Abstract
We conducted a longitudinal study to detect and isolate avian metapneumovirus (aMPV) in two highly productive poultry areas in Mexico. A total of 968 breeder hens and pullets from 2 to 73 weeks of age were analysed. Serology was performed to detect aMPV antibodies and 105 samples of tracheal tissue were collected, pooled by age, and used for attempted virus isolation and aMPV nested reverse transcriptase-polymerase chain reaction (nRT-PCR). The serological analysis indicated that 100% of the sampled chickens showed aMPV antibodies by 12 weeks of age. Five pools of pullet samples collected at 3 to 8 weeks of age were positive by nRT-PCR and the sequences obtained indicated 98 to 99% similarity with the reported sequences for aMPV subtype A. Virus isolation of nRT-PCR-positive samples was successfully attempted using chicken embryo lung and trachea mixed cultures with subsequent adaptation to Vero cells. This is the first report of detection and isolation of aMPV in Mexico.
Introduction
Avian metapneumovirus (aMPV) is the aetiological agent of turkey rhinotracheitis (Cook & Cavanagh, Citation2002) and is one of the agents involved in swollen head syndrome in broilers and breeder hens. aMPV affects chickens of all ages and interacts with bacterial agents such as Escherichia coli, Bordetella and Pasteurella, resulting in morbidity in the infected chickens (Buys et al., Citation1989; Cook, Citation2000a, Citationb). aMPV was first reported in South Africa in 1978 and was later detected in France, the UK, Israel, Asia, Brazil and the USA. aMPV is widely distributed throughout the world, except in Australia (Buys et al., Citation1989; Cook, Citation2000b), and the disease is characterized by an acute infection of the respiratory tract in turkeys and chickens. Chickens may present with inflammation of the intra-orbital and peri-orbital sinuses, nasal discharge, conjunctivitis, torticollis and lack of coordination. These signs are associated with bacterial infections, which result in higher mortality than following aMPV infection alone. In layer and breeder hens, the infection is associated with a decrease in egg production and hatchability, changes in egg shell and internal egg quality and temporary respiratory signs (Cook, Citation2000a, Citationb; Cook et al., Citation2000). aMPV belongs to the Paramyxoviridae family, Pneumovirinae subfamily and Metapneumovirus genus (Wang et al., Citation2011). Four subtypes of aMPV have been recognized: subtypes A and B were identified in South Africa and Europe, subtype C was originally detected in the USA, and subtype D was found in France (Bayon-Auboyer et al., Citation2000; Seal et al., Citation2000; Toquin et al., Citation2000; Govindarajan et al., Citation2006). In Latin America, subtypes A and B have been detected predominantly in Brazil (Dani et al., Citation1999; Chacon et al., Citation2007). In Mexico, aMPV-positive serology has been reported since the beginning of the 1990s, but aMPV detection or isolation has not yet been achieved (Lucio et al., Citation1991; García et al., Citation1994; Valladares et al., Citation1996). At the present time, clinical problems and positive serology are being observed, suggesting the presence of aMPV in Mexico.
The objective of the present study was to detect and isolate aMPV in pullets and breeder hens in two zones of high poultry production in Mexico.
Materials and Methods
Sampling
Longitudinal sampling was carried out in two highly productive zones of poultry farming in Mexico between July 2007 and November 2008. Samples were collected from 48 flocks in 10 farms of breeder hens and pullets in the states of Puebla and Jalisco. Clinical signs observed previously in these farms were characterized by respiratory signs, rhinitis, sinusitis, coughing and decreased egg production. None of the flocks analysed had a history of vaccination against aMPV. At the time of sample collection, there was no aMPV vaccine available in Mexico.
Serology
Initially, a total of 968 serum samples were collected from pullets and hens 2 to 73 weeks of age (418 birds from the state of Puebla and 550 birds from the state of Jalisco). The presence of antibodies specific to aMPV (A and B subtypes) in serum was evaluated using a quantitative commercial indirect enzyme-linked immunosorbent assay kit (FlockChek; IDEXX Laboratories Inc., Westbrook, ME, USA Maine, USA). The test was performed according to the manufacturer's directions. Antibody titres were expressed as a sample-to-positive (S/P) ratio according to the following formula:
Primary culture of trachea and lung of chicken embryos
To obtain primary cultures, 17-day-old specific pathogen free chicken embryos were used. These were refrigerated at 4°C for 1 h, and then the trachea and lungs of each embryo were collected aseptically. Disaggregation of tissues was achieved by incubation with a trypsin–ethylenediamine tetraacetic acid solution for 30 min at 37°C using a mechanical stirrer. The cell suspension was filtered through sterile gauze and centrifuged at 600 × g for 10 min. The pellet was suspended in 10 ml Dulbecco's modified Eagle's medium/nutrient mixture F12 Ham (Sigma-Aldrich, St Louis, Missouri, USA) adding approximately 1 × 106 cells/ml. Cultures were maintained in Dulbecco's modified Eagle's medium/nutrient mixture F12 Ham, supplemented with 10% foetal bovine serum for 24 to 48 h at 37°C in a 5% carbon dioxide atmosphere.
