Abstract
Rotaviruses infect humans and animals and are classified into eight groups (A to H). Group D rotavirus (RVD) has been described in birds, although relatively few reports are available. The present study focused on RVD, including epidemiological and molecular aspects of samples collected from broiler chickens in the state of Pará, Brazil. A total of 85 faecal samples were collected between 2008 and 2011 from 37 chicken farms located in eight different municipalities. The viral double-stranded RNA was extracted from faecal suspensions and analysed using polyacrylamide gel electrophoresis (PAGE), followed by reverse transcriptase-polymerase chain reaction (RT-PCR) and nucleotide sequencing of the VP6 and VP7 genes. Comparing the positive results, 16.5% (14/85) were obtained by PAGE and 35.3% (30/85) by RT-PCR. Samples from seven of eight municipalities were positive for RVD and infections were recorded in 17 (45.9%) of 37 chicken farms. The RVD infection rate was significantly higher in the 16-day to 30-day age group (62.2%; 23/37) compared with other ages. No consistent relationship was found between the infection rate and either the population density in poultry houses or the climatic conditions. The nucleotide sequences of the VP6 gene were 89.9 to 90.9% similar to the prototype strain 05V0049 and were 88.3 to 100% similar among themselves; VP7 gene nucleotide sequences were 84.3 to 85.4% similar to the prototype strain 05V0049 and 93.8 to 100% similar among themselves. Overall, this study provides new insights into the epidemiology and genome characterization of group D rotaviruses.
Introduction
Gastroenteritis caused by rotaviruses (RVs) affects both animals and humans. RVs are among the most common viruses found in avian species and have been detected in ducks, pheasants, chickens, pigeons, turkeys, as well as in wild bird species (McNulty et al., Citation1978; Takase et al., Citation1986; Gough et al., Citation1992; Legrottaglie et al., Citation1997; Otto et al., Citation2011; Ursu et al., Citation2011). RVs are classified into eight groups named A to H (Matthijnssens et al., Citation2011). Birds are infected by group A avian rotaviruses, which are found in both humans and animals, and by groups D, F and G that are found only in avian species (Kindler et al., Citation2013).
RVs are non-enveloped icosahedral particles with a genome of 11 segments of double-stranded RNA. Segments 1, 2, 3, 4, 6 and 9 code for structural proteins VP1, VP2, VP3, VP4, VP6 and VP7 respectively, and segments 5, 7, 8 and 10 for the non-structural proteins NSP1, NSP3, NSP2 and NSP4 respectively. NSP5 and NSP6 are coded for by segment 11 (Estes & Greenberg, Citation2013).
The VP6 protein, which is located in the intermediate layer, is hydrophobic, has antigenic and immunogenic potential, and is highly stable. VP6 also has conserved epitopes that are excellent targets for laboratory diagnosis, allowing species or group determination (Estes & Greenberg, Citation2013). The principal component of the external capsid is VP7 (Mattion et al., Citation1994), which plays an important role in the induction of neutralizing antibodies and is responsible for defining G serotype specificity (Estes & Greenberg, Citation2013).
RV infection in birds may be symptomatic or asymptomatic (Tamehiro et al., Citation2003; Villarreal et al., Citation2008; Bezerra et al., Citation2012). When clinical signs are present, they relate to enteritis, diarrhoea, dehydration, weakness and anorexia, which lead to weight loss and mortality that are translated into unfavourable economic outcomes (Page et al., Citation1982; Otto et al., Citation2006; Spackman et al., Citation2010).
Group D rotavirus (RVD) has been studied by Trojnar et al. (Citation2010), and more recently the VP6 gene was analysed by Otto et al. (Citation2011) and Bezerra et al. (Citation2012). Despite these recent advances there is still much to be learnt about RVD, which infects a number of different bird species. For this reason, the present study focused on the detection of RVD in broiler chickens from the state of Pará, North Brazil with regard to epidemiological and molecular aspects of RVD infection.
Materials and Methods
Samples
Samples were collected between 2008 and 2011 from 37 chicken farms. An average of one to four pooled samples was obtained from each farm, located in eight different municipalities in the state of Pará. A total of 85 faecal specimens, corresponding to 85 pooled samples, were obtained from broiler chickens without evident clinical signs of illness. Of these, 30 samples were described previously by Bezerra et al. (Citation2012). In the process of gathering samples, we made pools using samples from nine points in the same poultry house.
