge at 4 and 6 weeks p.v. with the H5N1 HPAI (A/Duck/Hungary/1180/2006) strain in vaccinated SPF chickens.
Humoral immunity
The serological titres in all unvaccinated chickens were below the threshold of positivity (≤ 23), regardless of the HA antigen used. The rNDV-H5 vaccine induced NDV-specific HI antibody from the second week p.v. with a mean titre of 26 (ai,aii), confirming the rNDV-H5 vaccine uptake. Indeed, all the chickens of the rNDV-H5/–, rNDV-H5/rNDV-H5 and rNDV-H5/iH5N9 groups were positive when tested with the La Sota NDV HA antigen. No NDV boost effect was observed after a second inoculation of the rNDV-H5 vaccine at 2 weeks of age, as shown by no significant difference between the rNDV-H5/– and rNDV-H5/rNDV-H5 groups at 6 weeks (aii). As expected, no interference on the NDV-specific humoral immunity was induced by the heterologous vaccination with the H5N9 inactivated vaccine.
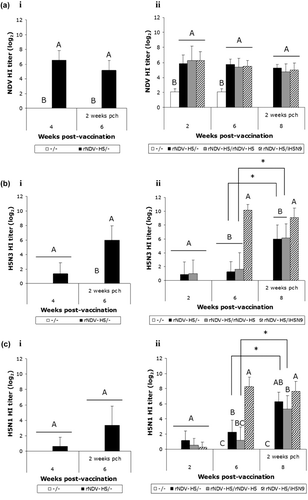
Only 1/8 of the rNDV-H5/– vaccinated chickens was AI-positive (average of 25) using the H5N3 LPAI and the H5N1 HPAI HA antigens (bi,ci) and the mean titres remained under the threshold of positivity regardless of the time post vaccination (2, 4 and 6 week p.v.). Following rNDV-H5 boost, the antibody titres did not increase in the rNDV-H5/rNDV-H5 group and the mean titres were not significantly different when compared with the single vaccinated group. These positive results were confirmed in a commercial ELISA AI kit specific to H5 (ID-VET, Montpellier, France), with only HI-positive sera found to be positive for the H5-specific ELISA (data not shown). All rNDV-H5/iH5N9 vaccinated chickens showed positive HI titres at 6 weeks when tested with both H5 antigens. This AIV-specific humoral immunity (average of 210) was significantly higher (P < 0.05) than the one measured in the unvaccinated and the other vaccinated groups ( bii,cii).
After challenge, a significant increase (P < 0.05) in the HI titres was observed for the rNDV-H5/– and rNDV-H5/rNDV-H5 groups when tested with the H5N3 LPAI and the H5N1 HPAI HA antigens, indicating viral replication. The challenge had no effect on the HI titres in the rNDV-H5/iH5N9 vaccinated chickens, regardless of the HA antigen used.
Local antibody-mediated immunity in the digestive tract
Supernatants from ex vivo duodenal tissue culture of vaccinated chickens were collected at different time-points to assess local NDV-specific and AIV-specific antibody-mediated immunity in the digestive tract (). NDV-specific IgGs were detected as early as 2 weeks post priming in the rNDV-H5/rNDV-H5 group in Trial II. The rNDV-H5/– and rNDV-H5/iH5N9 groups were not tested at this time point (see Experimental design in the Materials and Methods section). This local immunity was significantly higher (P < 0.05) in the rNDV-H5/iH5N9 group just 1 week after boost (third week p.v.; aii) and in the rNDV-H5/ rNDV-H5 group at 2 weeks after second inoculation (fourth week p.v.; aii), when compared with that of the unvaccinated chickens.
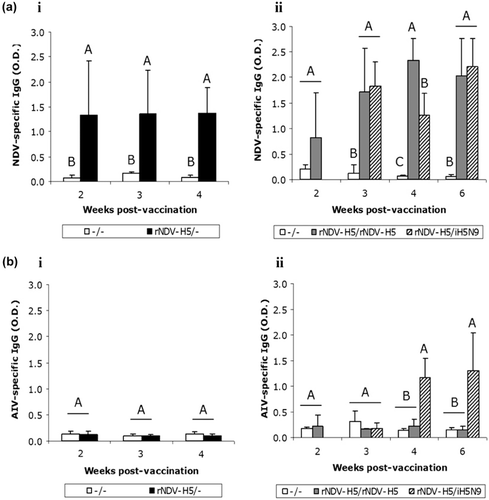
AI-specific IgG-mediated immunity could only be detected in the digestive tract of the rNDV-H5/iH5N9 group and this was significantly higher (P < 0.05) than that of the unvaccinated group from the second week after the inactivated boost (bii). The single or double rNDV-H5 vaccination did not induce a measurable local AIV-specific immunity over the 6-week period investigated in the present study. No AIV-specific IgA was detected in any of the vaccinated groups (bi,bii).
