Abstract
Susceptibility to avian influenza viruses (AIVs) can vary greatly among bird species. Chickens and turkeys are major avian species that, like ducks, have been extensively studied for avian influenza. To a lesser extent, minor avian species such as quail, partridges, and pheasants have also been investigated for avian influenza. Usually, such game fowl species are highly susceptible to highly pathogenic AIVs and may consistently spread both highly pathogenic AIVs and low-pathogenic AIVs. These findings, together with the fact that game birds are considered bridge species in the poultry–wildlife interface, highlight their interest from the transmission and biosecurity points of view. Here, the general pathobiological features of low-pathogenic AIV and highly pathogenic AIV infections in this group of avian species have been covered.
Introduction
Avian influenza viruses (AIVs) can infect a wide range of domestic poultry (both major and minor avian species) and wild birds under natural and experimental conditions. Based on the ability to produce disease and death in the major domestic poultry species (i.e. the chicken [Gallus domesticus]), the AIV group can be classified into two different pathotypes: low pathogenic (LP) and highly pathogenic (HP) (Swayne & Pantin-Jackwood, Citation2008). LPAIVs can be asymptomatic, but they typically cause mild to severe respiratory disease, often accompanied by a decrease of water or feed consumption, and drops in egg production. In contrast, HPAIVs cause severe systemic disease with very high mortality in chickens, but this does not necessarily provide a predictor for disease in other species (Swayne & Pantin-Jackwood, Citation2008).
Since the first isolation of an AIV from wild birds, an H5N3 HPAIV from common terns (Sterna hirundo) in South Africa in 1961 (Becker, Citation1966), influenza A viruses of all 16 haemagglutinin and nine neuraminidase subtypes have been isolated from more than 100 wild bird species belonging to more than 25 families (Alexander, Citation2007), which confirms that AIVs have a global distribution within free-living aquatic avian populations. Wild aquatic birds, particularly those belonging to the orders Anseriformes (waterfowl, i.e. ducks, geese, and swans) and Charadriiformes (gulls and shorebirds), have long been recognized as the natural host and reservoir for all LPAIVs (Slemons et al., Citation1974; Kawaoka et al., Citation1988; Alexander, Citation2000). Such viruses are highly adapted to their reservoirs, normally producing asymptomatic infections and replicating in the epithelial cells of the gastrointestinal tract, with high amounts of infectious virus shed in faeces during prolonged periods (Webster et al., Citation1978; Hinshaw et al., Citation1980). Therefore, the faecal–oral route is the primary route of transmission of LPAIVs in aquatic birds (Hinshaw et al., Citation1979; Alexander, Citation2007). LPAIVs can be found in numerous bird species other than their reservoirs (Munster et al., Citation2007), but it is unclear in which of these species LPAIVs are endemic and in which the virus is a transient agent. In contrast to LPAIVs, HPAIVs are not normally present in the wild-bird host reservoir (Rohm et al., Citation1995), but typically arise from H5 and H7 LPAIVs after having circulated in gallinaceous poultry for a variable period of time (Horimoto et al., Citation1995; Capua & Marangon, Citation2000; Abdelwhab et al., Citation2013). Therefore, the exposure of waterfowl viruses to poultry through either direct contact with wild birds (Alexander, Citation2007), live poultry markets (Suarez et al., Citation1999; Kung et al., Citation2003) or birds’ drinking water (Swayne, Citation2008) is critical in the emergence of HPAIVs. Since 2002, continuous outbreaks of the panzootic H5N1 HPAIV have been reported in domestic and wild Anseriformes in Asia and Eastern Europe (Hulse-Post et al., Citation2005; Kwon et al., Citation2005; Lee et al., Citation2005; Mase et al., Citation2005; Sturm-Ramirez et al., Citation2005; Stallknecht & Brown, Citation2008). Such evolved viruses are HP in these species under experimental conditions (Pantin-Jackwood et al., Citation2007; Londt et al., Citation2008), which is unusual for HPAIVs (Alexander et al., Citation1978, Citation1986). In contrast to LPAIVs, the H5N1 HP avian influenza (AI) viral shedding pattern in waterfowl is predominantly oral, involves low viral titres, and is of short duration (Sturm-Ramirez et al., Citation2005; Brown et al., Citation2006; Pantin-Jackwood & Swayne, Citation2007; Stallknecht & Brown, Citation2008).
Chickens and turkeys (Meleagris gallopavo) are major avian species that, like ducks, have been extensively studied for AI. Some studies suggest that turkeys are more susceptible to LPAIVs than are chickens (Tumpey et al., Citation2004; Pillai et al., Citation2010; Spackman et al., Citation2010), according also to field outbreaks (Capua et al., Citation2000; Mutinelli et al., Citation2003). Such high susceptibility demonstrates the relevance of turkeys as an intermediate host and source of infection for other land-based poultry or even mammals (Pillai et al., Citation2010; Spackman et al., Citation2010). Besides, turkeys seem to be highly susceptible to HPAIVs, displaying very similar viral dynamics and pathological findings to chickens (Alexander et al., Citation1986; Perkins & Swayne, Citation2001; Perkins & Swayne, Citation2003). Experimental studies in chickens and turkeys have shown that LPAIVs are shed predominantly from the oropharynx and in slightly lower quantity from the cloaca (Tumpey et al., Citation2004; Swayne & Beck, Citation2005; Spackman et al., Citation2010). Similar dynamics occur for HPAIVs, although in higher amounts than LPAIVs (Swayne et al., Citation2000; Swayne & Beck, Citation2005).
To date, several experimental studies and natural infections have assessed the susceptibility to both LPAIVs and HPAIVs of major avian species (chickens, turkeys, and domestic ducks) and wild aquatic birds, for which information has already been summarized and reviewed (Pantin-Jackwood & Swayne, Citation2007, Citation2009; Spickler et al., Citation2008; Swayne & Pantin-Jackwood, Citation2008; Pillai et al., Citation2010). While minor avian species have also been investigated, to our knowledge this is the first review to cover the general pathobiological features of LPAIV and HPAIV infections in this group of avian species.
Importance of minor avian species
Nowadays, major avian species such as chickens, turkeys, and domestic ducks constitute the primary source of avian meat and eggs in both developed and developing countries (Swayne, Citation2008). In addition, several other species of poultry are raised for meat, eggs, feathers, and hides throughout the world (Swayne, Citation2008). These minor species are small providers to agricultural production in the western world, but they are raised for restocking and hunting purposes in such developed countries (Slota et al., Citation2011), and in some developing countries they are relevant contributors to livelihoods (Swayne, Citation2008). These minor species include Japanese quail (J. quail; Coturnix c. japonica), European quail (E. quail; Coturnix c. coturnix), Bobwhite quail (B. quail; Colinus virginianus), ring-necked pheasant (R. pheasant; Phasianus colchicus), Chukar partridge (C. partridge; Alectoris chukar), red-legged partridge (R. partridge; Alectoris rufa), and pearl guinea fowl (P. guinea fowl; Numida meleagris), among others (Swayne, Citation2008). Collectively, not only can these species be found in the wild, but they are also normally raised in outdoor operations, which have higher AIV infection rates than intensive industrial poultry (Shortridge, Citation1999), or are found in live poultry market systems (Senne et al., Citation2005). Therefore, minor avian species could be considered bridge species in the poultry–wildlife interface, which highlights their interest from the transmission and biosecurity points of view.