Virus isolation
Once the seroprevalence for aMPV had been determined in the analysed flocks, a total of 105 tracheal tissue samples were collected from 3-week-old to 9-week-old pullets (75 birds from the state of Puebla, and 30 birds from the state of Jalisco). The samples were preserved in liquid nitrogen from the moment of collection until analysis, when they were thawed at environmental temperature. Tissues were pooled by week of age in each flock (five samples per pool), and samples were then macerated in a mortar with cell culture medium supplemented with antibiotics and a fungicide (100 iu/ml penicillin, 100 µg/ml streptomycin, 12.5 iu/ml nystatin and 100 µg/ml gentamycin), keeping a 1:10 w/v ratio. The macerate obtained was centrifuged at 200 × g for 20 min and the supernatant was filtered through 0.45 mm sterile nitrocellulose membranes. One millilitre of the supernatant was inoculated into a mixed primary culture of lung and trachea from 17-day-old specific pathogen free embryonated chicken eggs maintained in 25-cm2 bottles. Adsorption of the inoculum was performed for 2 h at 37°C with 5% carbon dioxide. The inoculum was then removed and fresh culture medium was added with 2% bovine foetal serum. The samples were observed daily under a microscope for cytopathic effect (CPE) for 120 h. After four blind passages, the supernatants from the last passage were inoculated onto Vero cells (green monkey kidney cells) for an additional four passages.
Indirect immunofluorescence
Indirect immunofluorescence (IF) was performed on Vero cell cultures infected with supernatants from the fourth primary culture passage. Vero cell monolayers in 96-well plates were inoculated with 50 µl diluted supernatant (1:5 v/v). Adsorption of the inoculum was performed for 2 h at 37°C with 5% carbon dioxide. The inoculum was then removed and 150 µl fresh culture medium were added with 2% bovine foetal serum. The inoculated cultures were incubated for 48 h at 37°C. Cultures contained in the plates were fixed with 4% paraformaldehyde. For the IF test, an anti-aMPV rabbit polyclonal purified antiserum (Nobilis TRT Inac; Intervet, Milton Keynes, Bucks, UK) was used as the primary antibody. The secondary antibody was anti-rabbit IgG produced in goats and conjugated with fluorescein isothiocyanate (Zymed Labs, Invitrogen, Carlsbad, CA, USA). Cell cultures were considered positive if they exhibited intense intracytoplasmic granular fluorescence.
Nested reverse transcriptase-polymerase chain reaction
Total RNA was extracted from tracheal tissues (n = 21 pools of samples) and supernatants from Vero cell cultures after the third or fourth passage (n = 12 supernatants) using the TRIzol® LS reagent (Life Technologies Corp., Carlsbad, CA, USA) according to the manufacturer's protocol. RNA was quantified using spectrophotometry at a wavelength of 260 nm (NanoDrop ND-1000, Thermo Fisher Scientific, Wilmington, DE, USA Wilmington, Delaware, USA). The inclusion criterion for nested reverse transcriptase-polymerase chain reaction (nRT-PCR) was an OD260/OD280 ratio greater than 1.8. A total of 500 ng RNA was used for amplification by duplex nRT-PCR, which was conducted as described previously by Juhasz & Easton (Citation1994). The nRT-PCR amplified a fragment from the G gene of aMPV subtype A (268 base pairs [bp]) and subtype B (361 bp). In the first round of amplification, primers G6 − (CTGACAAATTGGTCCTGATT) and G1+ (GGGACAAGTATC T/C C/A T/G AT) amplified an internal 444 bp fragment of the G gene. In the nested round, primer G5– (CAAAGA A/G CCAATAAGCCCA) was used for the two subtypes. The forward primer G8+A (CACTCACTGTTAGCGTCATA) was used to amplify subtype A and the primer G9+B (TAGTCCTCAAGCAAGTCCTC) was used for subtype B. All primers have been published previously (Naylor et al., Citation1997). PCR products were subjected to 2% agarose gel electrophoresis, stained with ethidium bromide and photographically documented.