Cell-mediated immunity
The splenocyte activation with PMA/Iono mitogens showed that all chickens in each group were immunocompetent (OD > 0.1 and SI > 2), validating the spleen cell activability (data not shown). The single vaccination with the rNDV-H5 vaccine (Trial I) induced a measurable level of NDV-specific cell-mediated immunity at 4 weeks p.v. (i). This cellular immune response was enhanced by second inoculation of the rNDV-H5, as shown by the higher value of the SI at 4 weeks in the rNDV-H5/rNDV-H5 group in Trial II, when compared with the rNDV-H5/– (Trial I) and rNDV-H5/iH5N9 (Trial II) groups (ii). The NDV-specific CMI decreased at 6 weeks p.v. in both the rNDV-H5/rNDV-H5 and rNDV-H5/iH5N9 groups, while the rNDV-H5/– group was not tested at this time point (see Experimental design in the Material and Methods section).
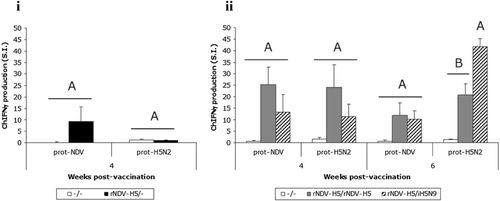
A significantly higher (P < 0.05) H5-specific CMI was observed in both rNDV-H5/rNDV-H5 and rNDV-H5/iH5N9 groups at 4 and 6 weeks p.v., when compared with that of the unvaccinated group. The kinetic profile differed depending on the vaccination scheme, with the rNDV-H5/rNDV-H5 group inducing an overall higher cellular response at 4 weeks p.v., whereas the rNDV-H5/iH5N9 vaccination regimen induced higher H5-specific CMI at 6 weeks p.v. No H5-specific cellular immune response could be detected at 4 weeks p.v. after a single vaccination with the rNDV-H5 vaccine in Trial I.
Discussion
H5N1 HPAI infection has devastating consequences to the poultry industry and several vaccination strategies have been described to improve control of AI, in combination with good biosecurity and prevention practices. In particular, vaccines with easier and lower cost of administration are urgently needed. In this context, NDV vector is currently underway for mass immunization against AI (Veits et al., Citation2006, Citation2008; Ge et al., Citation2007; Nayak et al., Citation2009; Sarfati-Mizrahi et al., Citation2010). Previous studies showed that rNDV-H5 provides good levels of protection against mortality in SPF chickens with a single administration (Veits et al., Citation2006, Citation2008; Ge et al., Citation2007; Nayak et al., Citation2009; Sarfati-Mizrahi et al., Citation2010), although the level of protection could be influenced by the homology between the H5 sequence of the vaccine insert and the HPAI challenge virus (Römer-Oberdörfer et al., Citation2008).