Certain minor species, such as the J. quail, have been extensively studied due to the interesting features they present towards AI. Several experimental infections in J. quail have reported either higher, similar or lower susceptibilities than chickens to H5 HPAIV (Alexander et al., Citation1986; Tashiro et al., Citation1987; Perkins & Swayne, Citation2001; Webster et al., Citation2002; Jeong et al., Citation2009; Saito et al., Citation2009). Besides, especially swine influenza viruses but also human influenza viruses readily replicate in J. quail (Makarova et al., Citation2003); however, further molecular adaptation may be necessary to allow efficient replication and transmission in this species (Perez et al., Citation2003a, Citationb). Recent studies suggested that multiple in vivo passages in J. quail facilitate the adaptation of duck AIVs to chicken (Sorrell & Perez, Citation2007; Hossain et al., Citation2008; Cilloni et al., Citation2010; Giannecchini et al., Citation2010). These cumulative observations along the years have been recently explained by molecular adaptation of quail AI strains, especially in haemagglutinin and neuraminidase genes, whose amino acid pattern might be intermediate between those of duck and chicken viruses (Castrucci et al., Citation1993; Perez et al., Citation2003a; Sorrell et al., Citation2010). In addition, quail carry sialic acid receptors functional for binding of avian and human influenza viruses (Wan & Perez, Citation2006; Costa et al., Citation2012). Less studied game fowl species such as E. quail, R. partridge, and golden pheasant (Chrysolophus pictus) may also express both types of receptors in the respiratory and intestinal tracts (Costa et al., Citation2012). Therefore, even if there are variations among species (Costa et al., Citation2012), gallinaceous birds could provide an optimal environment for the adaptation of wild-bird AIVs, generating novel variants that can cross the species barrier to domestic poultry and human beings. Collectively, these findings highlight the relevance of avian species other than chickens, turkeys, and ducks in the dynamics of AI.
Pathobiology of LPAIVs in minor gallinaceous species
Clinical signs
Typically, LPAIVs in gallinaceous poultry produce high morbidity (> 50%) and low mortality (< 5%) rates, although the latter can be greater if accompanied by secondary pathogens or if the disease occurs in young birds. Some studies suggest that certain gallinaceous species such as R. pheasants and J. quail are more susceptible to waterfowl LPAIVs than are chickens (Senne et al., Citation1992; Perez et al., Citation2003a; Humberd et al., Citation2006; Swayne & Halvorson, Citation2008; Spackman et al., Citation2010). Natural LPAIV infections in game fowl have reported either asymptomatic disease, like H7N6 in J. quail (Sugiura et al., Citation2009), or symptomatic disease with conjunctivitis, respiratory signs, and even neurologic signs including opistothonus, torticollis, and paralysis of the wings, with mortality rates reaching 30% as for H7N1 in P. guinea fowl (Mutinelli et al., Citation2003). Nevertheless, it is worth pointing out that such severe neurological signs are distinctive of HPAIVs, and thus LP traits of this particular AIV affecting P. guinea fowl should be confirmed. Indeed, under experimental conditions, LPAIVs do not typically cause morbidity or mortality in gallinaceous birds (Alexander et al., Citation1986; Makarova et al., Citation2003; Perez et al., Citation2003a,Citationb; Humberd et al., Citation2006, Citation2007; Bertran et al., Citation2011, Citation2013; Thontiravong et al., Citation2012a;). Nonetheless, some subtypes such as H9N2 or H10 may result in non-specific clinical signs including decreased activity, ruffled feathers, coughing and sneezing, diarrhoea, and fall in egg production as reported in J. quail (Makarova et al., Citation2003; Lavoie et al., Citation2007; Nili et al., Citation2007; Ebrahimi et al., Citation2011; Bonfante et al., Citation2013) and C. partridge (Nili et al., Citation2013).
Gross and microscopic lesions
Probably due to the infrequent clinical signs observed, few studies have assessed the histopathological changes caused by LPAIV in minor gallinaceous poultry (Nili et al., Citation2007, Citation2013; Bertran et al., Citation2011, Citation2013; Thontiravong et al.,Citation 2012b). Among such studies, R. partridges and E. quail lacked gross and microscopic lesions when inoculated intranasally with H7N9 and H7N2 LPAIVs, respectively (Bertran et al., Citation2011, Citation2013). On the contrary, J. quail inoculated with a duck-origin H3N2 LPAIV had mild nasal discharge at necropsy and congestion of the lung and duodenum, the former throughout the 7-day-long experiment and the latter only on 7 days post inoculation (dpi) (Thontiravong et al., Citation2012b). Recently, Nili et al. (Citation2013) observed hyperaemia in the trachea and small intestine at 6 dpi in two out of 25 C. partridges inoculated with H9N2 LPAIV.
Microscopic lesions were mostly detected on 7 dpi and were restricted to the trachea, lung, and duodenum; they consisted of heterophilic-to-lymphocytic tracheitis, deciliation and sloughing of epithelial cells in the trachea, mild to moderate bronchitis, peribronchiolar cuffing with heterophilic and lymphocytic infiltration surrounding the bronchioles, mild to moderate diffuse pulmonary congestion and haemorrhage, and mild to moderate duodenitis with heterophilic and monocytic infiltration (Thontiravong et al., Citation2012b). Minimal staining revealed by immunohistochemistry (IHC) was observed in macrophages within the duodenal lamina propria at 3 dpi (Thontiravong et al., Citation2012b). In another experimental infection with H9N2 LPAIV in J. quail, similar lesions were observed in the trachea and lung (Ebrahimi et al., Citation2011).
Viral shedding and transmission
Even if scarce pathology has been reported in LPAIV-infected game fowl, efficient viral replication may be a concern in terms of maintenance and spread of AI in both domestic and wild populations. As with chickens (Spickler et al., Citation2008), wild game fowl species have shown LPAI viral shedding primarily associated with the respiratory rather than the alimentary tract. However, differences in viral shedding effectiveness have been observed among species and for different viruses. Therefore, J. quail (Makarova et al., Citation2003; Bonfante et al., Citation2013), E. quail (Bertran et al., Citation2013), and R. pheasants (Humberd et al., Citation2006, Citation2007) are recognized as efficient shedders of LPAIV, especially by the oral route but occasionally also by the cloacal route. In particular, inoculation of LPAIV representing subtypes H1 to H15 proved that J. quail may support the replication (predominantly in the respiratory tract) of almost all of them (Makarova et al., Citation2003). On the contrary, partridges of the Chukar (Humberd et al., Citation2006) and red-legged (Bertran et al., Citation2011) subspecies may not be reservoirs for LPAIVs, since these birds are short-term shedders of such AIVs and replication might be limited to the respiratory tract. Linked to the ability to shed viable virus in sufficient amounts and duration, viral transmission among birds has been confirmed in all of the mentioned species except from the R. partridge, not only with avian-origin but also with human-origin and swine-origin influenza viruses (Alexander et al., Citation1986; Makarova et al., Citation2003; Perez et al., Citation2003a, Citationb; Humberd et al., Citation2006, Citation2007; Thontiravong et al., Citation2012a; Bonfante et al., Citation2013; Bertran et al., Citation2013). Based on viral shedding patterns, viral transmission among game fowl may occur through the oral–oral route, as some authors have already pointed out (Kobayashi et al., Citation1996; Makarova et al., Citation2003; Brown et al., Citation2006; Capua & Alexander, Citation2006; Jeong et al., Citation2009; Saito et al., Citation2009). Such viral shedding predominance is already known to differ from that observed in LPAIV waterfowl reservoirs (Webster et al., Citation1978).
The infectious period, incubation time, and clinical signs of LPAIV in minor gallinaceous species are summarized in .
Table 1. Infectious period, incubation time, and clinical signs following natural or experimental LPAIV infections in minor gallinaceous species.