DNA sequencing and phylogenetic analysis
The amplified products were identified in an agarose gel and purified with the QIAquick Gel Extraction Kit (Qiagen, Dusseldorf, Germany). Purified products were sequenced in both directions using an ABI Prism 3100 Genetic Analyzer with the BigDye® Terminator v2.0 Kit (Applied Biosystems, Foster City, California, USA) in the Instituto de Biología, Universidad Nacional Autónoma de México. The sequences obtained were compared with the GenBank database using BLAST software (http://blast.ncbi.nlm.nih.gov/Blast.cgi) from the National Center for Biotechnology Information (Zhang et al., Citation2000; Morgulis et al., Citation2008). The sequences were edited with BioEdit software v. 7.1.11 (Hall, Citation1999) and aligned using the clustalW software included in the BioEdit software package. To determine the relationships among the aMPV strains, a phylogenetic tree was constructed by the neighbour-joining method (Kimura two-parameter model for nucleotide sequences) with 1000 bootstrap replicates, using the MEGA v.5.0 software package (Tamura et al., Citation2011). For analysis, we used the five sequences obtained in this study and 19 aMPV subtype A sequences, in addition to subtype B, C, and D sequences, retrieved from GenBank. The sequences identified in this study have been submitted to GenBank under accession numbers JN041207.1 and KC867702.1 to KC867705.1.
Statistical analysis
Serum antibody titres were transformed using a log2 transformation. We used a linear model, which included the linear and quadratic effects of weeks of age, to analyse the trend in reactive serum titres across weeks of age in the analysed flocks. All statistical analyses were performed using the JMP® 9.0 statistical software package (SAS Institute, Inc., Cary, North Carolina, USA).
Results
Seroprevalence
The enzyme-linked immunosorbent assay results showed that 2-week-old and 5-week-old chickens had low percentages of birds positive for aMPV antibodies (7.1% and 18.7%, respectively) ( and ). At 7 weeks, 42.8% had detectable aMPV antibodies, and 71.1% of the 10-week-old chickens presented as seropositive. We found that 12-week-old chickens were the youngest group where 100% of the samples had aMPV antibodies. From week 12 onwards, the vast majority of chickens were positive for aMPV antibodies; the only groups without 100% positivity were chickens at 23, 26, and 33 weeks of age (where 88.8%, 95.2%, and 91.8% were seropositive, respectively) ( and ). Statistical analyses revealed differences between the antibody titre least-square means of the two analysed groups (P > 0.0001) (log2 8.85 compared with log2 11.51 for Jalisco and Puebla, respectively). Although antibody titres were different in the analysed populations, the seroconversion trend was similar (R 2 = 32.7%). Linear and quadratic effects of weeks of age of the flocks on the antibody titre trend were both significant and similar in the two populations analysed (P < 0.0001), but the intercept of the two populations differed statistically (P < 0.001) ().
Table 1. Seroprevalence of aMPV in pullets and breeder hens in the state of Jalisco, Mexico.
Table 2. Seroprevalence of aMPV in pullets and breeder hens in the state of Puebla, Mexico.
Virus isolation
Samples analysed in primary mixed embryo lung and trachea cultures did not present CPE in any of the passages. However, after adaptation to Vero cells, CPE was evident from the first and second passages after a 96 h incubation period. The CPE was characterized by the formation of syncytia (). None of the culture supernatants showed haemagglutination with chicken erythrocytes. Cultures were considered negative if no CPE was observed during the fourth passage in Vero cells. Positive samples (n = 5 pools) corresponded to tissues from 3-week-old, 7-week-old and 8-week-old chickens from two different flocks in the state of Jalisco and to tissues from 8-week-old chickens from one flock in the state of Puebla. An abnormal CPE was observed in one sample. Evaluation of this sample did not reveal positivity for aMPV by either IF or nRT-PCR. This situation is feasible due to the possible presence of another viral agent. This sample was discarded and not examined further. Cell culture plates from the fourth passage in primary cultures were analysed by indirect IF to confirm the presence of aMPV in Vero cell cultures ().
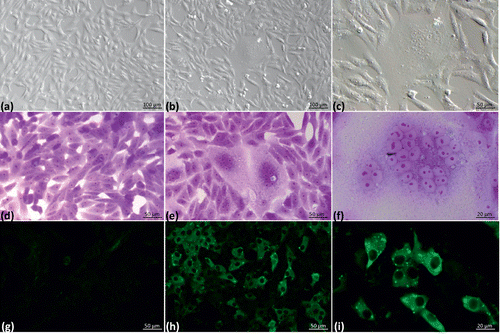
Table 3. Number of aMPV nRT-PCR-positive samples and positive virus isolates (identified by cytopathic effect and indirect immunofluorescence) in samples collected from chicken frocks in Jalisco and Puebla states, Mexico.
