Abstract
Escherichia coli colonies isolated from the bone marrow of fresh dead hens of laying flocks with the E. coli peritonitis syndrome (EPS) were genotyped using pulsed-field gel electrophoresis (PFGE). Typing is important from an epidemiological point of view and also if the use of autogenous (auto)vaccines is considered. Birds with EPS originated from one house of each of three layer farms and one broiler breeder farm. Farms were considered as separate epidemiological units. In total, six flocks were examined including two successive flocks of one layer farm and the broiler breeder farm. E. coli colonies (one per bird) from nine to 16 hens of each flock were genotyped. The clonality of E. coli within birds was studied using five colonies of each of nine to 14 birds per flock. E. coli genotypes, which totalled 15, differed between farms and flocks except for two successive layer flocks that shared three genotypes. One to five genotypes were found per flock with one or two genotypes dominating each outbreak. Within hens, E. coli bacteria were always clonal. Colonies of the same PFGE type always had the same multilocus sequence type. However, four PFGE types shared sequence type 95. Neither PFGE types nor multilocus sequence types were unambiguously related to avian pathogenic E. coli from EPS. In cases where persistence of E. coli strains associated with EPS is found to occur frequently, routine genotyping to select strains for autovaccines should be considered.
Introduction
Colibacillosis is a disease that occurs frequently in commercial poultry worldwide and has a significant economic impact (Gross & Siegel, Citation1959; Bisgaard & Dam, Citation1981; Barnes et al., Citation2008). The disease is induced by avian pathogenic Escherichia coli (APEC) and is considered the main cause of mortality in productive hens and in broilers (Yogaratnam, Citation1995; Dhillon & Jack, Citation1996; Jordan et al., Citation2005; Landman & Cornelissen, Citation2006; Barnes et al., Citation2008; Stokholm et al., Citation2010).
Several forms of the disease exist, either localized or generalized. Localized forms are characterized by lesions generally affecting one organ; for example, coliform omphalitis, coliform cellulitis, swollen head syndrome, diarrheal disease, coliform salpingitis/peritonitis/salpingoperitonitis (SPS). Generalized forms occur when virulent bacteria are able to access the bloodstream (bacteraemia), which leads to colisepticaemia. The sequelae of colisepticaemia as well as coligranulomas are also considered generalized forms of the disease (Bisgaard & Dam, Citation1981; Landman & Cornelissen, Citation2006; Barnes et al., Citation2008).
In broilers, fibrinous polyserositis (fibrinous peritonitis, pericarditis and perihepatitis)—often accompanied by septicaemia and mostly occurring in the second half of the growing period—is the most common form of colibacillosis, while in laying hens predominantly SPS and E. coli peritonitis syndrome (EPS) are diagnosed. Coliform SPS (“egg peritonitis”), which has been observed since the beginning of poultry farming, is a chronic condition and forms a substantial proportion of the “normal” mortality in flocks of productive hens (Bisgaard & Dam, Citation1981; Jordan et al., Citation2005; Stokholm et al., Citation2010). In contrast, EPS is characterized by acute mortality, soaring up to 10% in combination with severe septicaemia and fibrinous polyserositis lesions, while salpingitis rarely occurs. A decrease in egg production and an increase in downgraded eggs of a few per cent may be present. Flock and house associations are often observed with recurrent outbreaks within the same flock and in successive flocks in the same house. The disease has been observed in the USA and many European countries including the Netherlands from the mid 1990s onwards (Dhillon & Jack, Citation1996; Zanella et al., Citation2000; Vandekerchove et al., Citation2004; Landman & Cornelissen, Citation2006; Raviv et al., Citation2007; Landman et al., Citation2013).
Although the pathogenesis of EPS is largely unknown, this condition was recently successfully induced in egg-producing commercial brown layers by exposure of the hens to E. coli (chicken/NL/Dev/SP01404Cou/05) isolated from the bone marrow of a dead brown layer with EPS, by both artificial (intravenous and intraperitoneal) and natural (intratracheal, aerosol and intravaginal) infection routes. Approximately one-half of the birds exposed to an aerosol of relatively low doses of the earlier mentioned E. coli strain (estimated inhaled dose 105.1 to 106.2 colony-forming units [CFU] per hen) developed EPS, suggesting that the disease in the field is of respiratory origin. However, the vaginal infection route cannot be discarded as also approximately one-half of the birds inoculated by this route showed EPS, but high doses (108.7 to 108.9 CFU per hen) were needed (Landman et al., Citation2013).
Knowledge of the genetic diversity of the E. coli strains involved in EPS outbreaks, which is important from an epidemiological point of view and also if the use of (auto)vaccines is considered, is scarce. Only Olsen and coworkers genotyped E. coli bacteria obtained from laying hens with EPS (Olsen et al., Citation2011b). In the present study, therefore, the genetic relatedness of E. coli colonies obtained from the bone marrow of birds with EPS was studied using pulsed-field gel electrophoresis (PFGE). Birds with EPS originated from one house of each of three layer farms and one broiler breeder farm. Farms were considered as separate epidemiological units. In total, six flocks were examined including two successive flocks of one layer farm and the broiler breeder farm. Clonality of E. coli colonies was investigated within birds, between birds of the same farm including birds in successive flocks and between birds of all farms. Furthermore, E. coli colonies of each PFGE genotype found in this study were also characterized by multilocus sequence typing (MLST). Additionally, E. coli colonies obtained from cases of EPS not included in this study were analysed using MLST.
