Abstract
The molecular diversity in S3 gene sequences of turkey reovirus (TRV) was determined in poult enteritis syndrome (PES)-affected and apparently healthy turkey poults. Twenty-nine TRV-positive samples (15 from PES-affected flocks and 14 from apparently healthy flocks) were tested using self-designed primers for the S3 gene. Phylogenetic analysis revealed that the TRV S3 sequences of this study clustered in clade III and formed two different groups in this clade. The avian reoviruses from duck and goose formed clade I and those from chickens formed clade II. The clade III TRV sequences had a nucleotide percent identity of 88.9 to 100% among themselves but only of 59.5 to 63.5% and 69.2 to 72.6% with clades I and II, respectively. More amino acid substitutions were present in TRVs from PES-affected flocks than in those from apparently healthy flocks using ATCC VR-818 (AY444912) as a benchmark. All TRVs of this study showed substitutions at positions 244 and 285. The impact of these changes on the virulence of the virus, if any, needs to be studied.
Introduction
Avian orthoreovirus (ARV) is a member of the genus Orthoreovirus, family Reoviridae. The International Committee on Taxonomy of Viruses separates Orthoreovirus into many different species including mammalian orthoreovirus, avian orthoreovirus, aquareovirus, baboon orthoreovirus, Nelson Bay orthoreovirus, and reptilian orthoreovirus (King et al., Citation2012). The reoviruses contain 10 double-stranded RNA (dsRNA) segments enclosed in a non-enveloped icosahedral double capsid (Spandidos & Graham, Citation1976). The 10 genomic segments are separated into three size classes—large (L1, L2, L3), medium (M1, M2, M3), and small (S1, S2, S3, S4)—on the basis of their electrophoretic mobility in agarose gel (Spandidos & Graham, Citation1976). The proteins encoded by the L, M, and S genes are designated lambda (λ), mu (µ), and sigma (σ), respectively. The avian reovirus genome expresses at least eight structural proteins (λA, λB, λC, µA, µB, σA, σB, and σC) and four non-structural proteins (µNS, P10, P17, and σNS) (Varela & Benavente, Citation1994; Varela et al., Citation1996; Martinez-Costas et al., Citation1997).
The major component of the reovirion outer capsid, σB protein, contains group-specific neutralizing epitopes, is encoded by the ARV S3 gene segment, and is analogous to the mammalian reovirus S4 segment encoding the σ3 protein (Wickramasinghe et al., Citation1993; Varela et al., Citation1996). The outer capsid protein σC is encoded by the ARV S1 gene, which is used for viral attachment. The non-structural protein σNS is encoded by the ARV S4 genome segment (Schnitzer, Citation1985; Varela & Benavente, Citation1994) and binds single-stranded RNA in vitro in a sequence-independent manner (Yin & Lee, Citation1998; Touris-Otero et al., Citation2005). Unlike the mammalian reovirus genome, the ARV genome is able to induce cell fusion but lacks haemagglutination activity (Glass et al., Citation1973; Bodelon et al., Citation2001; Nibert & Schiff, Citation2001).
Avian reovirus is an important cause of diseases in chickens and turkeys and is involved in myocarditis, viral arthritis, infectious tenosynovitis, malabsorption, runting and stunting syndrome, and respiratory and enteric diseases (Glass et al., Citation1973; Hieronymus et al., Citation1983, Rosenberger, Citation2003; Pantin-Jackwood et al.., Citation2008; Jindal et al., Citation2010a; Mor et al., Citation2013a, Citationb; Moura-Alvarez et al., Citation2013). The reoviruses detected in turkeys are commonly called turkey reoviruses (TRVs) as opposed to ARV in chickens. The TRV is often isolated from cases of turkey enteritis and tenosynovitis resulting in considerable economic losses to turkey growers (Heggen-Peay et al., Citation2002; Mor et al., Citation2013a). Using ARV S4 gene primers in a reverse-transcription-polymerase chain reaction (RT-PCR), we have detected TRVs in cases of poult enteritis syndrome (PES) and also in apparently healthy breeder turkey flocks (Jindal et al., Citation2010a, Citationb). In this study, we investigate the S3 gene molecular diversity of TRVs detected from both PES-affected and apparently healthy turkey flocks in Minnesota.