The present work showed for the first time that a single 1-day-old administration of 105 EID50 enterotropic rNDV-H5 vaccine provided complete protection against clinical signs and mortality after infection with H5N1 HPAI at 4 weeks of age, while previous studies showed that 106 EID50 tracheotropic rNDV-H5 vaccine was protective at 3 weeks p.v. when chickens were inoculated at 1 day old or 1 week or 3 weeks old (Veits et al., Citation2006, Citation2008; Ge et al., Citation2007; Nayak et al., Citation2009; Sarfati-Mizrahi et al., Citation2010; Ramp et al., Citation2011; Lardinois et al., Citation2012). Nevertheless, one out of eight birds that had received a single 1-day-old rNDV-H5 vaccination died at 6 weeks of age in the present study, suggesting that the immunity induced by a single 1-day-old rNDV-H5 vaccination may not be long-lasting. Prime/boost vaccination schemes at 1 day/2 weeks of age were investigated and the programme including a boost with an inactivated H5N9 vaccine showed protection against morbidity and mortality. The homologous prime/boost with the rNDV-H5 vaccine did not improve the protection against morbidity nor mortality when compared with single rNDV-H5 administration. The improved protection induced by the rNDV-H5/iH5N9 vaccination scheme was in line with a higher humoral immune response, confirming that the antibody response plays a major role in the protection against AI, especially the protection induced by inactivated vaccine (Swayne & Kapczynski, Citation2008). Several studies demonstrated that inactivated vaccines can elicit strong systemic immune response preventing the mortality and the morbidity of the chickens when challenged with the H5N1 HPAIVs (Swayne et al., Citation2006; Bublot et al., Citation2007; Terregino et al., Citation2007; Isoda et al., Citation2008; Tian et al., Citation2010) and that this protective immunity can remain up to 138 weeks (Sasaki et al., Citation2009). Indeed, a very recent study showed that bivalent vaccine containing inactivated La Sota strain of NDV and reassortant highly pathogenic AI H5N1 virus at 106 EID50 and 107.5 EID50, respectively, induced high HI titres and afforded complete clinical and shedding protection after 3 weeks p.v. at 6 weeks of age in SPF chickens (Lee et al., Citation2013). In this study, the HI titres against homologous antigen were higher than those against heterologous antigen. Interestingly, the HI antibody titres against the H5N3 antigen, which is phylogenically closer to the H5N9 antigen included in the inactivated vaccine used in this study, were higher in the rNDV-H5/iH5N9 vaccinated chickens than historical values observed after a single inactivated H5N9 vaccine inoculation (Bublot et al., Citation2007), suggesting an efficient priming effect of the rNDV-H5 vaccine at 1 day old. Moreover, the cross-reactivity against H5N1 antigen of the serums from rNDV-H5/iH5N9 vaccinated birds was higher than those usually observed after a single vaccination with the inactivated H5N9 vaccine (Bublot et al., Citation2007). This could be explained by the origin of the HA gene inserted in the NDV vector originating from the H5N1 A/Turkey/1/2005 strain, which has higher identity to the Hungarian clade 2 H5N1 challenge antigen. Similar broader humoral immunity was observed previously after heterologous prime/boost vaccination scheme using a fowlpox vector (Steensels et al., Citation2009). This may be due to the use of two different haemagglutinins in the prime and the boost vaccines, the immune response after the second vaccination being directed only against the cross-reactive epitopes conserved between the two HAs (Bublot et al., Citation2008; van den Berg et al., Citation2008; Wang et al., Citation2010).
In our study, the single inoculation with the rNDV-H5 vaccine induced no or little AI-specific systemic antibody, while a strong humoral immune response was detected 3 weeks after vaccination by others (Veits et al., Citation2006; Ge et al., Citation2007; Nayak et al., Citation2009; Sarfati-Mizrahi et al., Citation2010). Interestingly, a recent study found a very low humoral immune response in one out of three experiments with rNDV-H5 using a tracheotropic strain whilst in the other experiments no AI-specific antibody was detected after vaccination in 1-day-old chickens (Lardinois et al., Citation2012). This could be related to the age of the chickens at vaccination and a lower maturity of their immune system at 1 day old. A previous study showed a lower and slower antibody production after immunization of 1-day-old chickens compared with immunization at 1 or 2 weeks old (Mast & Goddeeris, Citation1999). Surprisingly, a second administration of rNDV-H5 at 2 weeks did not increase the AI-specific humoral immunity. Such absence of AI-specific antibody after a second rNDV-H5 administration has also been observed by others (Römer-Oberdörfer et al., Citation2008). Nevertheless, most of the birds vaccinated once or twice with rNDV-H5 were protected despite the absence of detectable humoral response. This indicates that other immune mechanisms in the host must be involved in the rNDV-H5-induced clinical and shedding protection.
The induction of a potent virus-specific immune response at the mucosal surfaces, as observed in mice after mucosal administration of a DNA vaccine, was able to reduce the AIV replication in chickens (Torrieri-Dramard et al., Citation2011). Similarly, in our study, one or two administration(s) of rNDV-H5 induced a high IgG-mediated immunity against NDV in the digestive tract, which could be related to the enterotropic nature of the rNDV-H5 vaccine (Rauw et al., Citation2009). Nevertheless, no gut-associated AIV-specific antibody-mediated immunity could be detected after single or double vaccination with rNDV-H5, while the rNDV-H5/iH5N9 prime/boost vaccination scheme induced high AIV-specific IgG from 4 weeks of age. This duodenal AIV-specific IgG could originate from serum by transudation (Muir et al., Citation2000; Ferreira et al., Citation2010) and may partially explain the absence of virus shedding by the cloacal route. The same phenomenon might also occur in the respiratory tract, which could explain the lowest levels of virus shedding observed in the heterologous prime/boost group.