RNA polyacrylamide gel electrophoresis
All 85 samples were diluted 2:5 (v/v) in phosphate-buffered saline (pH 7.4). These suspensions were centrifuged at 700 × g for 20 min and the viral RNA was extracted from 600 ml supernatant using guanidine isothiocyanate/silica powder glass as described by Boom et al. (Citation1990). The polyacrylamide gel electrophoresis (PAGE) was performed using the technique described by Pereira et al. (Citation1983) for RVD detection. This method was used since it is able to identify the typical migration pattern, which shows a 5:2:2:2 distribution of electrophoretic segments. Although very specific, PAGE has lower sensitivity compared with reverse transcriptase-polymerase chain reaction (RT-PCR) (Bezerra et al., Citation2012). Thus, all samples were subsequently subjected to RT-PCR.
Reverse transcriptase-polymerase chain reaction
The primers used for amplification of the VP6 gene (RD6F and RD6R) were described by Bezerra et al. (Citation2012). New primers were developed at the Evandro Chagas Institute for amplification of the VP7 gene based on the RVD genome (Trojnar et al., Citation2010), using the PRIMER 3 program available on the National Center for Biotechnology Information website (http://www.ncbi.nlm.nih.gov/tools/primer-blast/). The forward primer is RD9F (5′-ACCATATAGGAGTGACGCACCT-3′) and the reverse primer is RD9R (5′-AGCCCACCACTTCTTCCAAT-3′). These primers amplify a fragment of 879 base pairs (bp). Briefly, the RT-PCR procedure was performed using the same conditions as for the VP6 gene described previously by Bezerra et al. (Citation2012). All samples were subjected to RT-PCR for amplification of the VP6 and VP7 genes and the positivity rate was determined based on the detection of both genes.
Sequencing
Eighteen positive samples were randomly selected for sequencing of the VP6 and VP7 genes. Nucleotide sequencing using both forward and reverse primers was performed using primers for VP6 (RD6F and RD6R) and for VP7 (RD9F and RD9R). The sequencing was preceded by purification with the QIAquick®PCR purification kit (Qiagen®, Valencia, CA, USA), according to the manufacturer's instructions. The sequences were determined using the Big Dye Terminator (Applied Biosystems, Carlsbad, CA, USA) kit and an ABI PRISM 3100 automatic sequencer (Applied Biosystems). The sequences obtained for the VP6 and VP7 genes were aligned and edited by BioEdit: Biological sequence alignment editor (v.7.0.5.3, Carlsbad, CA, USA). Dendrograms were produced in Molecular Evolutionary Genetics Analysis (MEGA v.5.05; Tamura et al., Citation2011) using the neighbour-joining method based on the parameters of Kimura (Citation1980) and a non-parametric bootstrap reliability test with 2000 replicates. Nucleotide sequences of VP6 and VP7 genes were compared with the RVD prototype 05V0049 (accession numbers VP6-GU733448 and VP7-GU733451) and other rotavirus prototypes that infect avian species: RVA Ch-02V0002G3 (accession numbers VP6-FJ169858 and VP7-EU486971), RVF 03V0568 (accession numbers VP6-HQ403603 and VP7-NC021627), and RVG 03V0567 (accession numbers VP6-HQ403604 and VP7-NC021582).
Statistical and epidemiological analyses
Data were analysed in Microsoft Excel 2012 and BioEstat 5.0 (Ayres et al., Citation2007). Significant differences in infection rates across age groups (1 to 15 days, 16 to 30 days and 31 to 45 days) were assessed using a one-sample binomial test and chi-square.
The possible effects of stocking density for chickens in the poultry house on infection rates was assessed by comparing densities of ≤9 birds/m2 with those of >9 birds/m2 (Manno et al., Citation2011), as analysed by the G test (Ayres et al., Citation2007). It was not possible to determine the density of the birds from which 13 of the samples were obtained. Potential effects of climatic variables were also evaluated and, because the Amazon region does not have well-defined seasons, samples were grouped into rainiest season (December to May) and less rainy season (June to November) (Lima et al., Citation2009). Differences between seasons relating to RVD prevalence were obtained using the chi-square test. In all analyses, the α≤ 0.05 significance level was considered.