Pathobiology of HPAIVs in minor gallinaceous species
Clinical signs
Similarly to chickens, HPAIV infections in game fowl species cause high morbidity and mortality rates, being able to affect 100% of the flock as observed during the H7N1 HPAIV epizootic in Italy (1999 to 2000) in J. quail, P. guinea fowl, and R. pheasants (Mutinelli et al., Citation2003). Under experimental conditions, Perkins and Swayne (Citation2001) demonstrated that the A/Chicken/Hong Kong/220/97 (H5N1) HPAIV strain caused high mortality in seven gallinaceous species including J. quail, B. quail, P. guinea fowl, R. pheasant, and C. partridge.
The clinical presentation mainly depends on the HPAIV strain and species of bird affected, and reflects the extent of HPAIV replication and damage to major organ systems. First, clinical signs are normally non-specific and include anorexia, lethargy, and ruffled feathers (Perkins & Swayne, Citation2001; Mutinelli et al., Citation2003; Jeong et al., Citation2009; Bertran et al., Citation2011, Citation2013). Infection typically progresses towards neurologic signs described as: motor function alterations, such as paresis to paralysis of legs and wings, inability to stand, loss of balance and recumbency with pedalling movements, incoordination, and flapping movements of the wings; and vestibular alterations as indicated by torticollis, nystagmus, head tilt, tremors of head and neck, convulsions, opisthotonus, and circling movements (Perkins & Swayne, Citation2001; Mutinelli et al., Citation2003; Isoda et al., Citation2006; Bertran et al., Citation2011, Citation2013). Finally, death may be the result of neurologic dysfunction alongside multiorgan failure (Perkins & Swayne, Citation2001; Bertran et al., Citation2011, Citation2013). However, differences in the type of clinical signs and the onset of both clinical signs and mean death time (MDT) are observed between species. Thus Perkins and Swayne (Citation2001) described that the onset of depression in P. guinea fowl and J. quail rapidly progressed to death within 6 to 8 h, while in B. quail, R. pheasants, and C. partridges the advancement of depression was more prolonged and often accompanied by progressive neurologic signs. Presence of neurologic signs varied with the species of bird, ranging from 8% in J. quail to 28% in C. partridges infected with the Eurasian H5N1 HPAIV (Perkins & Swayne, Citation2001). Besides, gastrointestinal signs were also present, such as increased faecal fluid and urates in all seven species tested, and mucous diarrhoea with strings of urates in B. quail, C. partridges, and R. pheasants (Perkins & Swayne, Citation2001). Previous studies with J. quail reported minimal clinical signs or even sudden deaths without apparent signs (Perkins & Swayne, Citation2001; Jeong et al., Citation2009; Saito et al., Citation2009); however, clinically neurologic dysfunction can be an evident sign in HPAIV-infected E. quail (Bertran et al., Citation2013).
The viral strain can also be a determinant of virulence; Bertran et al. (Citation2013) performed a comparative study of the pathogenesis and viral distribution in tissues of two different HPAIV subtypes (H7 and H5). With the earliest onset, most rapid progression of disease, and shortest MDT in H5N1 HPAIV-infected E. quail, it seems apparent that this virus was more virulent for this species than an H7N1 HPAIV strain.
Gross lesions
Unlike LPAIVs, which typically do not cause gross lesions in game fowl species (Bertran et al., Citation2011, Citation2013), lesions are normally present in HPAIV-infected gallinaceous birds and tend to reflect the clinical presentation of the disease. Consequently, birds that suddenly die in the absence of clinical signs may not show gross lesions, while most pronounced lesions are found in birds dying after a more progressive disease (Perkins & Swayne, Citation2001; Bertran et al., Citation2013).
Certain gross findings indicative of AI may be not as extensive and obvious for game fowl as for chickens (e.g. presence of cyanosis and petechial to ecchymotic haemorrhages in the unfeathered skin) (Swayne & Halvorson, Citation2008), but affected tissues are known to be target organs for influenza A viruses. Pancreatic necrotic lesions, characterized by multiple foci of parenchymal discoloration, seem to be a foremost lesion in HPAIV-infected gallinaceous poultry; such lesions have been consistently observed in B. quail and C. partridges experimentally infected with H5N1 (Perkins & Swayne, Citation2001), in E. quail experimentally infected with both H5N1 and H7N1 (Bertran et al., Citation2013), and in J. quail during the Italian H7N1 HPAIV epizootic (1999 to 2000) (Mutinelli et al., Citation2003). Another striking lesion in H5N1 HPAIV-infected game birds is splenomegaly with parenchymal mottling (Perkins & Swayne, Citation2001; Bertran et al., Citation2013). Renomegaly with parenchymal pallor and accentuated lobular surface architecture is commonly observed, often accompanied by urate accumulations in the ureters () (Perkins & Swayne, Citation2001; Bertran et al., Citation2011). Differences among species can be found regarding the presence of pulmonary consolidation with oedema and congestion to haemorrhage; in J. quail and P. guinea fowl it is a common finding, whereas in B. quail, R. pheasants, and C. partridges it may be less frequent (Perkins & Swayne, Citation2001). Mucosal haemorrhage in the alimentary tract, especially in lymphoid areas such as the oesophageal–proventricular junction, Peyer's patches, and caecal tonsil, can be found consistently in J. quail and P. guinea fowl (Perkins & Swayne, Citation2001). Similarly, multifocal petechia on the proventriculus–gizzard junction mucosa has been described in E. quail (Bertran et al., Citation2013). Pheasants, B. quail, and C. partridges can present marked distension of the enteric tract with yellow fluid (Perkins & Swayne, Citation2001). Haemorrhages in the fasciae sheaths of skeletal muscles are common in P. guinea fowl (Perkins & Swayne, Citation2001) and R. partridges () (Bertran et al., Citation2011), whereas petechial to ecchymotic haemorrhages on the epicardium and/or serosal surfaces of the liver and intestines can be variably present (Perkins & Swayne, Citation2001). Serous exudates in body cavities, such as the pericardial sac and coelom, can be observed in certain species, like R. pheasants (Perkins & Swayne, Citation2001).
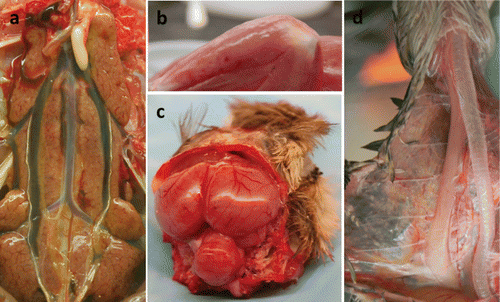
Some lesions seem to be more exclusive within a certain species. Thus, haemorrhage in the cloacal bursa and conjunctival hyperaemia and oedema has so far only been observed in P. guinea fowl (Perkins & Swayne, Citation2001), as well as mucosal hyperaemia to haemorrhage at the submucosal lymphoid tissue of the palate (Perkins & Swayne, Citation2001). Only R. pheasants and C. partridges present intense bile staining of the mucosa of the proventriculus and koilin lining of the ventriculus (Perkins & Swayne, Citation2001). R. partridges frequently display brain congestion and thymus atrophy () (Bertran et al., Citation2011).