Recent studies have reported that AI protection might not only be correlated with the levels of circulating antibodies (Thomas et al., Citation2006; Zhong et al., Citation2010) but also with the cellular immune response, as shown in mice after vaccination with AI inactivated or DNA vaccines (Hovden et al., Citation2009; Torrieri-Dramard et al., Citation2011). The present study shows for the first time that NDV and AI-specific CMI could be induced by the rNDV-H5 vaccine. Interestingly, both specific cellular immune responses seemed higher after the double rNDV-H5 vaccination than after the single inoculation, contrary to what was observed for the serological systemic and local immune responses. Moreover, the AI-specific cellular immune response was higher at 4 weeks in the group vaccinated twice with the rNDV-H5 vaccine in comparison with the group immunized with the rNDV-H5/iH5N9 vaccination scheme. Conversely, at 6 weeks of age, the CMI in the second group was higher than in the first group. Whether the more rapid induction of detectable CMI corresponds to an earlier onset of immunity after two vaccinations with rNDV-H5 compared with the heterologous prime/boost requires further investigation. In addition, the immune mechanisms involved after single or double rNDV-H5 inoculation that induces protection despite the absence of significant detectable AIV-specific antibody and/or only cellular response remains unclear.
Vaccination with live-ND vaccines is frequently primed by spray at the hatchery at 1 day old, often combined with live vaccine against infectious bronchitis. Our results indicate for the first time that 1-day-old mucosal administration of rNDV-H5 induced good levels of protection despite the absence of detectable AI-specific antibody. As for protection against NDV challenge, it is well known that the HI titre against NDV obtained after vaccination could be used as an indicator of the potential shedding protection induced by vaccination (Allan et al., Citation1978; Westbury et al., Citation1984). It remains to be investigated whether high levels (26) of NDV-specific antibodies detected from 2 week p.v. that remained stable up to 8 weeks p.v. (completion of the experiment here) can provide a high level of protection against NDV strains. In endemic countries, breeders are heavily vaccinated against both AI and ND. The potential interference of AIV-specific and NDV-specific maternal-derived antibodies on rNDV-H5 prime/boost vaccination efficacy will need further assessment. Boosting the rNDV-H5 immune response by an inactivated vaccine clearly increased AIV-specific immunity as well as the level of AI protection. The higher and broader protection induced by a heterologous prime/boost scheme observed here with a NDV vector confirmed previous observations reported with fowlpox vector (Bublot et al., Citation2008; Steensels et al., Citation2009; Wei et al., Citation2010). Our results suggest that this high level of protection might be achieved by inducing a combination of systemic and mucosal antibody as well as cellular immune responses. Further new immunological tools should be developed to allow a better characterization of the immune response induced by new vector vaccine candidates and understanding of the protection mechanisms required to control HPAI.
Acknowledgements
The authors are thankful to Sophie Lemaire, Martine Gonze and Stéphanie Lagrange for their excellent technical assistance. They are grateful to Christophe Delgrange and Marc Vandenbroeck for bird handling and sampling assistance. They also thank Vilmos Palfi for providing the HPAI challenge strain. AVINEW® is a registered trademark of Merial. All other marks are the property of their respective owners. This work was performed within the European NOVADUCK project supported by the European Commission within the FP6 programme (number: SSPE-CT-2006-44217).
References
- Allan, W.H., Lancaster, J.E. & Toth, B. (1978). Newcastle Disease Vaccines, Their Production and Use. ( 163). Rome: FAO Animal Production Series.
- Bublot, M., Karaca, K., Minke, J., Pritchard, N., Le Gros, F., Poulet, H., Audonnet, J.C., Swayne, D.E. & Nordgren, R. (2008). Current influenza vaccines for poultry and other animal species. In R.A. Trip (Ed). Immunobiology of Influenza Infection: Approaches for an Emerging Zoonotic Disease (pp. 117–136). Trivandrum: Research SignPost.