Materials and Methods
Samples
Intestinal contents were collected from PES-affected turkeys in Minnesota from 2007 to 2011. A flock was characterized as having PES if the poults exhibited clinical signs of lethargy, depression, and diarrhoea, or pathological changes of thin-walled intestines with watery contents. Intestinal contents collected from an individual flock were pooled to make a single pooled sample per flock. Phosphate-buffered saline (pH 7.4) was added to the pooled sample to make a 10% suspension, which was homogenized and then centrifuged at 2500 × g for 20 min. The supernatant was collected and tested for the presence of TRV by RT-PCR using S4 gene-specific primers (Jindal et al., Citation2010a). The 15 TRV-positive sample pools from PES flocks were tested further using the ARV S3 gene-specific primers.
In addition, 193 faecal samples were collected from five apparently healthy breeder turkey flocks from Minnesota. The details of sample collection and processing have been described previously (Jindal et al., Citation2010b). Briefly, five faecal samples each were collected from eight different areas of a barn, at five different time points. The five faecal samples from each area were pooled and processed for the detection of TRVs using the S4 gene-specific primers (Jindal et al., Citation2010b). The 14 TRV-positive non-PES samples were further tested using ARV S3 gene-specific primers. A total of 29 samples were thus tested with ARV S3 gene-specific primers.
RNA extraction and RT-PCR
From the supernatants of 29 TRV-positive intestinal (from PES-affected turkeys) or faecal (from apparently healthy turkeys) pools, RNA was extracted using TRIzol LS reagent (Invitrogen, Carlsbad, CA, USA). A known isolate of TRV (TX 98) served as a positive control while Newcastle disease virus, avian influenza virus, turkey coronavirus, avian rotavirus, and turkey astrovirus were used to determine the specificity of the RT-PCR. Newcastle disease virus and avian influenza virus were retrieved from the repository of the Minnesota Veterinary Diagnostic Laboratory (St Paul, MN, USA). Avian rotavirus and turkey astrovirus strains were kindly provided by Prof. Y. M. Saif of The Ohio State University (Wooster, OH, USA). The turkey coronavirus (NC95) was a gift from Prof. James Guy of North Carolina State University (Raleigh, NC, USA).
Extracted RNA was subjected to RT-PCR using ARV S3 gene self-designed degenerate primers (forward 36 to 59, 5′-TGAAGGYATTACATCGAGTTACAT-3′ and reverse 1035 to 1012, 5′-AGCTTCGGGTCCACARTCACATAC-3′; corresponding to nucleotide positions of AF465799). The RT-PCR was performed using a QIAGEN OneStep RT-PCR Kit (Qiagen, Valencia, CA, USA). The reaction mix consisted of 1 × RT-PCR reaction buffer, 320 µM of each deoxynucleoside triphosphate, 0.6 µM of each primer, 2 µl enzyme blend, and 4 µl extracted RNA for a total volume of 50 µl. Thermal cycling conditions for RT-PCR were: reverse transcription at 50°C for 30 min, Taq activation at 94°C for 15 min, 35 cycles of denaturation at 94°C for 1 min, annealing at 52°C for 1 min, extension at 72°C for 1 min, and final extension at 72°C for 10 min. The PCR products, approximately 1000 base pairs, were visualized in a 1.2% agarose gel in Tris–acetate–ethylenediamine tetraacetic acid buffer.
Sequencing and phylogenetic analysis
TRV-positive PCR products were purified with the QIAquick PCR purification kit (Qiagen) and were sequenced with previously described primers at the BioMedical Genomic Center, University of Minnesota. The forward and reverse nucleotide trace files were aligned, edited, and curated using Sequencher 5.1 software available from the Minnesota Supercomputing Institute (www.msi.umn.edu). The 29 TRV S3 sequences generated from this study and 35 ARV S3 sequences (with ≥80% of ARV S3 gene) from the National Center for Biotechnology Information (www.ncbi.nlm.nih.gov) were used to conduct phylogenetic analysis (). Additionally, the conserved TRV S3 5′ and 3′ untranslated region nucleotide ends were removed to compare nucleotide and amino acid differences between gene and open reading frame (ORF). The ARV S3 gene and ORF groups were each aligned using Clustal W (Drummond et al., Citation2011). The ARV S3 gene segment and ORF alignments were run with jModelTest (Darriba et al., Citation2012), indicating the maximum likelihood, with a F81 substitution model, as the appropriate phylogenetic analysis model for both datasets. Since the nucleotide percent identity was <1% between the gene segment and ORF alignments, and the F81 was the appropriate substitution model phylogenetic model for the S3 gene and ORF alignments (data not shown), only the ARV S3 ORF data are illustrated here. The sequence data used for phylogenetic analysis were submitted to the GenBank database (). The amino acid histogram was constructed using Geneious Pro (Drummond et al., Citation2011).
