Abstract
Avian infectious laryngotracheitis virus (ILTV) is an alphaherpesvirus of poultry that is spread worldwide. ILTV enters its host via the respiratory tract and the eyes. Although ILTV has been known for a long time, the replication characteristics of the virus in the respiratory and conjunctival mucosa are still poorly studied. To study these characteristics, two in vitro explant models were developed. Light microscopy and fluorescent terminal deoxynucleotidyl transferase dUTP nick end-labelling staining were used to evaluate the viability of mucosal explants, which were found to be viable up to the end of the experiment at 96 h of cultivation. The tracheal and conjunctival mucosal explants were inoculated with ILTV and collected at 0, 24, 48 and 72 h post inoculation (p.i.). ILTV spread in a plaque-wise manner in both mucosae. A reproducible quantitative analysis of this mucosal spread was evaluated by measuring plaque numbers, plaque latitude and invasion depth underneath the basement membrane. No major differences in plaque numbers were observed over time. Plaque latitude progressively increased to 70.4 ± 12.9 μm in the trachea and 97.8 ± 9.5 μm in the conjunctiva at 72 h p.i. The virus had difficulty crossing the basement membrane and was first observed only at 48 h p.i. The virus was observed at 72 h p.i. in 56% (trachea) and 74% (conjunctiva) of the plaques. Viability analysis of infected explants indicated that ILTV blocks apoptosis in infected cells of both mucosae but activates apoptosis in bystander cells.
Introduction
Infectious laryngotracheitis virus (ILTV) is an avian herpesvirus, classified within the order Herpesvirales, family Herpesviridae, subfamily Alphaherpesvirinae and genus Iltovirus. The virus is taxonomically designated Gallid herpesvirus 1 (GaHV1) (Davison, Citation2010). ILTV is a contagious respiratory virus of chickens with an active cytolytic replication that takes place in the epithelium of the trachea, larynx and conjunctiva, which may lead to severe mucosal epithelial damage and haemorrhages. These pathological changes lead to infectious laryngotracheitis (Bang & Bang, Citation1967; Tully, Citation1995; Guy & Bagust, Citation2003). During ILTV infection, it is not clear whether a viraemia occurs (Bagust, Citation1986; Guy & Bagust, Citation2003; Zhao et al., Citation2013). After an acute phase of infection, ILTV, like other alphaherpesviruses, establishes a lifelong latency within the trigeminal ganglion of the central nervous system. During stress, re-activation of the virus may occur, leading to the spread of ILTV to susceptible contact animals (Fuchs et al., Citation2007). The characteristic clinical signs of infectious laryngotracheitis during a mild form are nasal discharge, conjunctivitis and drops in egg production. During the severe form, additional signs are gasping, bloody mucous expectoration, dyspnoea and death due to asphyxia (Fuchs et al., Citation2007). Infectious laryngotracheitis has an economic impact by causing severe production losses due to a high morbidity and mortality and also by mass vaccination (Jones, Citation2010).
ILTV enters its host via the respiratory and ocular routes. After entry, initial virus replication takes place in the epithelium of the respiratory and conjunctival mucosa (Guy & Bagust, Citation2003). ILTV may then invade through the basement membrane (BM). During evolution of all animal species, most alphaherpesviruses have acquired intriguing tools to invade through the BM of the mucosa and to disseminate in the underlying lamina propria (Glorieux et al., Citation2009; Steukers et al., Citation2012). The precise replication characteristics and invasion mechanism of ILTV at the primary replication site (i.e. in the respiratory and conjunctival mucosa) are unknown.
The replication characteristics of ILTV in the respiratory and conjunctival mucosa may be investigated by performing animal experiments (Bang & Bang, Citation1967; Kirkpatrick et al., Citation2006). However, in vivo studies are contested more and more for ethical reasons. Furthermore, animal experiments are prone to large inter-animal variation. Hence, a fully standardized in vitro model, which mimics the in vivo situation, is useful to study host–virus interactions during the early stages of infection.