Microscopic lesions
Contrasting with the absence of histopathological findings in the majority of LPAIV infections (Bertran et al., Citation2011, Citation2013), microscopic lesions in HPAIV-infected game fowl are generally more consistent than are gross lesions. Extent and severity of lesions, as well as presence of antigen, may vary among avian species, viral strain, and stage of infection (Swayne & Pantin-Jackwood, Citation2008). Typically, histological findings consist of necrosis and/or inflammatory changes in multiple organs, most consistently and severely found in the pancreas, adrenal gland, heart, and brain (Perkins & Swayne, Citation2001; Isoda et al., Citation2006; Bertran et al., Citation2011, Citation2013). Therefore, pancreatic necrosis with antigen revealed by IHC in the pancreatic acinar cells may be common in J. quail (Perkins & Swayne, Citation2001; Mutinelli et al., Citation2003), B. quail (Perkins & Swayne, Citation2001), E. quail (Bertran et al., Citation2013), P. guinea fowl (Perkins & Swayne, Citation2001), C. partridge (Perkins & Swayne, Citation2001), and R. partridge (Bertran et al., Citation2011), often associated with lymphoplasmacytic infiltrates depending on the stage of infection (Bertran et al., Citation2011, Citation2013). In the same species, except from E. quail but including R. pheasants, adrenal necrosis with positive AIV antigen in corticotrophic and cortical cells is often present (Perkins & Swayne, Citation2001; Bertran et al., Citation2011). Alongside adrenal gland lesions, renal lesions and viral antigen can be present in some species, including R. partridges () (Bertran et al., Citation2011), E. quail (Bertran et al., Citation2013), and J. quail (Antarasena et al., Citation2006). Myocardial necrosis or myocarditis with IHC-positive cardiomyocytes is commonly observed in all the species mentioned above (Perkins & Swayne, Citation2001; Antarasena et al., Citation2006; Isoda et al., Citation2006; Bertran et al., Citation2011, Citation2013), sometimes together with myocyte necrosis and positive myocytes in the skeletal muscle (Perkins & Swayne, Citation2001; Bertran et al., Citation2011, Citation2013). The HPAIVs, especially the Eurasian H5N1 HPAIVs, are generally defined as neurotropic (Perkins & Swayne, Citation2001; Jones & Swayne, Citation2004; Swayne & Pantin-Jackwood, Citation2008) and are thought to reach the central nervous system via the haematogenous route (Chaves et al., Citation2011). Frequent lesions in the brain are lymphocytic meningoencephalitis with neuronal necrosis, neuronophagia, and focal gliosis () (Perkins & Swayne, Citation2001; Isoda et al., Citation2006; Bertran et al., Citation2011, Citation2013). Necrosis of the ependyma and neuropil of the spinal cord, especially in the areas surrounding the medullary canal, has been reported in E. quail (Bertran et al., Citation2013) and R. partridges (Bertran et al., Citation2011). Common cells containing AIV antigen are neurons (Perkins & Swayne, Citation2001; Mutinelli et al., Citation2003; Isoda et al., Citation2006; Bertran et al., Citation2011, Citation2013), glial cells (Perkins & Swayne, Citation2001; Bertran et al., Citation2011, Citation2013), and ependymal and choroid epithelial cells (Perkins & Swayne, Citation2001; Bertran et al., Citation2011, Citation2013) of the brain (). Spinal cord neurons and ependymal cells surrounding the medullary canal, as well as cells of the leptomeninges, may be positively stained by IHC when the spinal cord is affected (Bertran et al., Citation2011, Citation2013). Interestingly, in Bertran et al.'s (Citation2013) comparative study between H7 and H5 subtypes, H5N1 HPAIV showed a broader tissue distribution compared with H7N1 HPAIV (), suggesting that virus replication in a particular target organ other than respiratory or intestinal organs may contribute to the virulence of the HPAIV in E. quail, as previously stated for the J. quail (Saito et al., Citation2009).
![Figure 2. Microscopic lesions and positive viral antigen cells in H7N1 HPAIV-infected R. partridges (Bertran et al., Citation2011). 2a: Kidney and adrenal gland, 5 dpi Necrosis of the tubular epithelial cells of the renal cortex, multifocal to coalescent areas of necrosis of corticotrophic and corticotropic adrenal cells (haematoxylin and eosin [HE]). 2b: Kidney and adrenal gland, 5 dpi Tubular epithelial cells of the kidney, corticotrophic and corticotropic adrenal cells (IHC). 2c: Brain, 5 dpi Focal areas of malacia (HE). 2d: Brain, 5 dpi Neurons, ependymal cells, and glial cells (IHC). 2e: Nasal turbinates, 6 dpi Necrosis of single cells of the olfactory epithelium (HE). 2f: Nasal turbinates, 6 dpi Olfactory epithelial cells (IHC). 2g: Gizzard, 3 dpi Focal areas of degeneration and necrosis of the gastric glands (HE). 2h: Gizzard, 3 dpi Epithelial cells of the gastric glands (IHC).](/cms/asset/754a8b50-7a9e-4a14-a703-7e7ce9786517/cavp_a_876529_f0002_c.jpg)
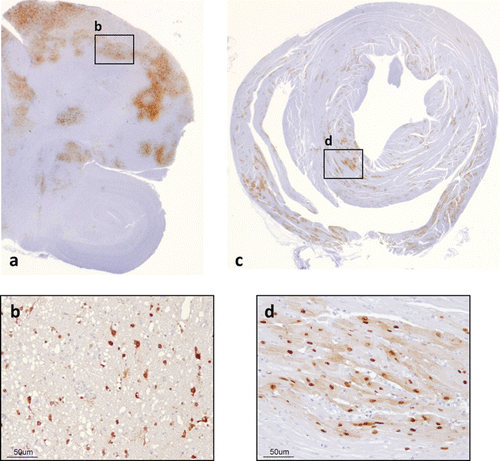
To some extent, and depending on the stage of infection, involvement of other systems is observed. Regarding the respiratory tract, in most game fowl species, such as J. quail, B. quail, P. guinea fowl, R. pheasants or C. partridges, nasal epithelial necrosis, rhinitis, and sinusitis can be observed, together with AIV antigen in nasal and sinus epithelium (Perkins & Swayne, Citation2001). In these species, interstitial pneumonia with positive pulmonary endothelial cells has also been described (Perkins & Swayne, Citation2001). In some cases, scarce lesions with strong antigenic staining in the olfactory and respiratory epithelial cells of nasal turbinates (), in pseudostratified epithelial cells of the trachea, and in air capillaries cells of the lung are indicative of active viral replication in these locations (Bertran et al., Citation2011). In naturally H5N1 HPAIV-infected J. quail, the epithelium and muscular layer of the trachea and endothelial cells of the septum of the lung have been reported to be positively stained by IHC (Antarasena et al., Citation2006). Among the different gallinaceous species tested, Perkins and Swayne (Citation2001) defined lesions in the digestive tract to be mainly confined to lymphoid-associated areas, especially the caecal tonsils but also the oesophageal–proventricular junction and the Peyer's patches of the small intestine. Heterophilic enteritis with enteric lymphoid depletion alongside enteric epithelial necrosis was a common finding in J. quail, B. quail, P. guinea fowl, R. pheasant, and C. partridge (Perkins & Swayne, Citation2001). In the absence of histopathological lesions, viral antigen has been described in the lamina propria of the caecal tonsils of E. quail (Bertran et al., Citation2013) and in the epithelium of the gastric glands of the gizzard in R. partridges () (Bertran et al., Citation2011). Non-lymphoid-associated tissues can also be affected, as necrosis and lymphoplasmacytic infiltrate in the gizzard has been described in E. quail, with positive AIV antigenic staining in the epithelial cells of the ventricular glands of the gizzard (Bertran et al., Citation2013). Epithelial degeneration and lymphoplasmacytic infiltrate in the rectum can also be observed in this species, being the muscularis externa of the rectum found positive for AIV antigen (Bertran et al., Citation2013). Viral antigen has also been observed in the mucosal epithelium of the rectum of J. quail (Antarasena et al., Citation2006). The haematopoietic tissues (i.e. spleen, cloacal bursa, thymus, and bone marrow) typically present cellular depletion and necrosis, although the spleen is one of the most rapidly affected organs (Perkins & Swayne, Citation2001). Lesions in the ovaries and testicles are limited, although certain cells of such tissues can be targeted by HPAIV (Perkins & Swayne, Citation2001). Among the other game bird species, only P. guinea fowl seems to display subcutaneous oedema as severe as in chickens (Perkins & Swayne, Citation2001). Viral antigen associated with the epithelium of feather follicles has frequently been described in various species; lesions such as necrosis of the epidermal collar epithelium with heterophilic infiltrate have been observed in the R. partridge (Bertran et al., Citation2011). Regardless of the species, viral antigen has been extensively found in macrophages and vascular endothelial cells of a number of tissues including the lung, pancreas, liver, kidney, heart, skeletal muscle, brain, spleen, and cloacal bursa, indicating the systemic nature of HPAIV infections in gallinaceous poultry (Perkins & Swayne, Citation2001; Antarasena et al., Citation2006; Bertran et al., Citation2011, Citation2013).