- Bublot, M., Le Gros, F.X., Nieddu, D., Pritchard, N., Mickle, T.R. & Swayne, D.E. (2007). Efficacy of two H5N9-inactivated vaccines against challenge with a recent H5N1 highly pathogenic avian influenza isolate from a chicken in Thailand. Avian Diseases, 51, 332–337. 10.1637/7623-042706R.1
- Bublot, M., Pritchard, N., Cruz, J.S., Mickle, T.R., Selleck, P. & Swayne, D.E. (2007). Efficacy of a fowlpox-vectored avian influenza H5 vaccine against Asian H5N1 highly pathogenic avian influenza virus challenge. Avian Diseases, 51, 498–500. 10.1637/7624-042706R.1
- Emery, D.L., Dufty, J.H. & Wood, P.R. (1988). An analysis of cellular proliferation, and synthesis of lymphokines and specific antibody in vitro by leucocytes from immunized cattle. Veterinay Immunology Immunopathology, 18, 67–80. 10.1016/0165-2427(88)90037-2
- Ferreira, H.L., Pirlot, J.F., Kaspers, B., Kothlow, S., van den Berg, T. & Lambrecht, B. (2010). Development of specific enzyme-linked immunosorbent assays to evaluate the duck immune response after experimental infection with H5N1 and H7N1 low-pathogenicity avian influenza viruses. Avian Diseases, 54, 660–667. 10.1637/8753-033109-ResNote.1
- Ferreira, H.L., Pirlot, J.F., Reynard, F., van den Berg, T., Bublot, M. & Lambrecht, B. (2012). Immune responses and protection against H5N1 highly pathogenic avian influenza virus induced by the Newcastle disease virus H5 vaccine in ducks. Avian Diseases, 56, 940–948. 10.1637/10148-040812-ResNote.1
- Ge, J., Deng, G., Wen, Z., Tian, G., Wang, Y., Shi, J., Wang, X., Li, Y., Hu, S., Jiang, Y., Yang, C., Yu, K., Bu, Z. & Chen, H. (2007). Newcastle disease virus-based live attenuated vaccine completely protects chickens and mice from lethal challenge of homologous and heterologous H5N1 avian influenza viruses. Journal of Virology, 81, 150–158. 10.1128/JVI.01514-06
- Hovden, A.O., Brokstad, K.A., Major, D., Wood, J., Haaheim, L.R. & Cox, R.J. (2009). A pilot study of the immune response to whole inactivated avian influenza H7N1 virus vaccine in mice. Influenza and Other Respiratory Viruses, 3, 21–28. 10.1111/j.1750-2659.2009.00075.x
- Huang, Z., Elankumaran, S., Panda, A. & Samal, S.K. (2003). Recombinant Newcastle disease virus as a vaccine vector. Poultry Science, 82, 899–906.
- Isoda, N., Sakoda, Y., Kishida, N., Soda, K., Sakabe, S., Sakamoto, R., Imamura, T., Sakaguchi, M., Sasaki, T., Kokumai, N., Ohgitani, T., Saijo, K., Sawata, A., Hagiwara, J., Lin, Z. & Kida, H. (2008). Potency of an inactivated avian influenza vaccine prepared from a non-pathogenic H5N1 reassortant virus generated between isolates from migratory ducks in Asia. Archives of Virology, 153, 1685–1692. 10.1007/s00705-008-0171-1
- Lambrecht, B., Gonze, M., Meulemans, G. & van den Berg, T.P. (2004). Assessment of the cell-mediated immune response in chickens by detection of chicken interferon-gamma in response to mitogen and recall Newcastle disease viral antigen stimulation. Avian Pathology, 33, 343–350. 10.1080/0307945042000220318
- Lardinois, A., Steensels, M., Lambrecht, B., Desloges, N., Rahaus, M., Rebeski, D. & van den Berg, T. (2012). Potency of a recombinant NDV-H5 vaccine against various HPAI H5N1 virus challenges in SPF chickens. Avian Diseases, 56, 928–936. 10.1637/10173-041012-ResNote.1