Materials and Methods
Experimental design, farms and flocks
The four chicken farms with outbreaks of EPS included in the present study (Farms A, B, C and D) were situated in different geographic locations, belonged to different integrations and had different veterinarians, and were therefore considered as separate epidemiological units. Farms A, B and C were layer farms with different housing systems (cage, floor and free range, respectively), while on Farm D broiler breeders were kept in a floor system. Farm C was the only organic and only multiple-age farm (). In Farm B, EPS was observed in the second laying period after forced moulting at 70 weeks of age. Two successive flocks of Farms C and D showed EPS, while this did not occur in flocks of Farms A and B. At 54 weeks of age, 10,560 hens from Flock A1 were sold and removed. Although mortality in this flock slightly increased from 41 weeks of age onwards (during week 53, 114 of 50,283 hens died), it exploded immediately after removal of the sold hens (in week 55, 549 of 38,983 hens died).
Table 1. Mortality in flocks of laying chickens with EPS from which E. coli colonies were subjected to PFGE and MLST genotyping.
Samples of birds originated from one and the same house per farm and were taken once from Farms A and B, while two successive flocks with EPS of Farms C and D were each sampled once.
Flock sizes, the age periods with increased mortality and mortality data are presented in . The start of the period of increased mortality in hens ranged from 24 weeks of age (Farm D, Flock 1) to 80 weeks of age (Farm B, moulted flock). Increased mortality continued until the end of the production period, except for one flock in which elevated mortality was observed from 41 to 57 weeks of age (Farm A). Mortality exceeding the norm ranged from 2.7 to 16.4% in hens. In cockerels, the mortality including culling based on the number present at 20 weeks of age in Flocks 1 and 2 of Farm D during the production period was 50.7% and 55.6%, respectively. Only Flock D1 was medicated. Medication was as follows: doxycycline (Soludox 50%, Eurovet Animal Health B.V., Bladel, the Netherlands), enrofloxacin (Enrox oraal 10%, Eurovet Animal Health B.V.), colistin (Coliplus DIVASA – FARMAVIC, S.A., GURB – VIC, Barcelona, Spain), doxycycline (Soludox 50%) plus tylosin (Tylan WO, Elanco Animal Health, Houten, the Netherlands), and flumequin (Enterflume® kalf kip, Dopharma, Raamsdonksveer, the Netherlands) at the age of 22 (preventive treatment), 25, 27, 28, and 34 weeks, respectively. Medications were given in the drinking water and the manufacturer's recommendations were followed for dosages and treatment duration (4 to 7 days). E. coli bacteria isolated from bone marrow at the age of 33 weeks showed resistance against doxycycline, were intermediate sensitive to flumequin, were intermediate sensitive to sensitive to enrofloxacin and were sensitive to colistin. Tylosin was not tested. Flock D2 was vaccinated intramuscularly twice at 8 and 17 weeks of age with an aqueous suspension of formalin inactivated E. coli autogenous vaccine (autovaccine) (dose = 108 CFU per chicken in 0.5 ml) prepared by the practitioner using E. coli colonies isolated from the bone marrow of approximately 10 hens with EPS of Flock D1. Egg production based on hens present was not affected in five of six flocks with EPS. In one flock with EPS, a drop in egg production of approximately 3 to 15% from 32 weeks of age until the end of the production period was observed (Farm C, Flock 1).
Selected hens (cockerels were not sampled) consisted of both birds that died within 24 hours before sampling (n = 102) and birds alive without clinical signs of disease (n = 27). The latter birds were stunned using a mixture of carbon dioxide and oxygen and exsanguinated by incision of the jugular vein. The age of the hens at sampling and the number of selected dead birds per flock ranged from 28 to 82 weeks and from 12 to 25 birds, respectively (). The number of clinically healthy hens that were examined ranged from one to eight per flock.
Table 2. Results of gross post-mortem examination and bacteriology of dead hens originating from chicken flocks with EPS.
To study genetic diversity within and between flocks, E. coli colonies (one per bird) from nine to 16 birds of each flock were compared using PFGE (n = 67 hens; ); moreover, the clonality of E. coli within birds was studied using five colonies of each of these birds except three birds (Flock C1, two birds; and Flock D2, one bird) because less than five colonies were isolated from these birds (n = 64 birds and 320 colonies). One E. coli colony obtained from each of two hens with EPS gathered in the years 2005 and 2009 was also included. In total, 325 E. coli colonies were examined: 320 colonies to study clonality within birds, one colony from each of the three hens from which less than five colonies were obtained, and one colony from each of the two hens sampled in the years 2005 and 2009.
Table 3. Number and prevalence of E. coli PFGE genotypes in chicken flocks with EPS.
Fifteen PFGE types were found and MLST was performed on each PFGE type for which two E. coli colonies obtained from different hens were used. Only one E. coli colony was used for six of 15 PFGE types as they were only found in one bird. Thus, 24 colonies were examined with MLST. Additionally, one E. coli colony obtained from the bone marrow of a hen with EPS from each of 39 flocks not included in this study was also typed using MLST. Colonies were collected between and including the years 2001 to 2012.
Post-mortem examination and bacteriology
Gross post-mortem examination was performed on all sampled birds. Macroscopic lesions and ovarian activity (in production, disturbed [flaccid, corrugated and leaking yellow follicles] and inactive [no yellow follicles present]) were recorded.
Bacteriological analysis of the bone marrow (femur) of selected birds was performed. The transversal cut bone physis was sterilized with a hot scalpel blade, after which a bone marrow sample was collected with a wire. A sheep-blood agar plate (K004P090; Biotrading, Mijdrecht, the Netherlands) was then inoculated, subsequently incubated overnight at 37°C under 5 to 10% carbon dioxide and thereafter visually inspected for colony growth and colony uniformity (purity). Biochemical identification of colonies was performed using the indole and β-glucuronidase test. All cultures were assumed to be pure based on colony uniformity and biochemical identification.
Birds with peritonitis and birds from which E. coli was isolated from the bone marrow were considered to have EPS.