The tracheal organ culture (TOC) system is commonly used to study host–pathogen interactions (Reemers et al., Citation2009). Generally, tracheal rings from chicks or 19-day-old chicken embryos are used for TOC (Jones & Hennion, Citation2008; Zhang et al., Citation2012).TOCs have been used for a long time for diagnosis and pathogenesis studies of ILTV (Ide, Citation1978; Bagust, Citation1986). The viability of TOCs has generally been analysed by two methods: one based on the ciliary beating and another based on the ciliary removal of latex beads (Anderton et al., Citation2004). However, these two methods evaluate only the epithelium viability and not the viability of the lamina propria of the tracheal mucosa and the underlying submucosa. Chicken conjunctival organ cultures (COC) have previously been used to study host–virus interactions (Darbyshire et al., Citation1976, but viability analysis was not performed. To study replication characteristics and the invasion mechanism of ILTV through the BM towards the lamina propria and the underlying submucosa, it is crucial to keep these layers viable.
The aims of the present study were to evaluate quantitatively the replication characteristics of ILTV in in vitro models of chicken tracheal and conjunctival mucosa explants, and to analyse the cell viability of infected mucosal explants. Hence, in vitro TOC and COC were established. The TOC and COC explants (organs) were placed on fine-meshed gauze and maintained for 96 h at an air–liquid interface. A viability analysis was performed using light microscopy (cilia beating) and fluorescence microscopy (terminal deoxynucleotidyl transferase dUTP nick end-labelling [TUNEL]-positive cells). The mucosal explants were inoculated with ILTV and replication kinetics were evaluated quantatively by measuring plaque number, plaque latitude and invasion depth underneath the BM in the TOC and COC. A viability analysis of infected mucosal explants was performed to examine the outcome of an ILTV infection in these mucosae.
Materials and Methods
Virus
A pathogenic Belgian isolate of ILTV (U76/1035) was used in this study (Meulemans & Halen, Citation1978). Stock virus was propagated in primary chicken embryo kidney cells that were collected from 19-day-old embryonated specific pathogen free eggs by trypsin disaggregation. Cells were grown in minimum essential medium (Gibco Life Technologies, Paisley, UK) supplemented with 2% foetal calf serum (Gibco). Virus titration was performed on the chorioallantoic membrane of 10-day-old chicken embryos to determine the median embryo infective dose (EID50) per millilitre. The ILTV strain had previously received an unknown number of passages. The strain was given a further passage in this laboratory and this material was used to inoculate the explants.
Isolation and cultivation of chicken tracheal and conjunctival explants
This study was performed in agreement with the guidelines of the Local Ethical and Animal Welfare Committee of the Faculty of Veterinary Medicine of Ghent University. Specific pathogen free chickens were killed humanely by intravenous injection of sodium pentobarbital (100 mg/kg body weight) into the brachial wing vein. Tracheas and conjunctivas were collected from three chickens 4 to 8 weeks old. Tracheas were carefully split into two equal halves with sharp surgical blades (Swann-Morton Ltd, Sheffield, UK). Conjunctival mucosa folds were carefully dissected from the underlying layers. TOC and COC explants covering a total area of 10 mm2 were made and placed on fine-meshed gauze in six-well culture plates (Nunc, Roskilde, Denmark), epithelium upwards. The explants were maintained in serum-free medium (50% Ham’s F12 [Gibco]/50% Dulbecco’s modified Eagle’s medium [Gibco]) supplemented with 100 U/ml penicillin (Continental Pharma, Brussels, Belgium), 0.1 mg/ml streptomycin (Certa, Braine l'Alleud, Belgium), 1 μg/ml gentamycin (Gibco) and 25 mM HEPES (Gibco). The epithelium of the explants was slightly immersed in the fluid to achieve an air–liquid interface; that is, just a thin film of fluid covering the epithelium. During the entire cultivation period, medium was not replaced. Explants were maintained up to 96 h at 37°C and 5% carbon dioxide.
Ciliary beating
Ciliary beating of the TOCs was checked on a daily basis with an Olympus CKX 31 light microscope (Olympus, Tokyo, Japan) at ×20 magnification.
Viability analysis by TUNEL assay
A viability analysis was performed in three independent experiments. TOC and COC were collected at 0, 24, 48, 72 and 96 h of in vitro cultivation. The In Situ Cell Death Detection Kit (Fluorescein; Roche Diagnostics GmbH, Mannheim, Germany), based on TUNEL (Roche Diagnostics GmbH, Mannheim, Germany), was used to detect apoptotic cells. The test was performed according to the manufacturer’s guidelines. Hoechst 33342 (Molecular Probes, Eugene, OR, USA) was used to visualize cell nuclei. The percentage of TUNEL-positive cells was determined in five randomly selected fields of 100 cells in both the epithelium and lamina propria with a fluorescence microscope (Leica DM RBE microscope; Leica Microsystems GmbH, Heidelberg, Germany).