- Lee, D.H., Park, J.K., Kwon, J.H., Yuk, S.S., Erdene-Ochir, T.O., Jang, Y.H., Seong, B.L., Lee, J.B., Park, S.Y., Choi, I.S. & Song, C.S. (2013). Efficacy of single dose of a bivalent vaccine containing inactivated Newcastle disease virus and reassortant highly pathogenic avian influenza H5N1 virus against lethal HPAI and NDV infection in chickens. PLoS One, 8, e58186. 10.1371/journal.pone.0058186
- Mast, J. & Goddeeris, B.M. (1999). Development of immunocompetence of broiler chickens. Veterinary Immunology and Immunopathology, 70, 245–256. 10.1016/S0165-2427(99)00079-3
- Miller, P.J., King, D.J., Afonso, C.L. & Suarez, D.L. (2007). Antigenic differences among Newcastle disease virus strains of different genotypes used in vaccine formulation affect viral shedding after a virulent challenge. Vaccine, 25, 7238–7246. 10.1016/j.vaccine.2007.07.017
- Muir, W.I., Bryden, W.L. & Husband, A.J. (2000). Immunity, vaccination and the avian intestinal tract. Developmental & Comparative Immunology, 24, 325–342. 10.1016/S0145-305X(99)00081-6
- Nayak, B., Rout, S.N., Kumar, S., Khalil, M.S., Fouda, M.M., Ahmed, L.E., Earhart, K.C., Perez, D.R., Collins, P.L. & Samal, S.K. (2009) Immunization of chickens with Newcastle disease virus expressing H5 hemagglutinin protects against highly pathogenic H5N1 avian influenza viruses. PLoS One, 4, e6509. 10.1371/journal.pone.0006509
- OIE (2012). Chapter 10.9. Newcastle Disease. In Terrestrial Animal Health Code. 21st edn. Paris: OIE.
- Park, M.S., Steel, J., Garcia-Sastre, A., Swayne, D. & Palese, P. (2006). Engineered viral vaccine constructs with dual specificity: avian influenza and Newcastle disease. Proceedings of the National Academy of Sciences USA, 103, 8203–8208. 10.1073/pnas.0602566103
- Pavlova, S.P., Veits, J., Mettenleiter, T.C. & Fuchs, W. (2009). Live vaccination with an H5-hemagglutinin-expressing infectious laryngotracheitis virus recombinant protects chickens against different highly pathogenic avian influenza viruses of the H5 subtype. Vaccine, 27, 5085–5090. 10.1016/j.vaccine.2009.06.048
- Ramp, K., Veits, J., Deckers, D., Rudolf, M., Grund, C., Mettenleiter, T.C. & Romer-Oberdorfer, A. (2011). Coexpression of avian influenza virus H5 and N1 by recombinant Newcastle disease virus and the impact on immune response in chickens. Avian Diseases, 55, 413–421. 10.1637/9652-011111-Reg.1
- Rauw, F., Anbari, S., van den Berg, T. & Lambrecht, B. (2011). Measurement of systemic and local respiratory cell-mediated immunity after influenza infection in chickens. Veterinary Immunology and Immunopathology, 143, 27–37. 10.1016/j.vetimm.2011.05.029
- Rauw, F., Gardin, Y., Palya, V., Anbari, S., Lemaire, S., Boschmans, M., van den Berg, T. & Lambrecht, B. (2010). Improved vaccination against Newcastle disease by an in ovo recombinant HVT-ND combined with an adjuvanted live vaccine at day-old. Vaccine, 28, 823–833. 10.1016/j.vaccine.2009.10.049
- Rauw, F., Gardin, Y., Palya, V., Van Borm, S., Gonze, M., Lemaire, S. & van den Berg, T. (2009). Humoral, cell-mediated and mucosal immunity induced by oculo-nasal vaccination of one-day-old SPF and conventional layer chicks with two different live Newcastle Disease vaccines. Vaccine, 27, 3631–3642. 10.1016/j.vaccine.2009.03.068
- Rauw, F., Palya, V., Gardin, Y., Tatar-Kis, T., Dorsey, K.M., Lambrecht, B. & van den Berg, T. (2012). Efficacy of rHVT-AI vector vaccine in broilers with passive immunity against challenge with two antigenically divergent Egyptian clade 2.2.1 HPAI H5N1 strains. Avian Diseases, 56, 913–922. 10.1637/10172-041012-Reg.1