Indirect immunofluorescence
The supernatant from the fourth primary culture passage was used to infect Vero cell cultures, and the presence of Vero cells with intracytoplasmic granular fluorescence (a characteristic of aMPV in cell culture) was investigated (). The incubation time (48 h) was sufficient to show the presence of aMPV in culture by indirect IF before CPE was observed. Samples positive by IF were consistent with those in which CPE was observed ( and ).
Nested reverse transcriptase-polymerase chain reaction
In the tracheal tissue pools (21 pools; each pool consisted of five tracheal tissues), 23.8% were nRT-PCR-positive. Positive samples were found in both states. The samples correspond to 3-week-old, 7-week-old and 8-week-old chickens from the state of Jalisco and to 8-week-old chickens from the state of Puebla. All positive samples isolated in cell cultures were positive by RT-PCR. The products observed on agarose agar electrophoresis corresponded to an approximate size of 268 bp, indicating that the viral genomes belonged to a subtype A strain. The supernatants from the third and fourth Vero culture passages from positive trachea samples were also found to be positive by nRT-PCR, with a similar size product ().
DNA sequencing and phylogenetic analysis
From the Vero cell culture passages that were positive for aMPV by nRT-PCR, we sequenced the aMPV strains. The following five virus isolates were named:
aMPV/A/chicken/Mx/Jal/2008 (accession number: JN041207.1),
aMPV/A/chicken/Mx/Jal/02/2008 (accession number: KC867702.1),
aMPV/A/chicken/Mx/Jal/03/2008 (accession number: KC867703.1),
aMPV/A/chicken/Mx/Jal/04/2008 (accession number: KC867704.1), and
aMPV/A/chicken/Mx/Pue/01/2008 (accession number: KC867705.1).
The sequences of these isolates indicate 98 to 99% similarity with 16 sequences of the G gene for aMPV subtype A viruses published in GenBank with the following accession numbers: AM778597.1 (aMPV/A/silky chicken/CHN/6342/2004); AM778598.1
(aMPV/A/chicken/CHN/6631/2004); AY734531.1 (TRTV/BR/169/2005); AY739717.1 (aMPV/A/BR/SHSBR668/2003);
AY739718.1 (aMPV/A/BR/SHSBR668/2003);
AY739719.1 (aMPV/A/BR/SHSBR669/2003);
AY842242.1(aMPV/A/chicken/BR/119/1995); AY842243.1 (aMPV/A/chicken/A/BR/121/1995); FJ796705.1 (aMPV/A/chicken/Korea/301/2008);
FJ796703.1 (aMPV/A/chicken/Korea/1336/2006); JF793649.1 (aMPV/A/GER/GB41/2009);
JF793650.1 (aMPV/A/GER/GB755/2008); JF793651.1 (aMPV/A/GER/GB1133/2007);
L34032.1 (TRTV/UK/CVL14/1/1988); L34030.1 (TRTV/FR/1556/1988); S40185.1 (TRTV/UK/1992); DQ666911.1 (aMPV/A/8544; vaccine progenitor strain); JF424833.1 (aMPV/IT/Ty/A/259-01/03; virulent strain from vaccine); and FJ828954.1 (aMPV/A/2007; vaccine strain). Of these sequences, 12 correspond to sequences obtained from chickens and seven from turkeys. Among the chicken sequences, five are aMPV sequences obtained from Brazil. A phylogenetic tree based on a partial sequence of the G gene with these published sequences from aMPV subtypes A, B, C and D showed that the Mexican strains are most closely related to the Chinese and Brazilian subtype A strains but with a common ancestor of European origin ().
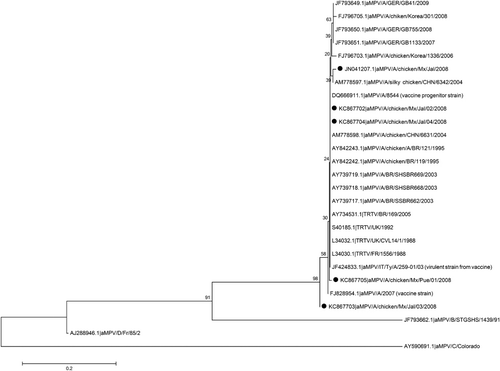
Discussion
Turkey rhinotracheitis was first described in South Africa in 1978 and was later identified in the UK, France, Spain, Germany, Italy, Ireland and Israel (Jones, Citation1996). The first reported presence in Latin America was by D'Arce et al. (Citation2005) from Brazil, who reported the isolation of aMPV from field samples using chicken embryo-related cells. In the present study, aMPV antibodies were detected and isolation of aMPV was possible from samples of breeder pullets collected in 2008. This is the first time that aMPV has been isolated in Mexico.