Results
S3 phylogeny
The ARV S3 gene degenerate primer set was specific and amplified all 29 turkey samples while not amplifying avian influenza virus, Newcastle disease virus, avian rotavirus, turkey astrovirus and turkey coronavirus. Using the TRV S3 sequences generated from this study (n = 29) and the ARV S3 sequence available from GenBank (n = 35), phylogenetic analysis revealed three distinct TRV clades (). In clade III, the TRV S3 sequences clustered into three groups. Our novel TRV S3 sequences were located in groups 1 and 2. Group 1 contained 14 TRV sequences from apparently healthy flocks and six from PES-affected cases, while group 2 included nine sequences from PES-affected cases and GenBank reference strains TX99, ATCC_VR818, NC98, TX98, and PEMS_85. Group 2 contained sequences only from PES-affected cases, while group 3 contained previously published TRVs (TX98 and PEMS_85). The duck and goose ARV sequences formed clade I while chicken ARV sequences formed clade II.
Clade III TRV sequences had nucleotide percent identity of 88.9 to 100% among themselves but only of 59.5 to 63.5% and 69.2 to 72.6% with clades I and II, respectively (). Clade II had the lowest nucleotide percent identity (83.2 to 100%) among themselves while clade I had the highest nucleotide percent identity (91.4 to 99.6%). In clade III, groups 1, 2, and 3 had nucleotide percent identity of 95.6 to 100%, 89.7 to 100%, and 100%, respectively. Clade III TRV sequences had an amino acid percent identity of 93.5 to 100% among themselves but only of 58.1 to 64.5% and 74.1 to 80.5% with clades I and II respectively ().
Table 1. ARV S3 nucleotide and amino acid percent identities among different clades.
Comparison of TRVs with chicken, duck and goose ARVs
Using ATCC VR-818 (AY444912, a turkey enteric reovirus) as a benchmark, alignment of amino acid sequences (positions 36 to 320) revealed no insertions or deletions in the portion of σB protein studied (). There were 35 amino acid substitutions, of which 14 (Q53H, D112N, E126T, R138S, L157I, D163A, H198N, D201E, T205I, T207A, A219V, K287R, S290A and N294D) were not observed in reoviruses of other avian species used for comparison. Of these 14 substitutions, two (R138S and H198N) were observed in nine and three TRVs of this study, respectively. The remaining 12 substitutions were observed in one or two TRVs of this study.
Table 2. Changes observed at the amino acid level in the turkey reoviruses of this study in comparison with ATCC VR 818.Footnotea
Eleven amino acid substitutions (Y69C, I92V, I110V, D128E, Q146R, K174R, T232D, A233S, L257I, I283V and S307A) observed in the σB protein were similar to those in reoviruses of duck, goose and/or chicken. However, they were not present in previously published TRV strains. Four substitutions (I110V, D128E, Q146R and T232D) were observed in nine, eight, 11, and 17 TRVs of this study, respectively. The remaining 10 amino acid substitutions (A106T, P125S, E167D, K174T, V199I, T232A, A239P, F244L, G285S and E298D) were similar to those of previously published TRV strains (NC/SEP-R44/03, PEMS85, TX98, NC98 and TX99). However, these 10 substitutions were not observed in the original TRV strain, ATCC VR-818 (AY444912) ().