Inoculation of GaHV1 (ILTV) and evaluation of mucosal spread
The tracheas and conjunctivas of three chickens were used. Explants were inoculated at 24 h of cultivation with ILTV (U76/1035). For the inoculation, explants were taken from their gauze and placed at the bottom of a 24-well plate with the epithelial surface upwards. Explants were washed twice with warm serum-free medium and inoculated with 1 ml virus stock containing log10 6.0 EID50 (1 h, 37°C, 5% carbon dioxide). After 1 h, inoculated explants were washed three times with warm medium and placed back on the gauze. Explants were collected at 0, 24, 48 and 72 h post inoculation (p.i.), embedded in Methocel® (Fluka Chemie AG, Buchs, Switzerland) and frozen at –70°C. Inoculation was performed for three different chickens. For each chicken, one explant was collected at each time point p.i. As a parameter of productive replication, virus titres were checked in the supernatant of inoculated explants in one replicate.
Immunofluorescence staining
At 0, 24, 48 and 72 h p.i., 150 consecutive cryosections of 15 μm were prepared from the frozen explants and the cryosections were fixed in methanol (25 min, –20°C). The cryosections were first stained for collagen IV, which is abundantly present in the BM and connective tissue, and next for ILTV antigens. The cryosections were incubated (1 h, 37°C) with goat anti-collagen IV antibodies (Southern Biotech, Birmingham, AL, USA) (1:50 in phosphate-buffered saline [PBS]), after which cryosections were washed three times (PBS), incubated (1 h, 37°C) with biotinylated rabbit anti-goat IgG antibodies (1:100 in PBS) and washed three times after incubation. Cryosections were then incubated (1 h, 37°C) simultaneously with streptavidin-Texas Red (Molecular Probes) (1:50 in PBS) and mouse monoclonal anti-ILTV gC antibodies (1:50 in PBS). (The monoclonal antibody against ILTV gC was provided by the Institute of Molecular Biology, Friedrich-Loeffler-Institute, Federal Research Institute for Animal Health, Greifswald-InselRiems, Germany.) Subsequently, after washing, the sections were incubated (1 h, 37°C) with fluorescein isothiocyanate-labelled goat anti-mouse IgG antibodies (Molecular Probes) (1:100 in PBS). Finally, after washing, the sections were incubated (10 min, room temperature) with Hoechst 33342 (1:100 in PBS), washed and mounted with glycerin-DABCO (Janssen Chimica, Beese, Belgium).
Evaluation of ILTV mucosal spread
A confocal microscope (Leica TCS SPE confocal microscope) was used for the analysis of ILTV replication in mucosal explants. Solid-state lasers were used to excite Texas Red (561 nm) and fluorescein isothiocyanate (488 nm) fluorophores. We analysed the replication characteristics of ILTV by a reproducible quantitative analysis system described by Glorieux et al. (Citation2009). Briefly, at 24, 48 and 72 h p.i. the average number of plaques was counted in a surface area of 8 mm2 of the explants (150 consecutive cryosections) derived from both the trachea and conjunctiva. Using the Leica confocal software, plaque dimensions, latitude and invasion depth (distance covered by ILTV underneath the BM) were measured at the maximal size for at least 10 different plaques per chicken at 0, 24, 48 and 72 h p.i. A maximum intensity projection or extended focus image of the confocal microscope was used to display three-dimensional information of the plaque. Borders and edges of the explant were excluded from the analysis; that is, only middle regions of the explant were considered.
Cell viability in ILTV-infected tracheal and conjunctival mucosa
The TUNEL assay was performed to detect apoptotic cells in infected TOC and COC mucosa explants. Immunofluorescence staining was performed to detect ILTV antigens. The protocol of both techniques is described above. TUNEL-positive cells were counted at 0, 24, 48 and 72 h p.i. Two regions of interest were taken into account for calculation: regions containing ILTV-negative cells; and regions containing ILTV-positive cells (plaque). In an ILTV-negative region, 10 randomly selected fields of 100 cells were evaluated in both the epithelium and the lamina propria. In regions of ILTV-positive cells, 10 randomly selected ILTV plaques were evaluated in both the epithelium and the lamina propria. The experiment was performed in triplicate.