Pulsed-field gel electrophoresis procedure
A suspension of each E. coli colony (n = 325 colonies) was made in 4 ml cell suspension buffer (100 mM Tris [1083820500; VWR, Amsterdam, the Netherlands]–100 mM ethylenediamine tetraacetic acid [EDTA, 11202911000; VWR], pH 8.0) having a concentration of 5.6 ± 0.5 McFarland (≈1.5 × 109 to 1.8 × 109 CFU/ml). Thereafter, 20 µl proteinase K, 20 mg/ml (P2308; Sigma-Aldrich, St Louis, MO, USA) was added to 400 µl cell suspension and combined with an equal volume of 1% melted SeaKem Gold agarose (161–3101; Lonza, Rockland, ME, USA)/1% sodium dodecyl sulphate (8170341000; VWR) and after mixing pipetted into disposable plug moulds (170–3713; Bio-Rad Laboratories, Hercules, CA, USA). After solidification, each plug was transferred to a well of a six-well plate containing 5 ml cell lysis buffer (50 mM Tris [1083820500; VWR], pH 8; 50 mM EDTA [11202911000; VWR], pH 8; 1% Sarcosyl [L5125-500G; Sigma-Aldrich]) and 25 µl proteinase K, 20 mg/ml (P2308; Sigma-Aldrich) and then incubated for 1.5 to 2 h at 54°C. Subsequently, the cell lysis buffer was replaced, twice with 8 ml/well prewarmed (50°C) sterile demineralized water and four times with 8 ml prewarmed TE buffer (10 mM Tris/1 mM EDTA), pH 8. Between each step, the plugs were incubated for 10 to 15 min at 50°C while placed on an orbital shaker (type Reax 3, Heidolph Instruments GmbH & Co. KG, Schwabach, Germany) at 120 rpm. Finally, the plugs were placed in fresh TE buffer and stored at 4°C until restriction-endonuclease digestion.
Restriction-endonuclease digestion
Digestion of the genomic DNA of the E. coli colonies and the Salmonella Braenderup H2812 (ATCC BAA-664) marker was performed with the restriction enzyme Xanthomonas badrii I (XbaI, 10674273001; Roche Diagnostics, Mannheim, Germany). The enzyme was diluted in SuRE Cut buffer H 1× (11417991001; Roche) to a final concentration of 10 U/sample before use. The agarose plugs were trimmed to fit on the comb (see “Pulsed-field gel electrophoresis”), combined with 200 µl diluted enzyme mixture in a 96-well plate and incubated for 3 to 6 h at 37°C on an orbital shaker (type Reax 3) at 120 rpm. In every PFGE run, the S. Braenderup marker was included as the molecular size standard. The digestion of S. Braenderup with XbaI (10674273001; Roche) produces fragment sizes ranging from 20.5 to 1135 kB.
Pulsed-field gel electrophoresis
After restriction-endonuclease digestion the plate was placed in a refrigerator for 10 min.
A 1.2% PFGE agarose gel was made by adding 1.8 g agarose (Seakem Gold, 161-3101; Lonza) to 150 ml of 0.5× TBE (Tris-borate–EDTA, BE50843; Lonza, Verviers, Belgium). The plugs were placed on the 45-well comb (170–3645; Bio-Rad Laboratories) and were allowed to dry for 20 min at room temperature before the agarose was added. After complete solidification, the 45-well comb was removed from the gel and the wells were filled in with some additional agarose. The gel was placed in the electrophoresis chamber, which was filled with 2 l of 0.5× TBE. Electrophoresis was performed using the contour clamped homogeneous electric field CHEF-DRIII system (Bio-Rad Laboratories). Electrophoresis conditions were set on an initial pulse time of 2.2 sec, final pulse time 54.2 sec at a constant voltage of 200 V (6 V/cm2) and a run time of 20 h. The gels were stained for 0.5 h with Gelred 3× (41001; Biotium, Hayward, California, USA) at room temperature and then photographed using ultraviolet light transillumination (Geldoc; Bio-Rad Laboratories).
Analysis of pulsed-field gel electrophoresis data
The DNA fingerprints generated were processed using BioNumerics software (Applied Maths, Kortrijk, Belgium) with the percentage similarity of profiles being calculated using the Dice coefficient and UPGMA for the generation of the dendrograms (optimization 1%, tolerance 1.5%). Colonies were considered to belong to the same genotype if similarity was >95%. For interpretation of the data, the criteria of Tenover (Tenover et al., Citation1995) were also used. Based on the numbers of colonies typed, detection limits with 95% confidence of PFGE genotypes were calculated (Johnson et al., Citation2004).
Multilocus sequence typing
MLST was performed according to the University of Warwick MLST scheme as described by Wirth et al. (Citation2006), which is based on seven housekeeping genes (adk, fumC, gyrB, icd, mdh, purA and recA). Primers and polymerase chain reaction (PCR) protocols are available on the website (http://mlst.warwick.ac.uk/mlst/dbs/Ecoli/documents/primersColi_html). Target genes and primers are presented in . The PCR protocols were modified to the following conditions: 10 µl iQ Supermix (170–8864; Bio-Rad Laboratories), 0.5 µl of 10 µM forward primer, 0.5 µl of 10 µM reverse primer, 8 µl Millipore quality water and 1 µl template DNA were mixed to a total volume of 20 µl. The PCR reaction for all genes was performed in a Verity thermal cycler (Life Technologies, Grand Island, NY, USA) and consisted of a denaturation step at 95°C during 3 min, followed by 30 cycles of 30 sec at 95°C, 30 sec at 62°C and 30 sec at 72°C. Finally, an extended elongation step was performed during 5 min at 72°C. Five microlitres of each PCR product were visualized on a 2% Multi Purpose Tris-borate–EDTA agarose gel (11388991001; Roche Diagnostics, Almere, the Netherlands) containing 0.05% v/v of 10 mg/ml ethidium bromide (161-0433; Bio-Rad). PCR products were purified and subsequently sequenced (Macrogen, Amsterdam, the Netherlands) using the same primers as were used for the PCR reaction.