All 29 TRV strains from this study had a conserved CHCC zinc-binding motif within the N terminus at amino acids 51 to 75 with four strains containing amino acid substitution in this region (). The TRV strains TK/MN/D-AB14/2011 and TK/MN/D-AB15/2011 had amino acid substitution from Z to H at position 53, while TRV strains TK/MN/D-AB02/2007 and TK/MN/D-AB05/2008 had a Y to C substitution at position 69. Differences in the CHCC zinc-binding motif were present in the previously published ARV sequences and our 29 TRV strains (). At position 52, clade I strains contained the amino acid T, while clades II and III contained S. At positions 62 and 63, clade II strains contained the amino acids T and L, while clades I and III strains contained the amino acids A and P, respectively. At position 65, clade I strains contained the amino acid T and clade III strains contained the amino acid S, while clade II strains contained the amino acids S, A, or P. At position 69, clade I strains and TRV strains TK/MN/D-AB02/2007 and TK/MN/D-AB05/2008 contained the amino acid C while clade II and the remaining clade III TRV strains contained the amino acid Y.
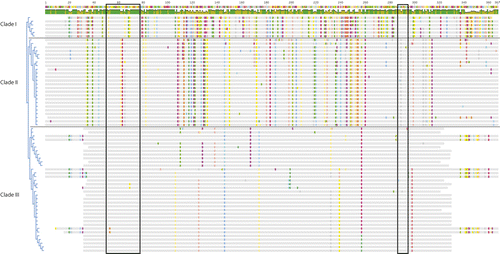
A dsRNA binding region was present at the C-terminus at amino acid positions 287 to 293. All 29 TRV strains from this study contained the conserved dsRNA binding region except for strains TK/MN/D-AB03/2007 and TK/MN/B-AB28/2008, which contained the amino acid R instead of K at position 287 and amino acid A instead of S at position 290 (). All clade II ARV strains used for comparison had the amino acid A while all clade I ARV strains and clade III TRVs had V at position 289.
Comparison of TRVs from PES-affected and apparently healthy flocks
Of the 35 amino acid substitutions in the σB region studied in comparison with AY444912, 22 amino acids were observed in TRV strains from PES-affected flocks while only four substitutions were in TRV strains from apparently healthy flocks (). The remaining substitutions in nine TRV strains involved both PES-affected and apparently healthy flocks. Of the 14 amino acid substitutions observed in TRV strains from this study, none was observed in previously published ARV σB protein sequences. Of these 14 amino acid substitutions, 12 were in TRV strains from PES-affected flocks and the remaining two were in apparently healthy flocks. Eight of the 12 TRV strains from PES-affected flocks contained four amino acid substitutions (I110V, D128E, R138S, and Q146R). The TRV strains with these four amino acid substitutions were in group 2. Also in group 2, the TRV strain TK/MN/D-AB12/2010 from a PES-affected flock contained amino acid substitutions at positions 110, 138, and 146 but not at 128. The amino acid substitutions at positions 110, 128, 138, and 146 were not observed in TRV strains from apparently healthy turkey flocks.
All 14 TRV strains (100%) from apparently healthy flocks had amino acid substitutions at positions 106, 125, 167, 174, 232, 239, and 298, whereas only 20 to 50% of the TRV strains from PES-affected flocks had amino acid substitutions at these positions when compared with ATCC VR-818 (AY444912). All TRV strains from this study contained amino acid substitutions at positions 244 and 285.
Discussion
The present study was conducted to determine the S3 gene molecular diversity in turkey reoviruses. The TRV S3 gene from apparently healthy turkey flocks had a higher nucleotide percent identity than seen with PES-affected flocks. This may be due to the difference in sampling. The sampling of apparently healthy flocks occurred for a total of 9 weeks consecutively and hence there was a higher chance of sampling the same reovirus strain during this short time period. Conversely, sampling from PES-affected flocks occurred over a 5-year period, and the chances of the same TRV strain originating from the same flock were low. Overall, the study revealed higher nucleotide and amino acid similarities with previously published TRV strains than with those of chicken, ducks, and goose reoviruses as has previously been reported (Kapczynski et al., Citation2002; Sellers et al., Citation2004).