By means of the serological investigation we carried out, this study generated serological profiles of chicken flocks located in the states of Jalisco and Puebla in Mexico in order to determine the decrease of passive immunity and the onset of aMPV infection in these two states. In this serological survey, we confirmed the presence of aMPV antibodies in pullets and hens. Positive chickens were first detected at 2 weeks of age, probably due to the presence of maternal antibodies. At 3 weeks of age, chickens were negative for aMPV antibodies, indicating the decline of maternal immunity. At 5 weeks, a low percentage of positive samples was observed (18.7%). With increase in age, the number of chickens with antibodies against aMPV increased following a positive linear and a negative quadratic trend (). By investigating seroprevalence over time, we were able to identify the moment when maternal antibody had declined to a level where the pullets were most susceptible to aMPV infection and we hypothesized, successfully, that this could be the optimal time to attempt virus isolation.
Isolation of aMPV is extremely difficult because the virus is present in tissues and excretions for a very short time after infection, and consequently it is hard to establish the time of onset of infection and therefore the time to sample in a field situation. Furthermore, identification of the virus by RT-PCR and its isolation become very difficult after the fourth day of infection (Cook et al., Citation1993, Citation2001). If virus isolation is attempted from field material it is advisable to first perform a serological profile in order to identify the decrease of passive immunity and the start of virus circulation in the field. By means of serology, it is possible to identify the point at which chickens are susceptible to infection and thus when the virus might be found in the target organ. In this case, the pullets from these flocks did not show any associated clinical signs and the five pools that were aMPV-positive coincided with the decrease of passive immunity. Previous studies in Mexico have demonstrated the presence of aMPV-specific antibodies. Lucio et al. (Citation1991) reported cases that were clinically compatible with the first positive serology for aMPV in 31-week-old to 33-week-old heavy breeder chickens. Valladares et al. (Citation1996) indicated that, on average, by 6.6 weeks of age there is positive serological evidence for the presence of aMPV in broilers, and by 10 weeks of age in breeder flocks. In each case, the clinical signs associated with aMPV were related to swollen head syndrome. The data obtained from the present work show that 42.8% of 7-week-old chickens have detectable aMPV antibodies. This result is similar to that observed by García et al. (Citation1994), who identified seropositivity in broilers at 7 weeks of age. Under the sampling conditions and the serological analysis in this study, we found the time of 100% seroconversion to be at 12 weeks of age. The statistical analysis showed that titre trend across weeks of age was similar in both states of the country, while differing in the titre average (see parallel curves in ). Given these serological results, we decided to attempt virus isolation from respiratory tract tissues of pullets aged 3 to 9 weeks.
Conventionally, the recommended culture systems to attempt isolation of aMPV, include the vitelline sac of chicken embryos inoculated at 6 days and harvested after 8 days, or tracheal organ cultures (TOC) derived from chicken or turkey embryos (Cook, Citation2000b). Replication of aMPV in cultures of ex vivo tissues such as TOC is an effective method to perform virus isolation by evaluating the ciliostasis that can be observed 3 to 5 days post infection (Cook, Citation2000b). However, the disadvantages of using TOC are that the TOC should be homogeneous; the reading of the ciliostasis may be subjective, and, to avoid the deposition of cellular residue in the tracheal ring lumen, the TOC must be maintained in constant motion. The disadvantage of inoculating the vitelline sac of embryos is that the inoculum might not be correctly deposited on the membrane.
In this study, we decided to use primary cultures of trachea and lungs taken from 17-day-old specific pathogen free embryos. We did this because it had been shown previously (Zaffuto et al., Citation2008) to be a good system used successfully for the isolation of other respiratory viruses of chickens, namely avian influenza and Newcastle disease viruses. Also, it has been proven previously that in vitro infection of primary small alveolar epithelial cells and normal bronchial epithelial cells with human metapneumovirus yield infection indices of 80 to 90% compared with those of the A549 cell line (human alveolar type-II-like epithelial cell line) (Bao et al., Citation2007). The advantage of performing aMPV isolation using primary trachea and lung cultures is that the organs are processed more quickly and easily because tissue integrity does not have to be maintained, as the whole epithelium is disaggregated at the time of incubation. The primary culture, by being a mixture of cell types, reproduces more closely the characteristics of an in vivo tissue. In cell lines, by increasing the number of passages, the expression of receptors can be modified, thus making infection by field samples less efficient.