Results
Detection of group D rotavirus
Fourteen of 85 samples (16.5%) analysed by PAGE presented an electrophoretic profile (5:2:2:2) consistent with that of RVD (). The RT-PCR amplified the VP6 and VP7 genes producing fragments of 742 bp and 879 bp respectively. RT-PCR of 16 samples that were negative by PAGE showed positive results, with a positivity rate of 35.3% (30/85). Thirty samples were positive for both VP6 and VP7 genes. Seven of eight sampled municipalities presented positive results for RVD, and the virus was found in almost one-half (17; 45.9%) of the 37 farms visited.
Group D rotavirus-positivity by age group, population density, and season
The highest frequency of RVD was detected in the 16 to 30 days age group (62.2%; 23/37), representing 76.7% (23/30) of all positive samples (P = 0.0003). Based on the binomial test (), this frequency (16 to 30 days class) was significantly higher than that for the 0 to 15 days (P = 0.035) and 31 to 45 days (P = 0.008) classes. Overall, the differences among age classes were significant (χ² = 26.6, degrees of freedom = 2, P < 0.0001).
Table 1. Detections of RVD using RT-PCR based on the age group of chickens raised on farms in the state of Pará, Brazil.
A total of 38.5% positive RVD samples (20/52) were detected in the high-density group (>9 birds/m2) and 25% (5/20) in the low-density group (≤9 birds/m2). However, the difference in infection rates, verified among these two groups, was not significant (G = 0.6529, P = 0.4191) (). The RVD positivity observed during the least rainy season was 34% (16/47), and in the rainiest season was 36.8% (14/38) (). No evidence of significant climatic effect was observed comparing the seasons and infection rates (χ² = 0.072, P = 0.9679).
Analysis of the VP6 and VP7 genes
The VP6 and VP7 gene sequences from 18 samples were analysed and classified as belonging to RVD ( and respectively). Regarding the VP6 gene, a fragment of 742 bp was analysed, corresponding to nucleotides 3 to 744 of the coding region of the gene (Trojnar et al., Citation2010). These nucleotide sequences were 89.9 to 90.9% similar to the prototype strain 05V0049 for RVD (accession number: GU733448) (Trojnar et al., Citation2010) and were 88.3 to 100% similar among themselves. Analysis of the amino acid sequences of the VP6 gene revealed a high degree of similarity (99.2 to 100.0%) between the positive samples of this study and the prototype strain 05V0049. Relating to other groups of rotavirus, a nucleotide similarity of 51.8 to 54.2% was observed for RVA (Ch-02V0002G3), 53 to 54.1% for RVF (03V0568) and 43 to 43.5% for RVG (03V0567). One amino acid substitution was detected in residue 12 when compared with the RVD strain 05V0049; 10 of the 18 samples contained lysine instead of arginine. The VP6 sequences analysed in the present study are available in Genbank (accession numbers: JN703463, JQ065734 to JQ065734, KC623164 to KC623166 and KJ101579 to KJ101589).