- Rauw, F., Palya, V., Van Borm, S., Welby, S., Tatar-Kis, T., Gardin, Y., Dorsey, K.M., Aly, M.M., Hassan, M.K., Soliman, M.A., Lambrecht, B. & van den Berg, T. (2011). Further evidence of antigenic drift and protective efficacy afforded by a recombinant HVT-H5 vaccine against challenge with two antigenically divergent Egyptian clade 2.2.1 HPAI H5N1 strains. Vaccine, 29, 2590–2600. 10.1016/j.vaccine.2011.01.048
- Römer-Oberdörfer, A., Veits, J., Helferich, D. & Mettenleiter, T.C. (2008). Level of protection of chickens against highly pathogenic H5 avian influenza virus with Newcastle disease virus based live attenuated vector vaccine depends on homology of H5 sequence between vaccine and challenge virus. Vaccine, 26, 2307–2313. 10.1016/j.vaccine.2008.02.061
- Sarfati-Mizrahi, D., Lozano-Dubernard, B., Soto-Priante, E., Castro-Peralta, F., Flores-Castro, R., Loza-Rubio, E. & Gay-Gutierrez, M. (2010). Protective dose of a recombinant Newcastle disease LaSota-avian influenza virus H5 vaccine against H5N2 highly pathogenic avian influenza virus and velogenic viscerotropic Newcastle disease virus in broilers with high maternal antibody levels. Avian Diseases, 54, 239–241. 10.1637/8735-032509-Reg.1
- Sasaki, T., Kokumai, N., Ohgitani, T., Sakamoto, R., Takikawa, N., Lin, Z., Okamatsu, M., Sakoda, Y. & Kida, H. (2009). Long lasting immunity in chickens induced by a single shot of influenza vaccine prepared from inactivated non-pathogenic H5N1 virus particles against challenge with a highly pathogenic avian influenza virus. Vaccine, 27, 5174–5177. 10.1016/j.vaccine.2009.06.070
- Shortridge, K.F., Zhou, N.N., Guan, Y., Gao, P., Ito, T., Kawaoka, Y., Kodihalli, S., Krauss, S., Markwell, D., Murti, K.G., Norwood, M., Senne, D., Sims, L., Takada, A. & Webster, R.G. (1998). Characterization of avian H5N1 influenza viruses from poultry in Hong Kong. Virology, 252, 331–342. 10.1006/viro.1998.9488
- Spackman, E., Senne, D.A., Myers, T.J., Bulaga, L.L., Garber, L.P., Perdue, M.L., Lohman, K., Daum, L.T. & Suarez, D.L. (2002). Development of a real-time reverse transcriptase PCR assay for type A influenza virus and the avian H5 and H7 hemagglutinin subtypes. Journal of Clinical Microbiology, 40, 3256–3260. 10.1128/JCM.40.9.3256-3260.2002
- Steensels, M., Bublot, M., Van Borm, S., De Vriese, J., Lambrecht, B., Richard-Mazet, A., Chanavat-Bizzini, S., Duboeuf, M., Le Gros, F.-X. & van den Berg, T. (2009). Prime-boost vaccination with a fowlpox vector and an inactivated avian influenza vaccine is highly immunogenic in Pekin ducks challenged with Asian H5N1 HPAI. Vaccine, 27, 646–654. 10.1016/j.vaccine.2008.11.044
- Swayne, D.E. (2006). Principles for vaccine protection in chickens and domestic waterfowl against avian influenza: emphasis on Asian H5N1 high pathogenicity avian influenza. Annals of the New York Academy of Sciences, 1081, 174–181. 10.1196/annals.1373.021
- Swayne, D.E., Beck, J.R., Perdue, M.L. & Beard, C.W. (2001). Efficacy of vaccines in chickens against highly pathogenic Hong Kong H5N1 avian influenza. Avian Diseases, 45, 355–365. 10.2307/1592975
- Swayne, D.E. & Kapczynski, D.R. (2008). Vaccines, vaccination, and immunology for avian influenza viruses in poultry. In D. Swayne (Ed.). Avian Influenza. (pp. 407–451). Ames: Blackwell Publishing.