There are no previous reports on the use of primary cultures of respiratory organs for attempted isolation of aMPV. However, based on the work of Zaffuto et al. (Citation2008), our hypothesis was that aMPV could be isolated using primary cultures of chicken embryo trachea and lung. This hypothesis proved to be correct. Once aMPV replication was obtained in primary cultures, virus adaptation to Vero cells was conducted. We observed CPE from five pools of samples from 3-week-old, 7-week-old and 8-week-old chickens at 96 and 120 h post inoculation in the first and second Vero cell passages. We found that indirect IF was a more efficient tool than CPE observation for aMPV detection because it was possible to detect positive cultures within 48 h of incubation. Jirjis et al. (Citation2002) detected aMPV after 24 h of incubation; however, this result was influenced by virus load in the cell cultures. In our study, the age of positive chickens (7 weeks old) corresponded to the time of highest susceptibility to aMPV infection because passive immunity had decreased, but less than 50% of pullets had antibodies against the virus. Under experimental conditions, Cook et al. (Citation2000) reported that in chickens inoculated via the ocular-nasal route it was possible to detect antibodies against aMPV at 14 days post infection, which is consistent with the results obtained from the serological analysis in the present study. Under field conditions in the UK, Cavanagh et al. (Citation1999) detected positive chickens by nRT-PCR between the ages of 5 and 7 weeks. These data are consistent with our study, as we found positive pullets by nRT-PCR at 3 to 8 weeks of age.
The main aMPV antigens are the external glycoproteins F and G. Based on sequence analysis and G glycoprotein antigenicity, two subtypes (A and B) were initially defined. These subtypes have only 38% amino acid identity. The existence of a wider diversity of aMPV isolates was appreciated, and a new subtype known as D was defined (Bayon-Auboyer et al., Citation2000; Govindarajan et al., Citation2006). In the USA, aMPV was not reported until 1996. The genetic sequence analysis of the isolations obtained in the USA indicated that they belonged to a new subtype known as C, which differed from subgroups A, B and D, which were all isolated in Europe (Seal, Citation1998; Seal et al., Citation2000). In the present study, sequence analysis of the aMPV isolates from tracheal samples and cultures show a 98 to 99% nucleotide sequence homology with aMPV subtype A sequences. The partial G gene sequences analysed in this study showed a distinct branching of subtype A viruses away from the other subtypes. In the Americas, studies of aMPV have only reported the presence of subtypes A and B in Brazil and subtype C in the USA (Dani et al., Citation1999; Seal et al., Citation2000). The sequences we obtained in this study indicate a close relation to the aMPV sequences from Brazil, but with a common ancestor in European strains (the UK and France). The origin of this aMPV subtype A circulating in Mexico could be from the importation of the progenitor in breeder birds coming from countries where the presence of this subtype is common. According to the proposal by Lupini et al. (Citation2011), the origin of the isolated virus is unlikely to be from vaccines or from vaccine strains that reverted to virulence. Analysis of the partial sequence of the gene G revealed that no mutation occurred in position 6358, which would indicate that the isolates reported in this study are of field origin (). In conclusion, the results obtained in the present study, reporting the isolation of aMPV subtype A in Mexico, demonstrate for the first time the presence of aMPV in flocks of layer pullets in two regions of high poultry production in this country.
Table 4. Nucleotide sequences of the partial G gene (6352 to 6364 nucleotides) of different aMPV subtype A strains demonstrating that the virus isolated in this work is not a vaccine strain or a virulent strain derived from a vaccine strain.
Conflicts of interest
The authors affirm that no financial or personal relationships exist that could have inappropriately influenced the content of this manuscript or the opinions expressed.
Acknowledgements
The authors wish to thank to Héctor Castillo-Juárez for his help regarding statistical analyses, José Manuel Saavedra-Montañez (FMVZ-UNAM) and Federico Vera (INTA-EEA, Argentina) for their aid in the interpretation of our results, and Laura Marquez Valdelamar (Instituto de Biología, UNAM) for technical assistance in the sequence analysis. The present study was funded by PAPIIT-IN208814-3. J. F. Rivera-Benitez is the recipient of a grant from the Consejo Nacional de Ciencia y Tecnología (CONACYT; ID 207402).