Viral shedding
As in chickens (Spickler et al., Citation2008), HPAI viral shedding in game fowl birds is associated with both the respiratory and digestive tracts, although slightly higher and longer viral oral shedding is observed (Jeong et al., Citation2009; Saito et al., Citation2009; Bertran et al., Citation2011, Citation2013; Sun et al., Citation2011). Collectively, gallinaceous species including J. quail (Jeong et al., Citation2009; Saito et al., Citation2009), E. quail (Bertran et al., Citation2013), and R. partridges (Bertran et al., Citation2011) can be considered efficient shedders of HPAIV. Viral transmission among birds has been proved in the above mentioned species and, like LPAIV, it probably occurs through the oral–oral route (Jeong et al., Citation2009; Saito et al., Citation2009; Bertran et al., Citation2011, Citation2013; Sun et al., Citation2011). Some HPAIV-infected gallinaceous birds, like R. partridges (Bertran et al., Citation2011) and E. quail (Bertran et al., Citation2013), may shed virus at high concentrations before the appearance of overt clinical signs and/or death. Therefore, spreading disease into the wild by releasing apparently healthy farm-reared game birds for hunting purposes could represent a substantial threat, which highlights the need for effective surveillance programmes in these species.
Interestingly, not only oral and cloacal secretions but also feathers could be a source for HPAIV transmission in game birds, as shown in R. partridges (Bertran et al., Citation2011) and E. quail (Bertran et al., Citation2013). Both studies confirmed high loads of viral RNA in feather pulp samples over a week following experimental infection with HPAIV (Bertran et al., Citation2011, Citation2013). To date, besides game fowl, the relevance of feathers as a location for viral replication and potential origin of dissemination in H5N1 HPAIV infection has been reported in H5N1 HPAIV infection in Anseriformes (Yamamoto et al., Citation2007, Citation2008a, Citationb, Citation2009; Londt et al., Citation2008) and in H7N1 HPAIV infection in chickens (Busquets et al., Citation2010). These results suggest that feathers could represent a source of environmental contamination, as well as a source of infection for other birds (including game birds or birds of prey) or mammals that pick dead individuals, and thus eat infected feathers or inhale their dusts. Furthermore, feathers may represent a risk of zoonotic infection when being plucked from dead infected birds without appropriate protective equipment, as already suggested for waterfowl (Yamamoto et al., Citation2009).
The infectious period, incubation time, and clinical signs of HPAIVs in minor gallinaceous species are summarized in . Gross pathology, histological lesions, and distribution of AIV antigen in minor gallinaceous species following HPAIV infections are summarized in .
Table 2. Infectious period, incubation time, and clinical signs following natural or experimental HPAIV infections in minor gallinaceous species.
Table 3. Gross pathology, histological lesions, and distribution of AIV antigen in minor gallinaceous species following natural or experimental HPAIV infections.
Conclusions
Minor avian species may represent a small proportion of birds reared for human purposes. Nevertheless, their value as wild birds in some geographical regions, alongside the high susceptibility to HPAIV and the active viral replication of both HPAIV and LPAIV, emphasizes their relevance within AI epidemiology. Indeed, they may be considered sentinel species for HPAIVs and reservoirs for AIVs in general. Collectively, these findings underline the complexity of managing AI outbreaks when different susceptible species are involved.
Acknowledgements
This work was partially funded by the coordinated project FAU2006-00019-C03-00 and the RTA2011-00111-C03 of the Instituto Nacional de Investigación y Tecnología Agraria y Alimentaria (INIA). Dr Bertran had an FPU Pre-Doctoral grant from the Ministerio de Educación, Cultura y Deporte of Spain.
References
- Abdelwhab, E.M., Veits, J. & Mettenleiter, T.C. (2013). Genetic changes that accompanied shifts of low pathogenic avian influenza viruses toward higher pathogenicity in poultry. Virulence, 4, 441–452. 10.4161/viru.25710
- Alexander, D.J. (2000). A review of avian influenza in different bird species. Veterinary Microbiology, 74, 3–13. 10.1016/S0378-1135(00)00160-7
- Alexander, D.J. (2007). An overview of the epidemiology of avian influenza. Vaccine, 25, 5637–5644. 10.1016/j.vaccine.2006.10.051
- Alexander, D.J., Allan, W.H., Parsons, D.G. & Parsons, G. (1978). The pathogenicity of four avian influenza viruses for fowls, turkeys and ducks. Research in Veterinary Science, 24, 242–247.
- Alexander, D.J., Parsons, G. & Manvell, R.J. (1986). Experimental assessment of the pathogenicity of eight avian influenza A viruses of H5 subtype for chickens, turkeys, ducks and quail. Avian Pathology, 15, 647–662. 10.1080/03079458608436328
- Antarasena, C., Sirimujalin, R., Prommuang, P., Blacksell, S.D., Promkuntod, N. & Prommuang, P. (2006). Tissue tropism of a Thailand strain of high-pathogenicity avian influenza virus (H5N1) in tissues of naturally infected native chickens (Gallus gallus), Japanese quail (Coturnix coturnix japonica) and ducks (Anas spp.). Avian Pathology, 35, 250–253. 10.1080/03079450600714510
- Becker, W.B. (1966). The isolation and classification of Tern virus: influenza virus A/Tern/South Africa/1961. The Journal of Hygiene, 64, 309–320. 10.1017/S0022172400040596
- Bertran, K., Dolz, R., Busquets, N., Gamino, V., Vergara-Alert, J., Chaves, A.J., Ramis, A., Abad, X.F., Hofle, U. & Majo, N. (2013). Pathobiology and transmission of highly and low pathogenic avian influenza viruses in European quail (Coturnix c. coturnix). Veterinary Research, 44, 23. 10.1186/1297-9716-44-23
- Bertran, K., Perez-Ramirez, E., Busquets, N., Dolz, R., Ramis, A., Darji, A., Abad, F.X., Valle, R., Chaves, A., Vergara-Alert, J., Barral, M., Hofle, U. & Majo, N. (2011). Pathogenesis and transmissibility of highly (H7N1) and low (H7N9) pathogenic avian influenza virus infection in red-legged partridge (Alectoris rufa). Veterinary Research, 42, 24. 10.1186/1297-9716-42-24