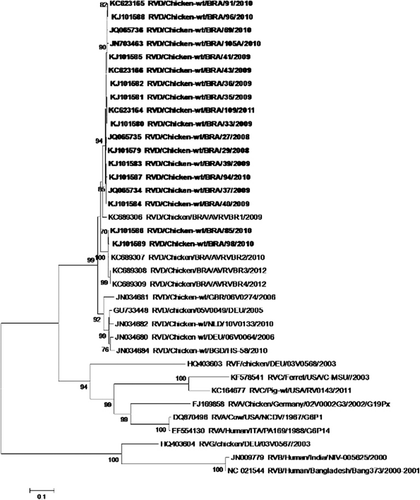
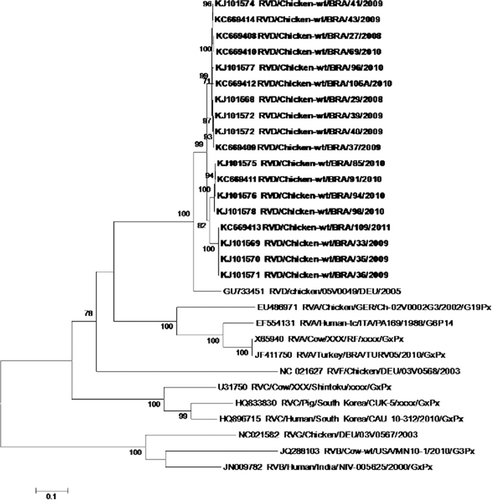
For the VP7 gene, the analysis resulted in a fragment of 841 bp, which corresponds to nucleotides 1 to 841 of the gene's coding region, using the prototype RVD (05V0049) as a reference. The nucleotide sequences were 84.3 to 85.4% similar to this prototype and were 93.8 to 100.0% similar among themselves. Compared with the prototypes of other RV groups, the VP7 sequences were generally less similar than those of the VP6 gene: for RVA, 52.1 to 53.1% (Ch-02V0002G3); for RVF, 46.1 to 47.2% (03V0568); and for RVG, 36.6 to 37.6% (03V0567). Analysis of the amino acid sequences of the VP7 gene revealed a similarity between the positive samples in this study and the prototype of 88.6 to 90.1%, and 96.4 to 100% among themselves. The divergence of VP7 amino acid sequence in comparison with the prototype 05V0049 is shown in . The VP7 sequences analysed here are available on Genbank (accession numbers KC669408 to KC669414 and KJ101568 to KJ101578).
Discussion
The occurrence of RVD has been reported in a number of bird species, particularly chickens and turkeys, giving importance to this viral group in terms of economic and commercial impact (Tamehiro et al., Citation2003; Otto et al., Citation2006; Karim et al., Citation2007; Savita et al., Citation2008; Islam et al., Citation2009). Detection of RVD has been based essentially on PAGE, owing to its typical electrophoretic profile. However, PAGE has a low sensitivity when compared with RT-PCR (Bezerra et al., Citation2012).
The present study included the use of PAGE and RT-PCR for detecting RVD in chickens and confirmed the lower sensitivity of PAGE, as demonstrated by a positivity rate of 16.5%, as compared with 35.3% using RT-PCR. Studies conducted in different countries using PAGE to record the frequency of RVD in birds obtained the following results: Bangladesh, 13.2 to 19.3% (Karim et al., Citation2007; Islam et al., Citation2009); India, 15.2% (Savita et al., Citation2008); and Germany, 35.3% (Otto et al., Citation2006). In addition, in different European countries RVD was detected by PAGE in 35.5% of chickens and in 57.6% of turkeys (Otto et al., Citation2011).
In the present study, 35.3% of the samples were RVD-positive using RT-PCR, in contrast to 65.9% among European chickens and turkeys using real-time RT-PCR (Otto et al., Citation2011). This difference may be related to clinical signs, since all birds in the European study had clinical signs, whereas in our study the chickens were apparently free of signs. In our study other viruses, such as picobirnavirus, were detected by PAGE in 15.3% (13/85) of chickens and in 49.4% (42/85) when using RT-PCR (Silva et al., Citation2014), and avian rotaviruses were detected by RT-PCR in 41.2% of chickens (35/85) (Silva et al., Citation2013).
While our study identified a significantly higher prevalence of RVD infection among chickens 16 to 30 days of age (P = 0.0003), compared with other age groups, Silva et al. (Citation2013) reported a higher positivity among chickens aged 31 to 45 days (48.6%). Unlike these studies, Tamehiro et al. (Citation2003) demonstrated that RV was an important aetiological agent of enteric disorders, especially in broiler chickens younger than 30 days of age. Furthermore, other studies found that chicks up to 15 days of age were the most affected age group (Pantin-Jackwood et al., Citation2008; Jindal et al., Citation2010). In the specific case of RVD, the few studies available suggest a higher susceptibility in broiler chickens 7 to 12 days of age, as compared with other ages (Karim et al., Citation2007; Islam et al., Citation2009).