References
- Bao, X., Liu, T., Spetch, L., Kolli, D., Garafolo, R. & Casola, A. (2007). Airway epithelial cell response to human metapneumovirus infection. Virology, 368, 91–101. 10.1016/j.virol.2007.06.023
- Bayon-Auboyer, M.H., Arnauld, C., Toquin, D. & Eterradossi, N. (2000). Nucleotide sequences of the F, L and G protein genes of two non-A/non-B avian pneumoviruses (APV) reveal a novel APV subgroup. Journal of General Virology, 81, 2723–2733.
- Buys, S.B., du Preez, J.H. & Els, H.J. (1989). Swollen head syndrome in chickens: a preliminary report on the isolation of a possible aetiological agent. Journal of South African Veterinary Association, 60, 221–222.
- Cavanagh, D., Mawditt, K., Britton, P. & Naylor, C. (1999). Longitudinal field studies of infectious bronchitis virus and avian pneumovirus in broilers using type-specific polymerase chain reactions. Avian Pathology, 28, 593–605. 10.1080/03079459994399
- Cook, J.K.A., Kinloch, S. & Ellis, M.M. (1993). In vitro and in vivo studies in chickens and turkeys on strains of turkey rhinotracheitis virus isolated from the two species. Avian Pathology, 22, 157–170. 10.1080/03079459308418907
- Cook, J.K.A. (2000a). Avian pneumovirus infections of turkeys and chickens. Veterinary Journal, 160, 118–125.
- Cook, J.K.A. (2000b). Avian rhinotracheitis. Revue Scientifique et Technique, 19, 602–613.
- Cook, J.K.A., Chesher, J., Orthel, F., Woods, M.A., Orbell, S.J., Baxendale, W. & Huggins, M.B. (2000). Avian pneumovirus infection of laying hens: experimental studies. Avian Pathology, 29, 545–556. 10.1080/03079450020016788
- Cook, J.K.A., Huggins, M.B., Orbell, S.J., Mawditt, K. & Cavanagh, D. (2001). Infectious bronchitis virus vaccine interferes with the replication of avian pneumovirus vaccine in domestic fowl. Avian Pathology, 30, 233–242. 10.1080/03079450120054640
- Cook, J.K.A. & Cavanagh, D. (2002). Detection and differentiation of avian pneumoviruses (metapneumoviruses). Avian Pathology, 31, 117–132. 10.1080/03079450120118603
- Chacon, J.L., Brandao, P.E., Buim, M., Villarreal, L. & Ferreira, A.J. (2007). Detection by reverse transcriptase-polymerase chain reaction and molecular characterization of subtype B avian metapneumovirus isolated in Brazil. Avian Pathology, 36, 383–387. 10.1080/03079450701589142
- D'Arce, R.C., Coswig, L.T., Almeida, R.S., Trevisol, I.M., Monteiro, M.C., Rossini, L.I., Di Fabio, J., Hafez, H.M. & Arns, C.W. (2005). Subtyping of new Brazilian avian metapneumovirus isolates from chickens and turkeys by reverse transcriptase-nested-polymerase chain reaction. Avian Pathology, 34, 133–136. 10.1080/03079450500059180
- Dani, M.A., Durigon, E.L. & Arns, C.W. (1999). Molecular characterization of Brazilian avian pneumovirus isolates: comparison between immunochemiluminescent Southern blot and nested PCR. Journal of Virological Methods, 79, 237–241. 10.1016/S0166-0934(99)00020-8
- García, F.A., Ávila, F.D., Padilla, R.I. & Rodríguez, V.Y. (1994). Descripción y etiologías de un brote con signos de cabeza hinchada en pollo de engorda [Etiology and description of outbreak of broilers with swollen head syndrome]. In ANECA. (ed). Memorias de la XIX Convención Nacional ANECA [Proceedings of the XIX ANECA National Convention] (pp. 94–96). Puerto Vallarta, Mexico.
- Govindarajan, D., Buchholz, U.J. & Samal, S.K. (2006). Recovery of avian metapneumovirus subgroup C from cDNA: cross-recognition of avian and human metapneumovirus support proteins. Journal of Virology, 80, 5790–5797. 10.1128/JVI.00138-06
- Hall, T.A. (1999). BioEdit: a user-friendly biological sequence alignment editor and analysis program for Windows 95/98/NT. Nucleic Acids Symposium Series, 41, 95–98.