Table 4. Target genes, primer name, primer sequence and product size of the seven housekeeping genes used for multilocus sequence typing of E. coli (Wirth et al., Citation2006) isolated from the bone marrow of hens with EPS.
Analysis of multilocus sequence typing data
Consensus sequences were constructed using DNA baser software v3.5.4 (Heracle BioSoft SRL, Pitesti, Romania). Multiple alignments were made with Molecular Evolutionary Genetics Analysis version 6 (Tamura et al., Citation2011). Sequence data were imported in BioNumerics, version 7.2 (Applied Maths, Sint-Martens-Latem, Belgium). Allelic profiles were identified and sequence types (STs) and clonal complexes (CCs) were assigned using the University of Warwick database (http://mlst.warwick.ac.uk/mlst/). A similarity matrix was created based on the allelic profiles and the genetic relatedness of E. coli colonies was visualized using a minimum spanning tree.
Ethics
The study was approved by the Institutional Experimental Committee, DEC-Consult Foundation according to Dutch law on experimental animals (Wet op de dierproeven).
Results
All clinical healthy hens selected from flocks with EPS (n = 27) showed productive ovaries and the absence of lesions at gross post-mortem examination. Furthermore, bacteria were not isolated from their bone marrow. Results of gross post-mortem examination and bacteriology of dead hens are summarized in . Out of 102 dead hens selected for examination, 98 (96%) had died due to EPS; and from 92% (90/98) of these hens with EPS, E. coli was isolated from the bone marrow. Eighty-four per cent of dead hens with EPS showed peritonitis (82/98), 4% (4/98) had salpingitis and 84% (82/98) had fully productive ovaries.
Within hens, examining nine to 14 hens with EPS per flock and five E. coli colonies per hen by PFGE, the E. coli bacteria were always clonal. As an example, this clonality is shown in (Farm A). The number of E. coli genotypes within flocks with EPS ranged from one to five with genotype prevalence between 6 and 100% (). Two successive flocks of layers, which both developed EPS and were kept in a free-range system in the same house on one multiple-age farm (Flocks 1 and 2 of Farm C) shared three E. coli genotypes (), while two successive flocks of broiler breeders also both suffering from EPS and kept in the same house in a floor system at another farm (Flocks 1 and 2 of Farm D) had no E. coli genotypes in common ().
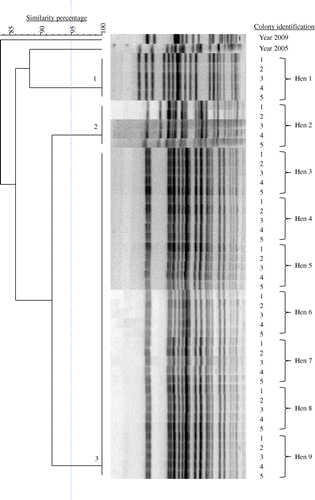
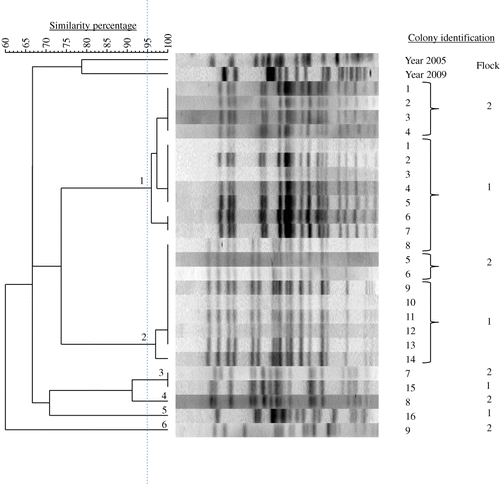
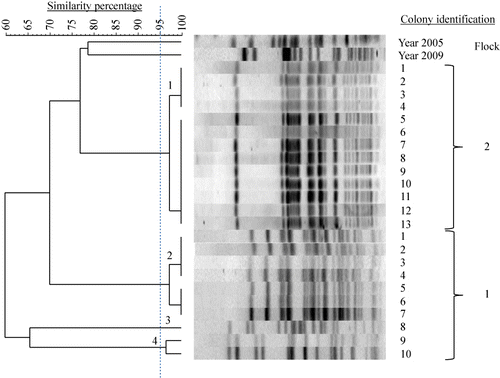
In total, 15 E. coli PFGE genotypes were detected in the six flocks examined. Farms had no E. coli genotypes in common and also genotypes isolated from layers with EPS previously (in 2005 and 2009) differed genetically from those found in the present study (). MLST results are listed in . Colonies belonging to the same PFGE type always had the same ST. MLST analysis revealed 10 different STs (ST 23, ST 56, ST 57, ST 117, ST 141, ST 351, ST 428, ST 429, ST 2535 and ST 4109) that correspond to a unique PFGE type, except for PFGE types 8, 9, 10 and 12, which all belong to the same ST (ST 95). One colony could not be classified to a ST assigned in the database. The colony representing PFGE type 11, designated Chicken/NL/Dev/SP01390Mhe/11, was submitted to the database and assigned to the new ST 4359. In addition, clonal relatedness was determined by classifying STs in CCs. The following CCs were found: CC 23, CC 95, CC 155 and CC 350. The remaining 12 colonies belonging to eight different STs could not be attributed to a CC in the database. PFGE typing of 39 E. coli colonies obtained from cases of EPS not included in this study revealed 36 different PFGE types (data not shown). Using MLST, 13 STs were detected in the former colonies, ST 10 (n = 1), ST 23 (n = 12), ST 58 (n = 1), ST 93 (n = 2), ST 95 (n = 8), ST 117 (n = 1), ST 140 (n = 8), ST 363 (n = 1), ST 369 (n = 1), ST 428 (n = 1), ST 429 (n = 1), ST 681 (n = 1) and ST 2944 (n = 1). The following five CCs could be assigned: CC 10 (n = 1), CC 23 (n = 13), CC 95 (n = 16), CC 155 (n = 1) and CC 168 (n = 2). Six colonies could not be attributed to a CC. Common STs in both sets of colonies (present study and the additional 39 colonies) were ST 23, ST 95, ST 117, ST 428, and ST 429. Common CCs were CC 23, CC 95 and CC 155. In , the MLST results of both sets of colonies and their genetic relatedness was visualized using a minimum spanning tree.