A trend for more frequent infection was observed in the houses with a higher density of chickens, even though the difference was not significant. Manno et al. (Citation2011) have shown that high animal densities may reflect poor hygiene conditions, which facilitates the dispersion of the virus, as well as increasing stress levels.
No typical seasonal pattern was observed when analysing infection rates over time. Barrios et al. (Citation1991) recorded a similar pattern in a study of RVA in birds. This may be related to the lack of any major seasonal variation in temperatures or humidity in the Amazon region.
Further studies involving both symptomatic and asymptomatic chickens are needed to better assess the role of different factors related to bird susceptibility to RVD infection. These factors may include age, population density and climatic parameters as suggested in the present study. Comparing the amino acid sequence of the VP6 gene with that of the German prototype (05V0049), there were only slight, minor differences. In contrast, with regard to the VP7 gene, the amino acid sequence of our samples was more divergent when compared with sample 05V0049, considering that a number of amino acid substitutions were observed in some samples. Further analyses are needed to determine whether these modifications of amino acid sequence have altered the conformational structure of the proteins.
The general lack of data on the nucleotide sequences for RVD precludes a more detailed discussion regarding similarities and differences between RVD strains. In this regard, further molecular epidemiology studies would be worthwhile, in an attempt to broaden our understanding of the true economic impact of RVD infection among broiler chickens.
In conclusion, the present study reports the occurrence of RVD in asymptomatic chickens at a frequency of 35.3% in poultry houses and 45.9% in farms, with a significantly higher rate in birds 16 to 30 days of age. Neither population density nor climatic conditions appeared to have any significant effect on infection rates. The amino acid sequence of the VP6 gene was well conserved in comparison with the prototype, whereas the VP7 gene showed lower similarity, presenting a number of unique variants. These new data provide additional important insights into the epidemiology of RVD, as well as broadening our knowledge of the RVD genome in Brazil and other regions of the world.
Acknowledgements
Delana A. M. Bezerra and Jane H. L. Kaiano received a grant fellowship from the Brazilian National Council for Development of Science and Technology (CNPq). The authors gratefully acknowledge the valuable statistical analysis provided by Dr Jones Siqueira and Dr Fernanda Sagica.
Funding
This work was partially supported by a grant from Pará State Secretary of Science and Technology, contract number 067/2008 (SEDECT/FAPESPA/PA).
Additional information
Funding
References
- Ayres, M., Ayres, J.R., Ma, D.L. & Santos, A.S. (2007). BioEstat 5.0: Aplicações estatísticas nas áreas das ciências biológicas e médicas [BioEstat 5.0: Statistical applications in the areas of biological and medical sciences] 5th edn. Belém: Instituto de Desenvolvimento Sustentável Mamirauá – IDSM/MCT/CNPq. (p. 364).
- Barrios, H., Viora, S., Franceschi, M. & Fliess, E. (1991). Incidencia de los rotavirus en establecimientos avícolas de producción intensiva [Incidence of rotavirus in intensive production poultry farms]. Revista Argentina de Microbioligia, 23, 5–21.
- Bezerra, D.A.M., Silva, R.R., Kaiano, J.H.L., Silvestre, R.V.D., Oliveira, D.S., Linhares, A.C., Gabbay, Y.B. & Mascarenhas, J.D.P. (2012). Detection of avian group D rotavirus using the polymerase chain reaction for the VP6 gene. Journal of Virological Methods, 185, 189–192. 10.1016/j.jviromet.2012.07.017
- Boom, R., Sol, C.J., Salimans, M.M., Jansen, C.L., Wertheim-Van dillen, P.M. & Van Der Noordaa, J. (1990). Rapid and simple method for purification of nucleic acids. Journal of Clinical Microbiology, 28, 495–503.
- Estes, M.K. & Greenberg, H.B. (2013). Rotaviruses. In D.M. Knipe & P.M. Howley (Eds). Fields’ Virology. 6th edn (pp. 1347–1401). Philadelphia, PA: Lippincott, Williams and Wilkins.
- Gough, R.E., Cox, W.J. & Devoy, J. (1992). Isolation and identification of rotavirus from racing pigeons. The Veterinary Record, 130, 273. 10.1136/vr.130.13.273
- Islam, M.S., Alam, M.M., Ahmed, M.U., Saifuzzaman, A.B.M., KobayashI, N., Kayesh, M.E.H. & Udin, M.S. (2009). Molecular epidemiologic study on rotavirus infection in human and birds in association with gastroenteritis. Bangladesh Journal of Veterinary Medicine, 7, 233–237.