- Bonfante, F., Patrono, L.V., Aiello, R., Beato, M.S., Terregino, C. & Capua, I. (2013). Susceptibility and intra-species transmission of the H9N2 G1 prototype lineage virus in Japanese quail and turkeys. Veterinary Microbiology, 165, 177–183. 10.1016/j.vetmic.2013.03.014
- Brown, J.D., Stallknecht, D.E., Beck, J.R., Suarez, D.L. & Swayne, D.E. (2006). Susceptibility of North American ducks and gulls to H5N1 highly pathogenic avian influenza viruses. Emerging Infectious Diseases, 12, 1663–1670. 10.3201/eid1211.060652
- Busquets, N., Abad, F.X., Alba, A., Dolz, R., Allepuz, A., Rivas, R., Ramis, A., Darji, A. & Majo, N. (2010). Persistence of highly pathogenic avian influenza virus (H7N1) in infected chickens: feather as a suitable sample for diagnosis. The Journal of General Virology, 91, 2307–2313. 10.1099/vir.0.021592-0
- Capua, I. & Alexander, D.J. (2006). The challenge of avian influenza to the veterinary community. Avian Pathology, 35, 189–205. 10.1080/03079450600717174
- Capua, I. & Marangon, S. (2000). The avian influenza epidemic in Italy, 1999–2000: a review. Avian Pathology, 29, 289–294. 10.1080/03079450050118403
- Capua, I., Mutinelli, F., Marangon, S. & Alexander, D.J. (2000). H7N1 avian influenza in Italy (1999 to 2000) in intensively reared chickens and turkeys. Avian Pathology, 29, 537–543. 10.1080/03079450020016779
- Castrucci, M.R., Donatelli, I., Sidoli, L., Barigazzi, G., Kawaoka, Y. & Webster, R.G. (1993). Genetic reassortment between avian and human influenza A viruses in Italian pigs. Virology, 193, 503–506. 10.1006/viro.1993.1155
- Chaves, A.J., Busquets, N., Valle, R., Rivas, R., Vergara-Alert, J., Dolz, R., Ramis, A., Darji, A. & Majo, N. (2011). Neuropathogenesis of a highly pathogenic avian influenza virus (H7N1) in experimentally infected chickens. Veterinary Research, 42, 106. 10.1186/1297-9716-42-106
- Cilloni, F., Toffan, A., Giannecchini, S., Clausi, V., Azzi, A., Capua, I. & Terregino, C. (2010). Increased pathogenicity and shedding in chickens of a wild bird-origin low pathogenicity avian influenza virus of the H7N3 subtype following multiple in vivo passages in quail and turkey. Avian Diseases, 54, 555–557. 10.1637/8919-050809-Reg.1
- Costa, T., Chaves, A.J., Valle, R., Darji, A., van Riel, D., Kuiken, T., Majo, N. & Ramis, A. (2012). Distribution patterns of influenza virus receptors and viral attachment patterns in the respiratory and intestinal tracts of seven avian species. Veterinary Research, 43, 28. 10.1186/1297-9716-43-28
- Ebrahimi, S.M., Ziapour, S., Tebianian, M., Dabaghian, M. & Mohammadi, M. (2011). Study of infection with an Iranian field-isolated H9N2 avian influenza virus in vaccinated and unvaccinated Japanese quail. Avian Diseases, 55, 195–200. 10.1637/9538-092110-Reg.1
- Giannecchini, S., Clausi, V., Di Trani, L., Falcone, E., Terregino, C., Toffan, A., Cilloni, F., Matrosovich, M., Gambaryan, A.S., Bovin, N.V., Delogu, M., Capua, I., Donatelli, I. & Azzi, A. (2010). Molecular adaptation of an H7N3 wild duck influenza virus following experimental multiple passages in quail and turkey. Virology, 408, 167–173. 10.1016/j.virol.2010.09.011
- Hinshaw, V.S., Webster, R.G. & Turner, B. (1979). Water-bone transmission of influenza A viruses? Intervirology, 11, 66–68. 10.1159/000149014
- Hinshaw, V.S., Webster, R.G. & Turner, B. (1980). The perpetuation of orthomyxoviruses and paramyxoviruses in Canadian waterfowl. Canadian Journal of Microbiology, 26, 622–629. 10.1139/m80-108
- Horimoto, T., Rivera, E., Pearson, J., Senne, D., Krauss, S., Kawaoka, Y. & Webster, R.G. (1995). Origin and molecular changes associated with emergence of a highly pathogenic H5N2 influenza virus in Mexico. Virology, 213, 223–230. 10.1006/viro.1995.1562
- Hossain, M.J., Hickman, D. & Perez, D.R. (2008). Evidence of expanded host range and mammalian-associated genetic changes in a duck H9N2 influenza virus following adaptation in quail and chickens. PLoS One, 3, e3170. 10.1371/journal.pone.0003170
- Hulse-Post, D.J., Sturm-Ramirez, K.M., Humberd, J., Seiler, P., Govorkova, E.A., Krauss, S., Scholtissek, C., Puthavathana, P., Buranathai, C., Nguyen, T.D., Long, H.T., Naipospos, T.S., Chen, H., Ellis, T.M., Guan, Y., Peiris, J.S. & Webster, R.G. (2005). Role of domestic ducks in the propagation and biological evolution of highly pathogenic H5N1 influenza viruses in Asia. Proceedings of the National Academy of Sciences of the United States of America, 102, 10682–10687. 10.1073/pnas.0504662102
- Humberd, J., Boyd, K. & Webster, R.G. (2007). Emergence of influenza A virus variants after prolonged shedding from pheasants. Journal of Virology, 81, 4044–4051. 10.1128/JVI.02346-06
- Humberd, J., Guan, Y. & Webster, R.G. (2006). Comparison of the replication of influenza A viruses in Chinese ring-necked pheasants and chukar partridges. Journal of Virology, 80, 2151–2161. 10.1128/JVI.80.5.2151-2161.2006
- Isoda, N., Sakoda, Y., Kishida, N., Bai, G.R., Matsuda, K., Umemura, T. & Kida, H. (2006). Pathogenicity of a highly pathogenic avian influenza virus, A/chicken/Yamaguchi/7/04 (H5N1) in different species of birds and mammals. Archives of Virology, 151, 1267–1279. 10.1007/s00705-005-0723-6
- Jeong, O.M., Kim, M.C., Kim, M.J., Kang, H.M., Kim, H.R., Kim, Y.J., Joh, S.J., Kwon, J.H. & Lee, Y.J. (2009). Experimental infection of chickens, ducks and quails with the highly pathogenic H5N1 avian influenza virus. Journal of Veterinary Science, 10, 53–60. 10.4142/jvs.2009.10.1.53
- Jones, Y.L. & Swayne, D.E. (2004). Comparative pathobiology of low and high pathogenicity H7N3 Chilean avian influenza viruses in chickens. Avian Diseases, 48, 119–128. 10.1637/7080
- Kawaoka, Y., Chambers, T.M., Sladen, W.L. & Webster, R.G. (1988). Is the gene pool of influenza viruses in shorebirds and gulls different from that in wild ducks? Virology, 163, 247–250. 10.1016/0042-6822(88)90260-7