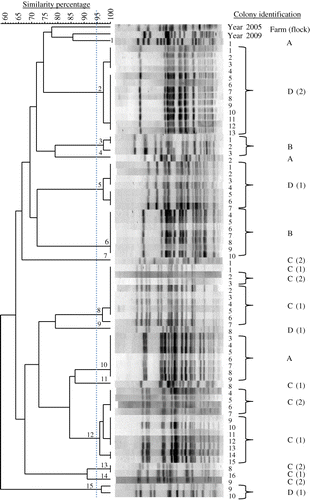
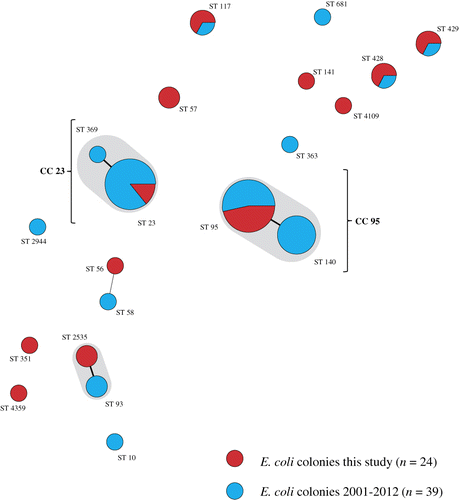
Table 5. PFGE type, farm, flock, allele numbers, sequence type and clonal complex of 24 E. coli colonies obtained from the bone marrow of hens with EPS analysed by multilocus sequence typing.
Discussion
A well-defined source, in terms of clinical signs and pathological lesions, from which microorganisms are obtained is essential for a meaningful interpretation of their biological characterization. Unfortunately, a number of studies on molecular typing and assessment of virulence factors of APEC concern bacteria that originate from poorly defined lesions, with colibacillosis often being the only pathological description (Mellata et al., Citation2003; Ewers et al., Citation2004; Kawano et al., Citation2006; Johnson et al., Citation2008). In some cases, however, E. coli was obtained from well-defined disease and lesions. Examples of such research are a study performed by Olsen et al. (Citation2011a) in which the clonality and virulence traits of E. coli associated with haemorrhagic septicaemia in turkeys was examined, studies concerning avian cellulitis (Singer et al., Citation2000; Jeffrey et al., Citation2002) and research on MLST and plasmid profile characterization of APEC associated with increased mortality in layers (Olsen et al., Citation2011b). In the present study we examined E. coli bacteria that also originated from well-defined disease outbreaks and lesions; that is, outbreaks of EPS in laying hens and in which only E. coli bacteria obtained from bone marrow were typed using PFGE and MLST.
The occurrence of virulence factors in the E. coli colonies was not assessed as the aim of the present research was to study the clonality of E. coli from EPS and not their virulence. However, the E. coli genotypes involved in EPS are probably virulent as apparently they are able to invade the bloodstream and subsequently the bone marrow in clinically healthy birds. This was corroborated by the absence of clinical signs of disease in all flocks (except Flock C1 in which lower egg production was observed) until EPS occurred.
A number of molecular typing methods for E. coli exist such as multi-locus enzyme electrophoresis (White et al., Citation1993), amplified ribosomal restriction analysis (Kawano et al., Citation2006), ribotyping (Olsen et al., Citation2011a), suppression subtractive hybridization (Mokady et al., Citation2005), PFGE (Singer et al., Citation2000; Ewers et al., Citation2004; Jeffrey et al., Citation2004; Kawano et al., Citation2006; Ozawa et al., Citation2010; Landman et al., Citation2013; Pires-dos-Santos et al., Citation2013) and MLST (Ewers et al., Citation2004; Ozawa et al., Citation2010; Pires-dos-Santos et al., Citation2013). The last two methods were chosen for the present investigation. PFGE is considered the most appropriate technique for the assessment of short-term epidemiological relatedness of bacterial strains as it identifies the microvariation required to distinguish between strains circulating within a geographic area (Maiden et al., Citation1998; Wieler & Laturnus, Citation2003; Goering, Citation2010). However, interpretation of banding patterns may be somewhat subjective making comparison of results between laboratories difficult. MLST based on sequences of seven housekeeping genes and lacking the disadvantages of PFGE (Maiden et al., Citation1998) was therefore performed on E. coli colonies belonging to each of the PFGE types detected in this study.
E. coli PFGE genotypes, which totalled 15, differed between farms and flocks except Flocks C1 and C2 who shared three genotypes. Although one to five genotypes were detected per flock, one or two genotypes dominated an EPS outbreak. In a number of cases a great genetic diversity of E. coli bacteria involved has been shown; for example, in SPS (Pires-dos-Santos et al., Citation2013) and in colibacillosis in chickens in Japan (Kawano et al., Citation2006). E. coli infections are often considered opportunistic whenever the bacteria of concern display great genetic variation. Limited genetic diversity of E. coli bacteria has been reported associated with acute haemorrhagic septicaemia in turkeys (Olsen et al., Citation2011a), in E. coli septicaemia lesions following cellulitis in chickens (Jeffrey et al., Citation2002) and in EPS cases found in free-range layer flocks (Olsen et al., Citation2011b). Also in the present study, limited genetic variation amongst E. coli colonies from bone marrow of laying hens with EPS was found. Such limited genetic variety is considered an indication for the non-opportunistic character of the E. coli infection, which has been confirmed experimentally for the two tested strains obtained from birds with EPS (Landman et al., Citation2010; Boelm et al., Citation2011).