- Jindal, N., Patnayak, D.P., Chander, Y., Ziegler, A.F. & Goyal, S.M. (2010). Detection and molecular characterization of enteric viruses in breeder turkeys. Avian Pathology, 39, 53–61. 10.1080/03079450903490289
- Karim, M.R., Rume, F.I., Alam, M.M. & Ahmed, M.U. (2007). Molecular epidemiologic study on avian rotavirus prevailing in Bangladesh. Bangladesh Journal of Veterinary Medicine, 5, 43–48.
- Kimura, M. (1980). A simple method for estimating evolutionary rate of base substitution through comparative studies of nucleotide sequences. Journal of Molecular Evolution, 16, 111–120. 10.1007/BF01731581
- Kindler, E., Trojnar, E., Heckel, G., Otto, P.H. & Johne, R. (2013). Analysis of rotavirus species diversity and evolution including the newly determined full-length genome sequences of rotavirus F and G. Infection, Genetics and Evolution, 14, 58–67.
- Legrottaglie, R., Rizzi, V. & Agrimi, P. (1997). Isolation and identification of avian rotavirus from pheasant chicks with signs of clinical enteritis. Comparative Immunology, Microbiology & Infectious Diseases, 20, 205–210. 10.1016/S0147-9571(97)00005-2
- Lima, K.R.S., Alves, J.A.K., Araújo, C.V., Manno, M.C., Jesus, M.L.C., Fernandes, D.L. & Tavares, F. (2009). Inner thermal environment assessment in broiler house with different roofs materials on the great metropolitan region of Belém. Revista Ciências Agrárias, 51, 37–50.
- Manno, M.C., Lima, K.R.S., Aguilar, C.A.L., Souza, N.S.S., Barata, Z.R.P. & Viana, M.A.O. (2011). Production of ammonia inside poultry houses with environmental changes. Amazonian Journal of Agricultural and Environmental Sciences, 54, 153–158.
- Matthijnssens, J., Ciarlet, M., McDonald, S.M., Attoui, H., Bányai, K., Brister, J.R., Buesa, J., Esona, M.D., Estes, M.K., Gentsch, J.R., Iturriza-Gómara, M., Johne, R., Kirkwood, C.D., Martella, V., Mertens, P.P., Nakagomi, O., Parreño, V., Rahman, M., Ruggeri, F.M., Saif, L.J., Santos, N., Steyer, A., Taniguchi, K., Patton, J.T., Desselberger, U. & Van, Ranst, M. (2011). Uniformity of rotavirus strain nomenclature proposed by the Rotavirus Classification Working Group (RCWG). Archives of Virology, 156, 1397–1413. 10.1007/s00705-011-1006-z
- Mattion, N., Cohen, J. & Estes, M. (1994). The rotavirus proteins. In A.Z. Kapikian (Ed.). Viral Infections of Gastrointestinal Tract (pp. 169–249). New York: Marcel Dekker.
- Mcnulty, M.S., Allan, G.M. & Stuart, J.C. (1978). Rotavirus infection in avian species. The Veterinary Record, 103, 319–320. 10.1136/vr.103.14.319-a
- Otto, P.H., Ahmed, M.U., Hotzel, H., Machnowska, P., Reetz, J, Roth, B., Trojnar, E. & Johne, R. (2011). Detection of avian rotaviruses of groups A, D, F and G in diseased chickens and turkeys from Europe and Bangladesh. Veterinary Microbiology, 156, 8–15. 10.1016/j.vetmic.2011.10.001
- Otto, P., Liebler-Tenorio, E.M., Elschner, M., Reetz, J., Lohren, U. & Dille, R. (2006). Detection of rotaviruses and intestinal lesions in broiler chicks from flocks with runting and stunting syndrome (RSS). Avian Diseases, 50, 411–418. 10.1637/7511-020106R.1
- Page, R.K, Fletcher, O.J, Rowland, G.N., Gaudry, D. & Villegas, P. (1982). Malabsorption syndrome in broiler chickens. Avian Diseases, 26, 618–624. 10.2307/1589910
- Pantin-Jackwood, M.J., Day, J.M., Jackwood, M.W. & Spackman, E. (2008). Enteric viruses detected by molecular methods in commercial chicken and turkey flocks in the United States between 2005 and 2006. Avian Diseases, 52, 235–244. 10.1637/8174-111507-Reg.1
- Pereira, H.G., Azeredo, R.S., Leite, J.P., Barth, O.M., Sutmoller, F., De Farias, V. & Vidal, M.N. (1983). Comparison of polyacrilamide gel electrophoresis (PAGE), immuno-electron microscopy (IEM) and enzyme immunoassay (EIA) for the rapid diagnosis of rotavirus infection in children. MemóriaInstituto Oswaldo Cruz, 78, 483–490.