- Kobayashi, Y., Horimoto, T., Kawaoka, Y., Alexander, D.J. & Itakura, C. (1996). Pathological studies of chickens experimentally infected with two highly pathogenic avian influenza viruses. Avian Pathology, 25, 285–304. 10.1080/03079459608419142
- Kung, N.Y., Guan, Y., Perkins, N.R., Bissett, L., Ellis, T., Sims, L., Morris, R.S., Shortridge, K.F. & Peiris, J.S. (2003). The impact of a monthly rest day on avian influenza virus isolation rates in retail live poultry markets in Hong Kong. Avian Diseases, 47, 1037–1041. 10.1637/0005-2086-47.s3.1037
- Kwon, Y.K., Joh, S.J., Kim, M.C., Sung, H.W., Lee, Y.J., Choi, J.G., Lee, E.K. & Kim, J.H. (2005). Highly pathogenic avian influenza (H5N1) in the commercial domestic ducks of South Korea. Avian Pathology, 34, 367–370. 10.1080/03079450500181257
- Lavoie, E.T., Sorrell, E.M., Perez, D.R. & Ottinger, M.A. (2007). Immunosenescence and age-related susceptibility to influenza virus in Japanese quail. Developmental and Comparative Immunology, 31, 407–414. 10.1016/j.dci.2006.07.009
- Lee, C.W., Suarez, D.L., Tumpey, T.M., Sung, H.W., Kwon, Y.K., Lee, Y.J., Choi, J.G., Joh, S.J., Kim, M.C., Lee, E.K., Park, J.M., Lu, X., Katz, J.M., Spackman, E., Swayne, D.E. & Kim, J.H. (2005). Characterization of highly pathogenic H5N1 avian influenza A viruses isolated from South Korea. Journal of Virology, 79, 3692–3702. 10.1128/JVI.79.6.3692-3702.2005
- Londt, B.Z., Nunez, A., Banks, J., Nili, H., Johnson, L.K. & Alexander, D.J. (2008). Pathogenesis of highly pathogenic avian influenza A/turkey/Turkey/1/2005 H5N1 in Pekin ducks (Anas platyrhynchos) infected experimentally. Avian Pathology, 37, 619–627. 10.1080/03079450802499126
- Makarova, N.V., Ozaki, H., Kida, H., Webster, R.G. & Perez, D.R. (2003). Replication and transmission of influenza viruses in Japanese quail. Virology, 310, 8–15. 10.1016/S0042-6822(03)00094-1
- Mase, M., Eto, M., Tanimura, N., Imai, K., Tsukamoto, K., Horimoto, T., Kawaoka, Y. & Yamaguchi, S. (2005). Isolation of a genotypically unique H5N1 influenza virus from duck meat imported into Japan from China. Virology, 339, 101–109. 10.1016/j.virol.2005.05.010
- Munster, V.J., Baas, C., Lexmond, P., Waldenstrom, J., Wallensten, A., Fransson, T., Rimmelzwaan, G.F., Beyer, W.E., Schutten, M., Olsen, B., Osterhaus, A.D. & Fouchier, R.A. (2007). Spatial, temporal, and species variation in prevalence of influenza A viruses in wild migratory birds. PLoS Pathogens, 3, e61. 10.1371/journal.ppat.0030061
- Mutinelli, F., Capua, I., Terregino, C. & Cattoli, G. (2003). Clinical, gross, and microscopic findings in different avian species naturally infected during the H7N1 low- and high-pathogenicity avian influenza epidemics in Italy during 1999 and 2000. Avian Diseases, 47, 844–848. 10.1637/0005-2086-47.s3.844
- Nili, H., Asasi, K., Dadras, H. & Ebrahimi, S.M. (2007). Pathobiology of H9N2 avian influenza virus in Japanese quail (Coturnix coturnix japonica). Avian Diseases, 51, 390–392. 10.1637/7550-033106R1.1
- Nili, H., Mohammadi, A., Habibi, H. & Firouzi, S. (2013). Pathogenesis of H9N2 virus in Chukar partridges. Avian Pathology, 42, 230–234. 10.1080/03079457.2013.779362
- Pantin-Jackwood, M.J., Suarez, D.L., Spackman, E. & Swayne, D.E. (2007). Age at infection affects the pathogenicity of Asian highly pathogenic avian influenza H5N1 viruses in ducks. Virus Research, 130, 151–161. 10.1016/j.virusres.2007.06.006
- Pantin-Jackwood, M.J. & Swayne, D.E. (2007). Pathobiology of Asian highly pathogenic avian influenza H5N1 virus infections in ducks. Avian Diseases, 51, 250–259. 10.1637/7710-090606R.1
- Pantin-Jackwood, M.J. & Swayne, D.E. (2009). Pathogenesis and pathobiology of avian influenza virus infection in birds. Revue Scientifique et Technique de l'OIE, 28, 113–136.
- Perez, D.R., Lim, W., Seiler, J.P., Yi, G., Peiris, M., Shortridge, K.F. & Webster, R.G. (2003a). Role of quail in the interspecies transmission of H9 influenza A viruses: molecular changes on HA that correspond to adaptation from ducks to chickens. Journal of Virology, 77, 3148–3156. 10.1128/JVI.77.5.3148-3156.2003
- Perez, D.R., Webby, R.J., Hoffmann, E. & Webster, R.G. (2003b). Land-based birds as potential disseminators of avian mammalian reassortant influenza A viruses. Avian Diseases, 47, 1114–1117. 10.1637/0005-2086-47.s3.1114
- Perkins, L.E. & Swayne, D.E. (2001). Pathobiology of A/Chicken/Hong Kong/220/97 (H5N1) avian influenza virus in seven gallinaceous species. Veterinary Pathology, 38, 149–164. 10.1354/vp.38-2-149
- Perkins, L.E. & Swayne, D.E. (2003). Comparative susceptibility of selected avian and mammalian species to a Hong Kong-origin H5N1 high-pathogenicity avian influenza virus. Avian Diseases, 47, 956–967. 10.1637/0005-2086-47.s3.956
- Pillai, S.P., Pantin-Jackwood, M., Yassine, H.M., Saif, Y.M. & Lee, C.W. (2010). The high susceptibility of turkeys to influenza viruses of different origins implies their importance as potential intermediate hosts. Avian Diseases, 54, 522–526. 10.1637/8770-033109-Review.1
- Rohm, C., Horimoto, T., Kawaoka, Y., Suss, J. & Webster, R.G. (1995). Do hemagglutinin genes of highly pathogenic avian influenza viruses constitute unique phylogenetic lineages? Virology, 209, 664–670. 10.1006/viro.1995.1301
- Saito, T., Watanabe, C., Takemae, N., Chaisingh, A., Uchida, Y., Buranathai, C., Suzuki, H., Okamatsu, M., Imada, T., Parchariyanon, S., Traiwanatam, N. & Yamaguchi, S. (2009). Pathogenicity of highly pathogenic avian influenza viruses of H5N1 subtype isolated in Thailand for different poultry species. Veterinary Microbiology, 133, 65–74. 10.1016/j.vetmic.2008.06.020
- Senne, D.A., Pearson, J.E. & Panigraphy, B. (1992). Live poultry markets: a missing link in the epidemiology of avian influenza. In B.C. Easterday (Ed.). Proceedings of the Third International Symposium on Avian Influenza (p. 50). Madison, Wisconsin.
- Senne, D.A., Pederson, J.C. & Panigraphy, B. (2005). Live-bird markets in the Northeastern United States: a source of avian influenza in commercial poultry. In R.S. Schrijver, & G. Koch (Eds.). Avian Influenza. Prevention and Control (pp. 19–24). Dordrecht: Springer.