- Jirjis, F.F., Noll, S.L., Halvorson, D.A., Nagaraja, K.V., Townsend, E.L., Goyal, S.M. & Shaw, D.P. (2002). Rapid detection of avian pneumovirus in tissue culture by microindirect immunofluorescence test. Journal of Veterinary Diagnostic Investigation, 14, 172–175. 10.1177/104063870201400216
- Jones, R.C. (1996). Avian pneumovirus infection: questions still unanswered. Avian Pathology, 25, 639–648. 10.1080/03079459608419171
- Juhasz, K. & Easton, A.J. (1994). Extensive sequence variation in the attachment (G) protein gene of avian pneumovirus: evidence for two distinct subgroups. Journal of General Virology, 75, 2873–2880. 10.1099/0022-1317-75-11-2873
- Lucio, D.E., Cortés, M.E. & Le Gros, F.X. (1991). Presentación del Síndrome de la cabeza hinchada en parvadas de aves reproductoras pesadas en México [Swollen Head Syndrome in heavy breeder flocks in Mexico]. In ANECA. (ed). Memorias de la XVI Convención Nacional ANECA [Proceedings of the XVI ANECA National Convention] & 40th WPDC (pp. 158–161). Acapulco, Mexico.
- Lupini, C., Cecchinato, M., Ricchizzi, E., Naylor, C.J. & Catelli, E. (2011). A turkey rhinotracheitis outbreak caused by the environmental spread of a vaccine-derived avian metapneumovirus. Avian Pathology, 40, 525–530. 10.1080/03079457.2011.607428
- Morgulis, A., Coulouris, G., Raytselis, Y., Madden, T.L., Agarwala, R. & Schaffer, A. A. (2008). Database indexing for production MegaBLAST searches. Bioinformatics, 24, 1757–1764. 10.1093/bioinformatics/btn322
- Naylor, C., Shaw, K., Britton, P. & Cavanagh, D. (1997). Appearance of type B avian pneumovirus in Great Britain. Avian Pathology, 26, 327–338. 10.1080/03079459708419215
- Seal, B.S., (1998). Matrix protein gene nucleotide and predicted amino acid sequence demonstrate that the first US avian pneumovirus isolate is distinct from European strains. Virus Research, 58, 45–52. 10.1016/S0168-1702(98)00098-7
- Seal, B.S., Sellers, H.S. & Meinersmann, R.J. (2000). Fusion protein predicted amino acid sequence of the first US avian pneumovirus isolate and lack of heterogeneity among other US isolates. Virus Research, 66, 139–147. 10.1016/S0168-1702(99)00133-1
- Tamura, K., Peterson, D., Peterson, N., Stecher, G., Nei, M. & Kumar, S. (2011). MEGA5: molecular evolutionary genetics analysis using maximum likelihood, evolutionary distance, and maximum parsimony methods. Molecular Biology and Evolution, 128, 2731–2739. 10.1093/molbev/msr121
- Toquin, D., Bayon-Auboyer, M.H., Senne, D.A. & Eterradossi, N. (2000). Lack of antigenic relationship between French and recent North American non-A/non-B turkey rhinotracheitis viruses. Avian Diseases, 44, 977–982. 10.2307/1593075
- Valladares, J.C., Barrientos, A., Angulo, B. & Juárez, D. (1996). Detección serológica del VTRT y su probable relación con enfermedad respiratoria en el noreste de México [Serological detection of TRTV and probably related to respiratory disease in the northeast of Mexico]. In ANECA. (ed). Memorias de la XXI Convención Nacional ANECA [Proceedings of the XXI ANECA National Convention] & 45th WPDC (pp. 338–340). Cancun, Mexico.
- Wang, L-F., Collins, P.L., Fouchier, R.A.M., Kurath, G., Lamb, R.A., Randall, R.E., & Rima, B.K. (2011). Paramyxoviridae. In King, A.M.Q., Adams, M.J., Carstens, E.B., Lefkowitz, E.J. (eds). Virus taxonomy: classification and nomenclature of viruses. Ninth Report of the International Committee on Taxonomy of Viruses (pp. 640–653). San Diego, CA: Elsevier Academic Press
- Zaffuto, K.M., Estevez, C.N. & Afonso, C.L. (2008). Primary chicken tracheal cell culture system for the study of infection with avian respiratory viruses. Avian Pathology, 37, 25–31. 10.1080/03079450701774850
- Zhang, Z., Schwartz, S., Wagner, L. & Miller, W. (2000). A greedy algorithm for aligning DNA sequences. Journal of Computational Biology, 7, 203–214. 10.1089/10665270050081478