Statistical analysis
SigmaPlot (Systat Software, Inc., San Jose, CA, USA) software was used to analyse the data statistically for one-way analysis of variance. The results of three independent experiments are shown as the mean ± standard deviation (SD) and P < 0.05 was considered significant.
Results
Ciliary beating
The ciliary beating of the tracheal explants was normal until the end of the study (96 h of cultivation).
Viability of tracheal and conjunctival mucosa explants
The viability of the TOC and COC mucosa explants was assessed based on the percentage of TUNEL-positive cells. We did not observe a significant increase in the number of positive cells at 0, 24, 48, 72 and 96 h of in vitro cultivation for either the epithelium or the lamina propria (). The percentage of TUNEL-positive cells in the epithelium of the trachea and conjunctiva ranged from 0.0 ± 0.0 to 0.9 ± 0.1% at 0 and 96 h, respectively. In the lamina propria of the trachea and conjunctiva, the percentage of TUNEL-positive cells ranged from 0.0 ± 0.0 to 1.4 ± 1.8% at 0 and 96 h respectively. Negligible mortality was observed in the tracheal cartilage.
Table 1. Apoptosis in the epithelium and lamina propria of chicken tracheal mucosa explants during in vitro cultivation.
GaHV1 (ILTV) interactions with tracheal and conjunctival mucosa
Evaluation of ILTV mucosal spread Inoculation of chicken tracheal and conjunctival mucosa explants with ILTV led to the formation of viral antigen-positive plaques (group of closely connected cells) in the mucosa. At 0 h, no infection was observed. At 24, 48 and 72 h p.i., clear ILTV-infected plaques were found in the tracheal and conjunctival mucosa. Representative images of plaques at 24 h p.i. are illustrated in . We observed ILTV infection only in the epithelial cells. We did not observe infection at the edges and ventral region of the cryosections. Dissemination kinetics of plaques in the mucosa was evaluated by measuring the plaque number, maximal plaque latitude and invasion depth underneath the BM at 0, 24, 48 and 72 h p.i. The viral titres in the supernatant of inoculated explants increased from log10 2.7 EID50/ml at 24 h p.i. to log10 4.3 EID50/ml at 72 h p.i.
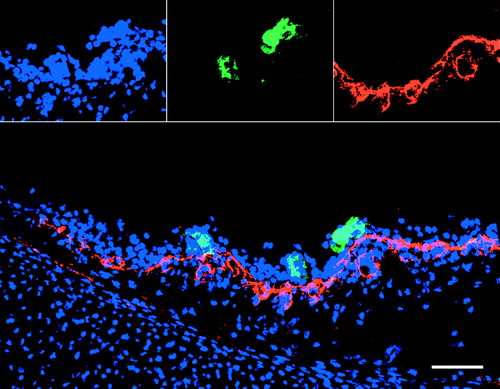
Plaque number
Mucosal explants covering a total area of 10 mm2 were evaluated using a Leica confocal microscope. Borders and edges of the explants were excluded from the analysis. The results for the average number of plaques are given per 8 mm2 or per one explant (150 consecutive cryosections). Mean values ± SD are represented in for three independent experiments. The number of plaques slightly increased with time.
Plaque latitude
Plaque latitudes were measured using Leica confocal software. Mean values ± SD are represented in for three independent experiments. The plaque latitude increased steadily over time from 0 to 72 h p.i. In the tracheal mucosa, the latitude increased from 29.4 ± 9.0 μm (24 h p.i.) to 55.4 ± 5.6 μm (48 h p.i.) and 70.4 ± 12.9 μm (72 h p.i.). In the conjunctiva, the latitude increased from 17.2 ± 3.4 μm (24 h p.i.) to 59.8 ± 5.3 μm (48 h p.i.) and 97.8 ± 9.5 μm (72 h p.i.).