- Swayne, D.E., Lee, C.W. & Spackman, E. (2006). Inactivated North American and European H5N2 avian influenza virus vaccines protect chickens from Asian H5N1 high pathogenicity avian influenza virus. Avian Pathology, 35, 141–146. 10.1080/03079450600597956
- Swayne, D.E., Suarez, D.L., Schultz-Cherry, S., Tumpey, T.M., King, D.J., Nakaya, T., Palese, P. & Garcia-Sastre, A. (2003). Recombinant paramyxovirus type 1-avian influenza-H7 virus as a vaccine for protection of chickens against influenza and Newcastle disease. Avian Diseases, 47, 1047–1050. 10.1637/0005-2086-47.s3.1047
- Terregino, C., Toffan, A., Beato, M.S., De Nardi, R., Drago, A. & Capua, I. (2007). Conventional H5N9 vaccine suppresses shedding in specific-pathogen-free birds challenged with HPAI H5N1 A/Chicken/Yamaguchi/7/2004. Avian Diseases, 51, 495–497. 10.1637/7565-033106R.1
- Thomas, P.G., Keating, R., Hulse-Post, D.J. & Doherty, P.C. (2006). Cell-mediated protection in influenza infection. Emerging infectious diseases, 12, 48–54. 10.3201/eid1201.051237
- Tian, G., Zeng, X., Li, Y., Shi, J. & Chen, H. (2010). Protective efficacy of the H5 inactivated vaccine against different highly pathogenic H5N1 avian influenza viruses isolated in China and Vietnam. Avian Diseases, 54, 287–289. 10.1637/8707-031709-ResNote.1
- Torrieri-Dramard, L., Lambrecht, B., Ferreira, H.L., van den Berg, T., Klatzmann, D. & Bellier, B. (2011). Intranasal DNA vaccination induces potent mucosal and systemic immune responses and cross-protective immunity against influenza viruses. Molecular Therapy, 19, 602–611. 10.1038/mt.2010.222
- Van Borm, S., Steensels, M., Ferreira, H.L., Boschmans, M., De Vriese, J., Lambrecht, B. & van den Berg, T. (2007). A universal avian endogenous real-time reverse transcriptase-polymerase chain reaction control and its application to avian influenza diagnosis and quantification. Avian Diseases, 51, 213–220. 10.1637/7552-033106R.1
- van den Berg, T., Lambrecht, B., Marche, S., Steensels, M., Van Borm, S. & Bublot, M. (2008). Influenza vaccines and vaccination strategies in birds. Comparative Immunology, Microbiology & Infectious Diseases, 31, 121–165.
- Veits, J., Romer-Oberdorfer, A., Helferich, D., Durban, M., Suezer, Y., Sutter, G. & Mettenleiter, T.C. (2008). Protective efficacy of several vaccines against highly pathogenic H5N1 avian influenza virus under experimental conditions. Vaccine, 26, 1688–1696. 10.1016/j.vaccine.2008.01.016
- Veits, J., Wiesner, D., Fuchs, W., Hoffmann, B., Granzow, H., Starick, E., Mundt, E., Schirrmeier, H., Mebatsion, T., Mettenleiter, T.C. & Romer-Oberdorfer, A. (2006). Newcastle disease virus expressing H5 hemagglutinin gene protects chickens against Newcastle disease and avian influenza. Proceedings of the National Academy of Sciences USA, 103, 8197–8202. 10.1073/pnas.0602461103
- Wang, T.T., Tan, G.S., Hai, R., Pica, N., Petersen, E., Moran, T.M. & Palese, P. (2010). Broadly protective monoclonal antibodies against H3 influenza viruses following sequential immunization with different hemagglutinins. PLoS Pathogens, 6, 1–9.
- Wei, C.J., Boyington, J.C., McTamney, P.M., Kong, W.P., Pearce, M.B., Xu, L., Andersen, H., Rao, S., Tumpey, T.M., Yang, Z.Y. & Nabel, G.J. (2010). Induction of broadly neutralizing H1N1 influenza antibodies by vaccination. Science, 329, 1060–1064. 10.1126/science.1192517
- Westbury, H.A., Parsons, G. & Allan, W.H. (1984). Duration of excretion of virulent Newcastle disease virus following challenge of chickens with different titres of serum antibody to the virus. Australian Veterinary Journal, 61, 44–46. 10.1111/j.1751-0813.1984.tb07189.x
- Zhong, W., Liu, F., Dong, L., Lu, X., Hancock, K., Reinherz, E.L., Katz, J.M. & Sambhara, S. (2010). Significant impact of sequence variations in the nucleoprotein on CD8 T cell-mediated cross-protection against influenza A virus infections. PLoS One, 5, e10583. 10.1371/journal.pone.0010583