In the present study, only one PFGE genotype was found in the bone marrow of individual birds. Considering that five colonies obtained from each of 64 individual birds (n = 320 colonies) were tested, failure to detect a genotype will be <1% in cases where the respective genotype occurs in all birds at a prevalence of ≥1.5% (Johnson et al., Citation2004). Thus, the chance that other genotypes present in single birds were missed is very small. The occurrence of one APEC genotype at bird level is in agreement with a study on cellulitis, where E. coli populations obtained from septicaemic lesions following cellulitis appeared to be homogeneous in single birds, while the cellulitis lesions contained heterogeneous E. coli bacteria (Singer et al., Citation2000; Jeffrey et al., Citation2002). An explanation was not found for the variation in genotypes found at flock level versus the occurrence of homogeneous E. coli populations originating from septicaemic organs in single birds.
Besides studying the clonality of E. coli colonies associated with EPS outbreaks, PFGE was used for molecular epidemiology by assessing the occurrence of persistent APEC in two sets of two successive flocks with this syndrome kept in the same houses on two farms. In one case, most colonies of the second flock (Flock C2, also suffering from EPS) belonged to two genotypes that also dominated in the previous flock (Flock C1). However, these flocks were housed on a multiple-age farm, which probably has favoured the persistence of the E. coli genotypes of concern. Colonies with the same PFGE type always had the same ST. However, four PFGE types shared ST 95. In the present study, 12 STs were detected, while Olsen et al. (Citation2011b) found eight STs in 10 flocks of free-range layers with EPS. Both studies revealed five STs in common (ST 23, ST 57, ST 95, ST 117 and ST 141).
Neither results of PFGE nor results of MLST were unambiguously related to APEC from EPS. The presence of large plasmids is considered characteristic of APEC (Doetkott et al., Citation1996) and is probably an important factor determining virulence (Cusumano et al., Citation2010). Olsen et al. (Citation2011b) also found large plasmids in E. coli bacteria from EPS cases, which were of replicon type IncFIB. Future research focusing on the characterization and transmission of E. coli plasmids may help to better understand the disease-inducing potential and pathogenesis of E. coli strains that induce EPS.
The EPS outbreaks studied occurred in six flocks of laying hens (both layer type and meat type) housed in different systems and took place on four farms that were considered as separate epidemiological units. In three flocks, disease started in the period from the start of production to peak production, while in the remaining cases it occurred at older ages. In both broiler breeder flocks with EPS included in this study (Farm D), cockerels showed high mortality including culling. Although one cannot rule out that this may (in part) have been due to EPS because cockerels were not sampled, very high mortality rates (including culling) of about 43% are common amongst broiler breeder males (Peak & Brake, Citation2001). Remarkably, the mortality in Flock A1 increased dramatically following the removal of 10,560 hens from the flock of approximately 50,000 layers at 54 weeks of age. This might be attributed to the generation of airborne dust probably containing E. coli bacteria and the subsequent respiratory transmission of EPS. The latter is in agreement with experimental studies on the pathogenesis of this disease (Landman et al., Citation2013). One flock with EPS showed reduced egg production (Farm C, Flock 1). However, it is not likely that EPS causes drops in egg production because in five other EPS flocks it was not affected at all. EPS continued until the end of the production period in five flocks. The type and distribution of lesions and the percentage of E. coli re-isolations from bone marrow () paralleled those of a previous field study (Landman & Cornelissen, Citation2006).
Flock D1 was treated several times with antibiotics, but without significant effect. This might have been due to resistance (doxycycline) or intermediate sensitivity (flumequin) of the E. coli bacteria or the lack of absorption of the antibiotic (colistin). However, treatment with enrofloxacin to which the E. coli bacteria were sensitive or intermediary sensitive also failed. Possibly the continuous airborne challenge with E. coli strains able to induce EPS may explain the failure of the antibiotic treatment of EPS reported here and observed frequently in the field. Due to the shortcomings of antibiotic treatment for colibacillosis (development of resistance, residues in eggs, birds in a flock are not ill synchronically and remain in a contaminated environment), vaccination is often used as a prevention strategy. However, the efficacy of vaccines has been questionable because no single trait or group of traits that define APEC involved in EPS have been identified (making selection of candidate vaccines difficult) and in general cross-protection is lacking (Deb & Harry, Citation1976), probably due to the great diversity of serovars of E. coli (Ewers et al., Citation2007). Autovaccines are therefore often used in the field and are expected to be highly effective if birds are challenged homologously (Deb & Harry, Citation1976; Boelm et al., Citation2011). Although vaccination of Flock D2 with the autovaccine failed, probably due to heterologous challenge, it may have reduced the number of E. coli genotypes involved to one, which was distinct from those found in Flock D1. Genotyping E. coli bacteria associated with an EPS outbreak and inclusion of the most important genotypes in an autovaccine will probably improve its efficacy provided the APEC strains of concern persist at the farm. The latter will result in a homologous challenge, as would probably have occurred in the second flock of multiple-age Farm C. In cases where the persistence of APEC strains associated with EPS is found to occur on a large scale, genotyping of these strains as a tool to select strains for the production of autovaccines could be considered a routine procedure.
Acknowledgements
The authors thank G.J. Boelm and W. Vije for their technical assistance with this study.
Funding
The authors thank the Dutch Commodity Board for Poultry and Eggs for funding this work.