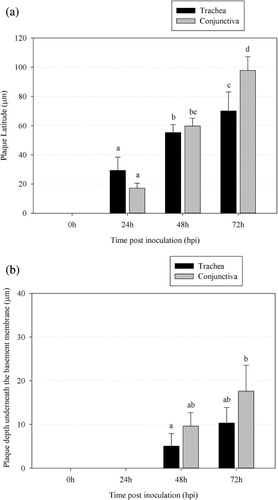
Plaque invasion depth
Plaque invasion is the vertical spread of ILTV in the mucosa. The plaque invasion depth is the distance covered by ILTV-infected tissue underneath the BM (Glorieux et al., Citation2009). Plaque invasion depths were measured using Leica confocal software. The evolution of ILTV plaque formation at 0, 24, 48 and 72 h p.i. in the trachea and conjunctiva are illustrated in . At 0 and 24 h p.i., infected plaques did not cross the BM. ILTV started BM invasion slowly, as infected plaques only crossed the BM between 24 and 48 h p.i. At 48 h p.i., about 31% of the plaques showed invasion through the BM of the trachea and about 43% of the plaques showed invasion through the BM of the conjunctiva. At 72 h p.i., this percentage increased to 56% in the trachea and 74% in the conjunctiva. Mean values ± SD are represented in for three independent experiments. The average depths underneath the BM increased from 5.1 ± 2.9 μm at 48 h p.i. to 10.3 ± 3.6 μm at 72 h p.i. among all plaques of the tracheal mucosa. In the conjunctival mucosa, the average depths underneath the BM increased from 9.6 ± 3.1 μm at 48 h p.i. to 17.6 ± 6 μm at 72 h p.i. among all plaques.
Cell viability in ILTV-infected tracheal and conjunctival mucosa
After inoculation with ILTV, a large number of cells were ILTV infected. A small number of TUNEL-positive cells were observed in both regions of ILTV-infected and non-infected cells (). In the ILTV-infected regions, TUNEL-positive cells were usually observed in the vicinity of ILTV-infected cells. The ILTV-infected cells were mostly not TUNEL-positive. In the tracheal and conjunctival epithelium, the percentage of ILTV-positive cells that were TUNEL-positive ranged from 0.4% at 24 h p.i. to 1.4% at 72 h p.i. A representative confocal image illustrating TUNEL-positive cells in the vicinity of ILTV-infected cells but not in the ILTV-infected plaque is shown in .
Table 2. Percentage of TUNEL-positive cells in both epithelium and lamina propria of a region of ILTV-negative and of a region of ILTV-positive cells (plaque) at different times post inoculation.
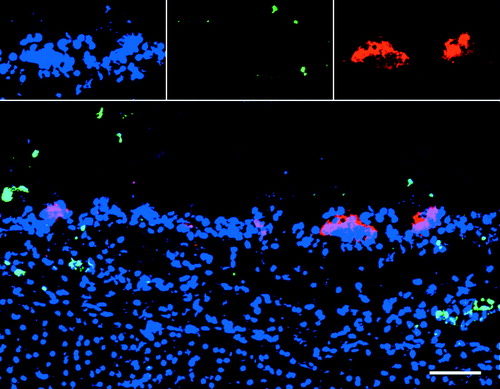
Discussion
In the present study, chicken tracheal and conjunctival mucosa explants were established, the replication kinetics of ILTV investigated in them and cell viability was analysed. Even though in vivo laboratory animals are the best system for studying host–pathogen interactions, physiological inter-individual differences and different environmental conditions are important drawbacks. The use of an in vitro culture system offers the opportunity to study host–pathogen interactions under more controlled conditions. In cell cultures, cell–cell and cell–extracellular matrix interactions are reduced due to the lack of a three-dimensional architecture of the culture (Freshney, Citation2005). In vitro explant (organ) cultures are excellent alternative models that mimic natural conditions and are in line with the ‘three Rs’ principle: Reduction (reduction of number of animals), Replacement (no use of living animals) and Refinement (minimizing the pain) (Russell & Burch, Citation1959). A major advantage of this in vitro model is that explants of the same animals can be used to compare different viral strains.
The culture technique that was used in this study for chicken TOC and COC explants is similar to that used for porcine (Glorieux et al., Citation2007), bovine (Steukers et al., Citation2012), equine (Vandekerckhove et al., Citation2009) and human (Glorieux et al., Citation2011) respiratory explants. To our knowledge, there are no reports on the viability of cells in the epithelial layers, lamina propria and underlying connective tissue of chicken TOC and COC. Hence, in this study the viability of epithelial cells and cells in the lamina propria of TOC and COC were evaluated by quantifying TUNEL-positive cells at 0, 24, 48, 72 and 96 h of in vitro cultivation. The TUNEL-positive cells remained below 1.5% up to 96 h of cultivation in both the epithelium and lamina propria of TOC and COC. We can thus state that both TOC and COC were successfully maintained for at least 96 h in culture at an air–liquid interface without significant changes in tissue viability.