- Savita, A.L.K., Malik, Y.P.S. & Minakshi, G.P. (2008). Detection and characterization of group A and D avian rotaviruses in India. Indian Journal of Biotechnology, 7, 554–556.
- Silva, R.R., Bezerra, D.A.M., Kaiano, J.H.L., Manno, M.C., Oliveira, D.S., Sagica, F.E.S., Gabbay, Y.B., Chaves, S.O.C., da Silva, A.L.C., Alfieri, A, A. & Mascarenhas, J.D.P. (2013). Molecular epidemiology of avian rotavirus in fecal samples of broiler chickens in Amazon Region, Brazil, from August 2008 to May 2011. Revista Pan-Amazônica de Saúde, 4, 55–62. 10.5123/S2176-62232013000200007
- Silva, R.R., Bezerra, D.A.M., Kaiano, J.H.L., Manno, M.C., Oliveira, D.S., Silvestre, R.V.D., Gabbay, Y.B., Ganesh, B. & Mascarenhas, J.D.P. (2014). Genogroup I avian picobirnavirus detected in Brazilian broiler chickens: a molecular epidemiology study. Journal of General Virology, 95, 117–122 10.1099/vir.0.054783-0
- Spackman, E., Day, J.M. & Pantin-Jackwood, M.J. (2010). Astrovirus, reovirus, and rotavirus concomitant infection causes decreased weight gain in broad-breasted white poults. Avian Diseases, 54, 16–21. 10.1637/8986-070909-Reg.1
- Takase, K., Nonaka, F., Sakaguchi, M. & Yamada, S. (1986). Cytopathic avian rotavirus isolated from duck faeces in chicken kidney cell cultures. Avian Pathology, 15, 719–730. 10.1080/03079458608436334
- Tamehiro, C.Y., Alfieri, A.F., Medici, C. & Alfieri, A. A. (2003). Segmented double-stranded genomic RNA viruses in fecal samples from broiler chicken. Brazilian Journal of Microbiology, 34, 344–348. 10.1590/S1517-83822003000400013
- Tamura K, Peterson D, Peterson N, Stecher G, Nei M, and Kumar S (2011). MEGA5: Molecular Evolutionary Genetics Analysis using Maximum Likelihood, Evolutionary Distance, and Maximum Parsimony Methods. Molecular Biology and Evolution 28, 2731–2739.
- Trojnar, E., Otto, P., Roth, B., Reetz, J. & Johne, E. (2010). The genome segments of a group D rotavirus possess group A-like conserved termini but encode group-specific proteins. Journal of Virology, 84, 10254–10265. 10.1128/JVI.00332-10
- Ursu, K., Papp, H., Kisfali, P., Rigó, D., Melegh, B., Martella, V. & Bányai, K. (2011). Monitoring of group A rotaviruses in wild-living birds in Hungary. Avian Diseases, 55, 123–127. 10.1637/9428-061610-RESNOTE.1
- Villarreal, L.Y.B., Uliana, G., Valenzuela, C., Chacón, J.LV., Saidenberg, A.B.S., Sanches, A.A., Brandão, P.E., Jerez, J.A. & Ferreira, A.J.P. (2008). Rotavirus detection and isolation from chickens with or without symptoms. Brazilian Journal of Poultry Science, 8, 187–191.