The International Committee on Taxonomy of Viruses divides reoviruses into many different species. These viruses are speciated based on >85% amino acid percent identity within a species and <65% between species for the conserved core protein, and amino acid identity of >55% within a species and <35% between species for the outer core proteins (King et al., Citation2012). Additionally, nucleotide percent identity of homologous gene segments should be >75% within a species and <60% between species (King et al., Citation2012). From our TRV sequences and previously published ARV S3 gene sequences, the nucleotide percent identities indicate that turkey, chicken, duck, and goose reoviruses belong to the Orthoreovirus species. Sellers et al. (Citation2004) reported that TRV could potentially be considered a separate Orthoreovirus species. Further, turkey reoviruses when inoculated into chickens failed to produce clinical disease (Day, Citation2009), and a highly pathogenic chicken reovirus strain S1133 only produced mild clinical signs in pathogen-free turkeys and no clinical disease in commercial turkeys (Spackman et al., Citation2005). However, failing to cause clinical disease in another host species does not implicate speciation. Moreover, the lowest amino acid identity among ARV S3 sequence was 56.1%, and the International Committee on Taxonomy of Viruses Orthoreovirus criteria of >55% amino acid identity is still met. Complete genome sequence of multiple ARV strains from different host species is needed to further address the speciation question.
Phylogenetic analysis of the S3 gene revealed that TRV strains cluster independently from chicken, duck, and goose S3 sequences. This may suggest that TRV and ARV are separate Orthoreovirus species (Kapczynski et al., Citation2002; Sellers et al., Citation2004). However, Rosenberger et al. (Citation1989) classified chicken ARV isolates into low, intermediate, or high pathogenicity based on clinical signs, mortality, weight depression, pathology and viral persistence, although these isolates were antigenically similar based on cross-neutralization and cross-protection studies.
Our TRV strains clustered into two different groups within clade III; none of our strains grouped in group 3 of clade III. The TRV σB amino acid differences in this study need to be investigated further. Some of the TRV amino acid substitutions have not been reported previously while the other TRV σB amino acid substitutions match those of duck and goose reoviruses. Future investigations should study the importance of these substitutions. Amino acid differences between PES and apparently healthy flocks were also observed. Point mutations were more common in TRV strains from PES-affected turkeys as compared with those from apparently healthy turkeys. Liu et al. (Citation2003) studied different ARV S-class segments and reported genetic reassortment to be frequent in ARV strains, which may help these viruses to continue circulating. While mutations in ARV S3 gene have been reported earlier (Sellers et al., Citation2004; Kort et al., Citation2013), it is still not known whether the amino acid changes observed in TRV strains of this study affect antigenicity and/or pathogenicity.
The ARV σB protein, like the mammalian reovirus σ3 protein, is composed of two independent functional domains: a zinc-finger motif and a dsRNA binding region (Schiff et al., Citation1988; Le Gall-Recule et al., Citation1999). The σ3 protein putative zinc-finger in the CCHC box (amino acid positions 51 to 73) is believed to increase the stability of reoviruses, which has been reported here and in previously published ARV σB sequences (Mabrouk & Lemay, Citation1994; Le Gall-Recule et al., Citation1999; Kapczynski et al., Citation2002; Sellers et al., Citation2004). The ARV σB protein, like the mammalian reovirus σ3 protein, contains a C-terminal functional domain, which binds dsRNA-binding through a repeated basic amino acid motif (Schiff et al., Citation1988; Miller & Samuel, Citation1992; Kapczynski et al., Citation2002; Sellers et al., Citation2004)). Although the functional activities of ARV σB proteins have not been studied, it is presumed to have the same role as the mammalian σ3.
The present study thus reveals nucleotide and amino acid changes in the S3 gene of TRV strains. The impact of these changes on the virulence of the virus, if any, needs to be studied. Since TRV sequences were geographically limited to the USA, phylogenetic analysis of more geographically diverse sequences would provide additional information on the genetic diversity of TRVs.
Acknowledgements
This study was partially funded by a grant from the Rapid Agricultural Response Fund, University of Minnesota.