- Shortridge, K.F. (1999). Poultry and the influenza H5N1 outbreak in Hong Kong, 1997: abridged chronology and virus isolation. Vaccine, 17 Suppl 1, S26–29. 10.1016/S0264-410X(99)00102-4
- Slemons, R.D., Johnson, D.C., Osborn, J.S. & Hayes, F. (1974). Type-A influenza viruses isolated from wild free-flying ducks in California. Avian Diseases, 18, 119–124. 10.2307/1589250
- Slota, K.E., Hill, A.E., Keefe, T.J., Bowen, R.A., Miller, R.S. & Pabilonia, K.L. (2011). Human-bird interactions in the United States upland gamebird industry and the potential for zoonotic disease transmission. Vector Borne and Zoonotic Diseases, 11, 1115–1123. 10.1089/vbz.2010.0114
- Sorrell, E.M. & Perez, D.R. (2007). Adaptation of influenza A/Mallard/Potsdam/178–4/83 H2N2 virus in Japanese quail leads to infection and transmission in chickens. Avian Diseases, 51, 264–268. 10.1637/7538-032906R.1
- Sorrell, E.M., Song, H., Pena, L. & Perez, D.R. (2010). A 27-amino-acid deletion in the neuraminidase stalk supports replication of an avian H2N2 influenza A virus in the respiratory tract of chickens. Journal of Virology, 84, 11831–11840. 10.1128/JVI.01460-10
- Spackman, E., Gelb, J.,Jr,Preskenis, L.A., Ladman, B.S., Pope, C.R., Pantin-Jackwood, M.J. & McKinley, E.T. (2010). The pathogenesis of low pathogenicity H7 avian influenza viruses in chickens, ducks and turkeys. Virology Journal, 7, 331. 10.1186/1743-422X-7-331
- Spickler, A.R., Trampel, D.W. & Roth, J.A. (2008). The onset of virus shedding and clinical signs in chickens infected with high-pathogenicity and low-pathogenicity avian influenza viruses. Avian Pathology, 37, 555–577. 10.1080/03079450802499118
- Stallknecht, D.E., &Brown, J.D. (2008). Ecology of avian influenza in wild birds. In D.E. Swayne (Ed.). Avian Influenza 1st edn (pp. 43–58). Ames, IA: Blackwell Publishing.
- Sturm-Ramirez, K.M., Hulse-Post, D.J., Govorkova, E.A., Humberd, J., Seiler, P., Puthavathana, P., Buranathai, C., Nguyen, T.D., Chaisingh, A., Long, H.T., Naipospos, T.S., Chen, H., Ellis, T.M., Guan, Y., Peiris, J.S. & Webster, R.G. (2005). Are ducks contributing to the endemicity of highly pathogenic H5N1 influenza virus in Asia? Journal of Virology, 79, 11269–11279. 10.1128/JVI.79.17.11269-11279.2005
- Suarez, D.L., Garcia, M., Latimer, J., Senne, D. & Perdue, M. (1999). Phylogenetic analysis of H7 avian influenza viruses isolated from the live bird markets of the Northeast United States. Journal of Virology, 73, 3567–3573.
- Sugiura, K., Fushimi, K., Takehisa, T., Miwa, M., Saito, T., Uchida, Y. & Onodera, T. (2009). An outbreak of H7N6 low pathogenic avian influenza in quails in Japan. Veterinaria Italiana, 45, 481–489.
- Sun, H., Jiao, P., Jia, B., Xu, C., Wei, L., Shan, F., Luo, K., Xin, C., Zhang, K. & Liao, M. (2011). Pathogenicity in quails and mice of H5N1 highly pathogenic avian influenza viruses isolated from ducks. Veterinary Microbiology, 152, 258–265. 10.1016/j.vetmic.2011.05.009
- Swayne, D.E. (2008). Epidemiology of avian influenza in agricultural and other man-made systems. In D.E. Swayne (Ed.). Avian Influenza 1st edn (pp. 59–85). Ames, IA: Blackwell Publishing.
- Swayne, D.E. & Beck, J.R. (2005). Experimental study to determine if low-pathogenicity and high-pathogenicity avian influenza viruses can be present in chicken breast and thigh meat following intranasal virus inoculation. Avian Diseases, 49, 81–85. 10.1637/7260-081104R
- Swayne, D.E., Garcia, M., Beck, J.R., Kinney, N. & Suarez, D.L. (2000). Protection against diverse highly pathogenic H5 avian influenza viruses in chickens immunized with a recombinant fowlpox vaccine containing an H5 avian influenza hemagglutinin gene insert. Vaccine, 18, 1088–1095. 10.1016/S0264-410X(99)00369-2
- Swayne, D.E. & Halvorson, D.A. (2008). Influenza. In Y.M. Saif, J.R. Glisson, A.M. Fadly, L.R. McDougald & L.K. Nolan (Eds.). Diseases of Poultry 12th edn (pp. 153–184). Ames, IA: Blackwell Publishing.
- Swayne, D.E. & Pantin-Jackwood, M.J. (2008). Pathobiology of avian influenza virus infections in birds and mammals. In D.E. Swayne (Ed.). Avian Influenza 1st edn (pp. 87–122). Ames, IA: Blackwell Publishing.
- Tashiro, M., Reinacher, M. & Rott, R. (1987). Aggravation of pathogenicity of an avian influenza virus by adaptation to quails. Archives of Virology, 93, 81–95. 10.1007/BF01313895
- Thontiravong, A., Kitikoon, P., Wannaratana, S., Tantilertcharoen, R., Tuanudom, R., Pakpinyo, S., Sasipreeyajan, J., Oraveerakul, K. & Amonsin, A. (2012a). Quail as a potential mixing vessel for the generation of new reassortant influenza A viruses. Veterinary Microbiology, 160, 305–313.
- Thontiravong, A., Wannaratana, S., Tantilertcharoen, R., Prakairungnamthip, D., Tuanudom, R., Sasipreeyajan, J., Pakpinyo, S., Amonsin, A., Kitikoon, P. & Oraveerakul, K. (2012b). Comparative study of pandemic (H1N1) 2009, swine H1N1, and avian H3N2 influenza viral infections in quails. Journal of Veterinary Science, 13, 395–403. 10.4142/jvs.2012.13.4.395
- Tumpey, T.M., Kapczynski, D.R. & Swayne, D.E. (2004). Comparative susceptibility of chickens and turkeys to avian influenza A H7N2 virus infection and protective efficacy of a commercial avian influenza H7N2 virus vaccine. Avian Diseases, 48, 167–176. 10.1637/7103
- Wan, H. & Perez, D.R. (2006). Quail carry sialic acid receptors compatible with binding of avian and human influenza viruses. Virology, 346, 278–286. 10.1016/j.virol.2005.10.035
- Webster, R.G., Guan, Y., Peiris, M., Walker, D., Krauss, S., Zhou, N.N., Govorkova, E.A., Ellis, T.M., Dyrting, K.C., Sit, T., Perez, D.R. & Shortridge, K.F. (2002). Characterization of H5N1 influenza viruses that continue to circulate in geese in Southeastern China. Journal of Virology, 76, 118–126. 10.1128/JVI.76.1.118-126.2002
- Webster, R.G., Yakhno, M., Hinshaw, V.S., Bean, W.J. & Murti, K.G. (1978). Intestinal influenza: replication and characterization of influenza viruses in ducks. Virology, 84, 268–278. 10.1016/0042-6822(78)90247-7
- Yamamoto, Y., Nakamura, K., Kitagawa, K., Ikenaga, N., Yamada, M., Mase, M. & Narita, M. (2007). Pathogenesis in call ducks inoculated intranasally with H5N1 highly pathogenic avian influenza virus and transmission by oral inoculation of infective feathers from an infected call duck. Avian Diseases, 51, 744–749. 10.1637/0005-2086(2007)51[744:PICDII]2.0.CO;2
- Yamamoto, Y., Nakamura, K., Okamatsu, M., Miyazaki, A., Yamada, M. & Mase, M. (2008a). Detecting avian influenza virus (H5N1) in domestic duck feathers. Emerging Infectious Diseases, 14, 1671–1672.
- Yamamoto, Y., Nakamura, K., Okamatsu, M., Yamada, M. & Mase, M. (2008b). Avian influenza virus (H5N1) replication in feathers of domestic waterfowl. Emerging Infectious Diseases, 14, 149–151. 10.3201/eid1401.071036
- Yamamoto, Y., Nakamura, K., Yamada, M. & Ito, T. (2009). Zoonotic risk for influenza A (H5N1) infection in wild swan feathers. The Journal of Veterinary Medical Science, 71, 1549–1551. 10.1292/jvms.001549