Additional information
Funding
References
- Barnes, H.J., Nolan, L.K. & Vaillancourt, J.P. (2008). Colibacillosis. In Y.M. Saif, A.M. Fadly, J.R. Glisson, L.R. McDougald, L.K. Nolan, & D.E. Swayne (Eds.). Diseases of Poultry (12 ed., pp. 691–732). Ames: Iowa State Press and Blackwell Publishing Company.
- Bisgaard, M. & Dam, A. (1981). Salpingitis in poultry. II. Prevalence, bacteriology, and possible pathogenesis in egg-laying chickens. Nordisk Veterinaer Medicin, 33, 81–89.
- Boelm, G.J., Heuvelink, A.E. & Landman, W.J.M. (2011). Induction of Escherichia coli peritonitis in layers and the preventive effect of inactivated E. coli autovaccines. In XVIIth World Veterinary Poultry Association Congress (pp. 641–646). Cancún, Méjico.
- Cusumano, C.K., Hung, C.S., Chen, S.L. & Hultgren, S.J. (2010). Virulence plasmid harbored by uropathogenic Escherichia coli functions in acute stages of pathogenesis. Infection and Immunity, 78, 1457–1467. 10.1128/IAI.01260-09
- Deb, J.R. & Harry, E.G. (1976). Laboratory trials with inactivated vaccines against Escherichia coli (O78 K80) infection in fowls. Research in Veterinary Science, 20, 131–138.
- Dhillon, A.S. & Jack, O.K. (1996). Two outbreaks of colibacillosis in commercial caged layers. Avian Diseases, 40, 742–746. 10.2307/1592290
- Doetkott, D.M., Nolan, L.K., Giddings, C.W. & Berryhill, D.L. (1996). Large plasmids of avian Escherichia coli isolates. Avian Diseases, 40, 927–930. 10.2307/1592319
- Ewers, C., Janssen, T., Kiessling, S., Philipp, H.C. & Wieler, L.H. (2004). Molecular epidemiology of avian pathogenic Escherichia coli (APEC) isolated from colisepticemia in poultry. Veterinary Microbiology, 104, 91–101. 10.1016/j.vetmic.2004.09.008
- Ewers, C., Li, G., Wilking, H., Kiessling, S., Alt, K., Antao, E.M., Laturnus, C., Diehl, I., Glodde, S., Homeier, T., Bohnke, U., Steinruck, H., Philipp, H.C. & Wieler, L.H. (2007). Avian pathogenic, uropathogenic, and newborn meningitis-causing Escherichia coli: how closely related are they? International Journal of Medical Microbiology, 297, 163–176. 10.1016/j.ijmm.2007.01.003
- Goering, R.V. (2010). Pulsed field gel electrophoresis: a review of application and interpretation in the molecular epidemiology of infectious disease. Infection, Genetics and Evolution, 10, 866–875. 10.1016/j.meegid.2010.07.023
- Gross, W.B. & Siegel, P.B. (1959). Coliform peritonitis of chickens. Avian Diseases, 3, 370–373. 10.2307/1587575
- Jeffrey, J.S., Nolan, L.K., Tonooka, K.H., Wolfe, S., Giddings, C.W., Horne, S.M., Foley, S.L., Lynne, A.M., Ebert, J.O., Elijah, L.M., Bjorklund, G., Pfaff-McDonough, S.J., Singer, R.S. & Doetkott, C. (2002). Virulence factors of Escherichia coli from cellulitis or colisepticemia lesions in chickens. Avian Diseases, 46, 48–52. 10.1637/0005-2086(2002)046[0048:VFOECF]2.0.CO;2
- Jeffrey, J.S., Singer, R.S., O'Connor, R. & Atwill, E.R. (2004). Prevalence of pathogenic Escherichia coli in the broiler house environment. Avian Diseases, 48, 189–195. 10.1637/7043
- Johnson, T.J., Wannemuehler, Y., Johnson, S.J., Stell, A.L., Doetkott, C., Johnson, J.R., Kim, K.S., Spanjaard, L. & Nolan, L.K. (2008). Comparison of extraintestinal pathogenic Escherichia coli strains from human and avian sources reveals a mixed subset representing potential zoonotic pathogens. Applied Environmental Microbiology, 74, 7043–7050. 10.1128/AEM.01395-08
- Johnson, W.O., Su, C.L., Gardner, I.A. & Christensen, R. (2004). Sample size calculations for surveys to substantiate freedom of populations from infectious agents. Biometrics, 60, 165–171. 10.1111/j.0006-341X.2004.00143.x
- Jordan, F.T., Williams, N.J., Wattret, A. & Jones, T. (2005). Observations on salpingitis, peritonitis and salpingoperitonitis in a layer breeder flock. The Veterinary Record, 157, 573–577.
- Kawano, M., Yaguchi, K. & Osawa, R. (2006). Genotypic analyses of Escherichia coli isolated from chickens with colibacillosis and apparently healthy chickens in Japan. Microbiology & Immunology, 50, 961–966. 10.1111/j.1348-0421.2006.tb03872.x
- Landman, W.J.M. & Cornelissen, R.A. (2006). Escherichia coli salpingitis and peritonitis in layer chickens: an overview. Tijdschrift voor Diergeneeskunde, 131, 814–822.
- Landman, W.J.M., Heuvelink, A. & Van Eck, J.H. (2013). Reproduction of the Escherichia coli peritonitis syndrome in laying hens. Avian Pathology, 42, 157–162. 10.1080/03079457.2013.775694
- Landman, W.J.M., Heuvelink, A.E. & Van Eck, J.H.H. (2010). Induction of Escherichia coli peritonitis in layers. In 59th Western Poultry Disease Conference (pp. 66–69). Vancouver, Canada.