References
- Bodelon, G., Labrada, L., Martinez-Costa, J. & Benavente, J. (2001). The avian reovirus genome segment S1 is a functionally tricistronic gene that expresses one structural and two nonstructural proteins in infected cells. Virology, 290, 181–191. 10.1006/viro.2001.1159
- Day, J.M. (2009). The diversity of the orthoreoviruses: molecular taxonomy and phylogenetic divides. Infection Genetics and Evolution, 9, 390–400. 10.1016/j.meegid.2009.01.011
- Darriba, D., Taboada, G.L., Doallo, R. & Posada, D. (2012). jModelTest 2: more models, new heuristics and parallel computing. Nature Methods, 9, 772. 10.1038/nmeth.2109
- Drummond, A.J., Ashton, B., Buxton, S., Cheung, M., Copper, A., Duran, C., Field, M., Held, J., Kearse, M., Markowitz, S., Moir, R., Stones-Havas, S., Sturrock, S., Thierer, T. & Wilson, A. (2011). Geneious v5.4. Available from http://www.geneious.com/
- Glass, S.E., Naqi, S.A., Hall, C.F. & Kerr, K.M. (1973). Isolation and characterization of a virus associated with arthritis of chickens. Avian Diseases, 17, 415–424. 10.2307/1589226
- Heggen-Peay, C.L., Qureshi, M.A., Edens, F.W., Sherry, B., Wakenell, P.S., O'Connell, P.H. & Schat, K.A. (2002). Isolation of a reovirus from poult enteritis and mortality syndrome and its pathogenicity in turkey poults. Avian Diseases, 46, 32–47. 10.1637/0005-2086(2002)046[0032:IOARFP]2.0.CO;2
- Hieronymus, D.R.K., Villegas, P. & Kleven, S.H. (1983). Identification and serological differentiation of several reovirus strains isolated from chickens with suspected malabsorption syndrome. Avian Diseases, 27, 246–254. 10.2307/1590390
- Jindal, N., Patnayak, D.P., Chander, Y., Ziegler, A.F., & Goyal, S.M. (2010a). Detection and molecular characterization of enteric viruses from poult enteritis syndrome in turkeys. Poultry Science, 89, 217–226. 10.3382/ps.2009-00424
- Jindal, N., Patnayak, D.P., Chander, Y., Ziegler, A.F. & Goyal, S.M. (2010b). Detection and molecular characterization of enteric viruses in breeder turkeys. Avian Pathology, 39, 53–61. 10.1080/03079450903490289
- Kapczynski, D.R., Sellers, H.S., Simmons, V. & Schultz-Cherry, S. (2002). Sequence analysis of the S3 gene from a turkey reovirus. Virus Genes, 25, 95–100. 10.1023/A:1020130410601
- King, A.M.Q., Lefkowiz, E., Adams, M. & Carstens, E. (2012). Virus Taxonomy: Ninth Report of the International Committee on Taxonomy of Viruses. Reoviridae. Waltham: Academic Press. pp. 541–637.
- Kort, Y.H., Bourogaa, H., Gribaa, L., Scott-Algara, D. & Ghram, A. (2013). Molecular characterization of avian reovirus isolates in Tunisia. Virology Journal, 10, 12. http://www.virologyj.com/content/10/1/12.
- Le Gall-Recule, G., Cherbonnel, M., Arnauld, C., Blanchard, P., Jestin, A. & Jestin, V. (1999). Molecular characterization and expression of the S3 gene of Muscovy duck reovirus strain 89026. Journal of General Virology, 80, 195–203.
- Liu, H.J., Lee, L.H., Hsu, H.W., Kuo, L.C. & Liao, M.H. (2003). Molecular evolution of avian reovirus: evidence for genetic diversity and reassortment of the S-class genome segments and multiple cocirculating lineages. Virology, 314, 336–349. 10.1016/S0042-6822(03)00415-X
- Mabrouk, T. & Lemay, G. (1994). Mutations in a CHCC zinc-binding motif of the reoviruses σ3 protein decrease its intracellular stability. Journal of Virology, 68, 5287–5290.
- Martinez-Costas, J., Grande, A., Varela, R., Garcia-Martinez, C. & Benavente, J. (1997). Protein architecture of avian reovirus S1133 and identification of the cell attachment protein. Journal of Virology, 71, 59–64.
- Miller, J.E. & Samuel, C.E. (1992). Proteolytic cleavage of the reovirus sigma 3 protein results in enhanced double-stranded RNA-binding activity: identification of a repeated basic amino acid motif within the C-terminal binding region. Journal of Virology, 66, 5347–5356.