- Maiden, M.C., Bygraves, J.A., Feil, E., Morelli, G., Russell, J.E., Urwin, R., Zhang, Q., Zhou, J., Zurth, K., Caugant, D.A., Feavers, I.M., Achtman, M. & Spratt, B.G. (1998). Multilocus sequence typing: a portable approach to the identification of clones within populations of pathogenic microorganisms. Proceedings of the National Academy of Sciences of the United States of America, 95, 3140–3145. 10.1073/pnas.95.6.3140
- Mellata, M., Dho-Moulin, M., Dozois, C.M., Curtiss III, R., Brown, P.K., Arne, P., Bree, A., Desautels, C. & Fairbrother, J.M. (2003). Role of virulence factors in resistance of avian pathogenic Escherichia coli to serum and in pathogenicity. Infection and Immunity, 71, 536–540. 10.1128/IAI.71.1.536-540.2003
- Mokady, D., Gophna, U. & Ron, E.Z. (2005). Extensive gene diversity in septicemic Escherichia coli strains. Journal of Clinical Microbiology, 43, 66–73. 10.1128/JCM.43.1.66-73.2005
- Olsen, R.H., Chadfield, M.S., Christensen, J.P., Scheutz, F., Christensen, H. & Bisgaard, M. (2011a). Clonality and virulence traits of Escherichia coli associated with haemorrhagic septicaemia in turkeys. Avian Pathology, 40, 587–595. 10.1080/03079457.2011.618942
- Olsen, R.H., Stockholm, N.M., Permin, A., Christensen, J.P., Christensen, H. & Bisgaard, M. (2011b). Multi-locus sequence typing and plasmid profile characterization of avian pathogenic Escherichia coli associated with increased mortality in free-range layer flocks. Avian Pathology, 40, 437–444. 10.1080/03079457.2011.592822
- Ozawa, M., Baba, K. & Asai, T. (2010). Molecular typing of avian pathogenic Escherichia coli O78 strains in Japan by using multilocus sequence typing and pulsed-field gel electrophoresis. The Journal of Veterinary Medical Science, 72, 1517–1520. 10.1292/jvms.10-0174
- Peak, S.D. & Brake, J. (2001). Influence of feeding program on broiler breeder mortality. In Proceedings of the 2001 Australian Poultry Science Symposium (pp. 113–120). Sydney, Australia.
- Pires-dos-Santos, T., Bisgaard, M. & Christensen, H. (2013). Genetic diversity and virulence profiles of Escherichia coli causing salpingitis and peritonitis in broiler breeders. Veterinary Microbiology, 162, 873–880. 10.1016/j.vetmic.2012.11.008
- Raviv, Z., Ferguson-Noel, N., Laibinis, V., Wooten, R. & Kleven, S.H. (2007). Role of Mycoplasma synoviae in commercial layer Escherichia coli peritonitis syndrome. Avian Diseases, 51, 685–690. 10.1637/0005-2086(2007)51[685:ROMSIC]2.0.CO;2
- Singer, R.S., Johnson, W.O., Jeffrey, J.S., Chin, R.P., Carpenter, T.E., Atwill, E.R. & Hirsh, D.C. (2000). A statistical model for assessing sample size for bacterial colony selection: a case study of Escherichia coli and avian cellulitis. Journal of Veterinary Diagnostic Investigation, 12, 118–125. 10.1177/104063870001200203
- Stokholm, N.M., Permin, A., Bisgaard, M. & Christensen, J.P. (2010). Causes of mortality in commercial organic layers in Denmark. Avian Diseases, 54, 1241–1250. 10.1637/9375-041910-Reg.1
- Tamura, K., Peterson, D., Peterson, N., Stecher, G., Nei, M. & Kumar, S. (2011). MEGA5: molecular evolutionary genetics analysis using maximum likelihood, evolutionary distance, and maximum parsimony methods. Molecular Biology and Evolution, 28, 2731–2739. 10.1093/molbev/msr121
- Tenover, F.C., Arbeit, R.D., Goering, R.V., Mickelsen, P.A., Murray, B.E., Persing, D.H. & Swaminathan, B. (1995). Interpreting chromosomal DNA restriction patterns produced by pulsed-field gel electrophoresis: criteria for bacterial strain typing. Journal of Clinical Microbiology, 33, 2233–2239.
- Vandekerchove, D., De Herdt, P., Laevens, H. & Pasmans, F. (2004). Colibacillosis in caged layer hens: characteristics of the disease and the aetiological agent. Avian Pathology, 33, 117–125. 10.1080/03079450310001642149
- White, D.G., Wilson, R.A., Emery, D.A., Nagaraja, K.V. & Whittam, T.S. (1993). Clonal diversity among strains of Escherichia coli incriminated in turkey colisepticemia. Veterinary Microbiology, 34, 19–34. 10.1016/0378-1135(93)90004-Q
- Wieler, L.H. & Laturnus, C. (2003). Classification and typing of campylobacter: application of genotypical methods in veterinary medicine. Genome Letters, 2, 53–61. 10.1166/gl.2003.000
- Wirth, T., Falush, D., Lan, R., Colles, F., Mensa, P., Wieler, L.H., Karch, H., Reeves, P.R., Maiden, M.C., Ochman, H. & Achtman, M. (2006). Sex and virulence in Escherichia coli: an evolutionary perspective. Molecular Microbiology, 60, 1136–1151. 10.1111/j.1365-2958.2006.05172.x
- Yogaratnam, V. (1995). Analysis of the causes of high rates of carcase rejection at a poultry processing plant. The Veterinary Record, 137, 215–217. 10.1136/vr.137.9.215
- Zanella, A., Alborali, G.L., Bardotti, M., Candotti, P., Guadagnini, P.F., Martino, P.A. & Stonfer, M. (2000). Severe Escherichia coli O111 septicaemia and polyserositis in hens at the start of lay. Avian Pathology, 29, 311–317. 10.1080/03079450050118430