- Mor, S.K., Sharafeldin, T.A., Abin, M., Kromm, M., Porter, R.E., Goyal, S.M. & Patnayak, D.P. (2013a). The occurrence of enteric viruses in light turkey syndrome. Avian Pathology, 42, 497–501. 10.1080/03079457.2013.832145
- Mor, S.K., Sharafeldin, T.A., Porter, R.E., Ziegler, A., Patnayak, D.P. & Goyal, S.M. (2013b). Isolation and characterization of a turkey arthritis reovirus. Avian Diseases, 57, 97–103. 10.1637/10353-090712-Reg.1
- Moura-Alvarez, J., Chacon, J.V., Scanavini, L.S., Nunez, L.F.N., Astolfi-Ferreira, C.S., Jones, R.C. & Piantino Ferreira, A.J. (2013). Enteric viruses in Brazilian turkey flocks: single and multiple virus infection frequency according to age and clinical signs of intestinal disease. Poultry Science, 92, 945–955. 10.3382/ps.2012-02849
- Nibert, M.L. & Schiff, L.A. (2001). Reoviruses and their replication. In D.M. Knipe & P.M. Hooley (Eds.), Fields Virology 4th edn (pp. 1679–1728). Philadelphia, PA: Lippincott Willams & Wilkins.
- Pantin-Jackwood, M.J., Day, J.M., Jackwood, M.W. & Spackman, E. (2008). Enteric viruses detected by molecular methods in commercial chicken and turkey flocks in the United States between 2005 and 2006. Avian Diseases, 52, 235–244. 10.1637/8174-111507-Reg.1
- Rosenberger, J.K. (2003). Reovirus infections. In Y.M. Saif, H.J. Barnes, J.R. Glisson, A.M. Fadly, L.R. McDougald & D.E. Swayne (Eds.), Diseases of Poultry 11th edn (pp. 283–298). Ames, IA: Iowa State University Press.
- Rosenberger, J.K., Sterner, F.J., Botts, S., Lee, K.P. & Margolin, A. (1989). In vitro and in vivo characterization of avian reoviruses. I. Pathogenicity and antigenic relatedness of several avian reovirus isolates. Avian Diseases, 33, 535–544. 10.2307/1591118
- Schiff, L.A., Nibert, M.L., Co, M.S., Brown, E.G. & Fields, B.N. (1988). Distinct binding sites for zinc and double-stranded RNA in the reovirus outer capsid protein σ3. Molecular and Cellular Biology, 8, 273–283.
- Schnitzer, T.J. (1985). Protein coding assignment of the S genes of the avian reovirus S1133. Virology, 141, 167–170. 10.1016/0042-6822(85)90194-1
- Sellers, H.S., Linnemann, E.G., Pereira, L. & Kapczynski, D.R. (2004). Phylogenetic analysis of the sigma 2 protein gene of turkey reoviruses. Avian Diseases, 48, 651–657. 10.1637/7181-032304R
- Spackman, E., Pantin-Jackwood, M., Day, J. & Sellers, H. (2005). The pathogenesis of turkey origin reoviruses in turkeys and chickens. Avian Pathology, 34, 291–296. 10.1080/03079450500178501
- Spandidos, D.A. & Graham, A.F., (1976). Physical and chemical characterization of an avian reovirus. Journal of Virology, 19, 968–976.
- Touris-Otero, F., Martinez-Costas, J., Vakharia, V.N. & Benavente, J. (2005). Characterization of the nucleic acid-binding activity of the avian reovirus non-structural protein σNS. Journal of General Virology, 86, 1159–1169. 10.1099/vir.0.80491-0
- Varela, R. & Benavente, J. (1994). Protein coding assignment of avian reovirus strain S1133. Journal of Virology, 68, 6775–6777.
- Varela, R., Martinez-Costas, J., Mallo, M. & Benavente, J. (1996). Intracellular post translational modifications of S1133 avian reovirus proteins. Journal of Virology, 70, 2974–2981.
- Wickramasinghe, R., Meanger, J., Enriquez, C.E. & Wilcox, G.E. (1993). Avian reovirus proteins associated with neutralization of virus infectivity. Virology, 194, 688–696. 10.1006/viro.1993.1309
- Yin, H.S. & Lee, L.H. (1998). Identification and characterization of RNA-binding activities of avian reovirus non-structural protein σNS. Journal of General Virology, 79, 1411–1413.