The term “TOC” can be used to address both explant cultures on a gauze and on tracheal ring cultures. We did not observe differences in the viability of cells between tracheal rings and the explants on the gauze system (data not shown). The explant on the gauze system is more useful to cultivate different mucosal tissues such as nasal septum, conchae, conjunctival mucosa, genital mucosa and cornea and to compare viral replication in these tissues. We have also established corneal mucosa explants but did not observe ILTV infection of them (data not shown).
The chicken TOC and COC were susceptible to ILTV infection. The infection pattern of ILTV in the mucosal explants mimics extremely well the in vivo observations made by Bang & Bang (Citation1967). The purpose of the present study was to analyse the behaviour of ILTV in the mucosa by immunofluorescence and mathematical quantitative analysis using confocal microscopy and software ImageJ, as described by Glorieux et al. (Citation2009). By doing so, a complete three-dimensional picture of the horizontal and vertical spread of ILTV in the mucosa was obtained. This novel approach is an ideal tool for studying cellular and molecular aspects of the invasion mechanisms of pathogens (Glorieux et al., Citation2009; Vandekerckhove et al., Citation2010; Glorieux et al., Citation2011; Steukers et al., Citation2012). The approach allows a thorough comparison of different alphaherpesvirus replication kinetics in their respective species. The ILTV-induced plaques were present in the epithelial layer starting from 24 h p.i. The plaque latitude increased in time and the ILTV-infected plaques started to cross the basement membrane from 48 h p.i.
Mucosa explant models of porcine, equine, human and bovine origin were developed in our laboratory to study virus–host interactions and mucosal invasion. By using these explants, invasion processes of different alphaherpesviruses (EHV1, EHV4, BHV1, SHV1 and HSV1) have already been described. Based on their invasion mechanism, alphaherpesviruses can be classified into three types: EHV4 shows replication in the respiratory epithelial cells without invasion through the BM; BHV1, SHV1 and HSV1 exhibit a plaque-wise spread through the BM in the in vitro explant model; and EHV1 spreads only laterally and does not breach the BM (similar to EHV4), but invades deeper tissues of the respiratory tract via infected leukocytes that function as Trojan horses (Glorieux et al., Citation2009; Vandekerckhove et al., Citation2010, Citation2011; Glorieux et al., Citation2011; Steukers et al., Citation2012).
When comparing the replication kinetics of ILTV in the trachea and conjunctiva, we observed some interesting findings. At 72 h p.i., the plaque latitude in the conjunctival mucosa was significantly larger compared with that in the tracheal mucosa. The percentage of the plaques that penetrated through the BM was larger in the conjunctiva (48 h p.i., 43%; and 72 h p.i., 74%) compared with the trachea (48 h p.i., 31%; and 72 h p.i., 56%). Plaque latitude and invasion depth kinetics indicated that at later time points (48 and 72 h p.i.) ILTV showed a more extensive replication in the conjunctival mucosa compared with the tracheal mucosa. The differences in the replication kinetics of ILTV are probably due to variation in its tropism for the trachea and conjunctiva, an observation reported previously by Kirkpatrick et al. (Citation2006). In the future, a study of the replication characteristics of different ILTV strains on mucosal explants may help to understand their tropism for the trachea and conjunctiva.
In BHV1, SHV1 and HSV1 infection, the lateral mucosal spread and vertical invasion depth evolved similarly with increasing time p.i. In the case of ILTV, the invasion through the BM was very restricted. At 48 h p.i., 30.9% of the plaques in the trachea and 43.3% of the plaques in the conjunctiva were crossing the BM. This finding is in contrast to plaques induced by SHV1, HSV1 and BHV1. With SHV1 and HSV1, 100% of the plaques crossed the BM at 24 h p.i. (Glorieux et al., Citation2009, Citation2011). With BHV1, 90% of the plaques went through the BM at 48 h p.i. (Steukers et al., Citation2011). ILTV has possibly not developed enough tools to breach the BM quickly, unlike BHV1, SHV1 and HSV1. The restricted ILTV invasion through the BM agrees with the in vivo pathogenesis of ILTV, where no clear evidence exists for viraemia (Bagust, Citation1986; Guy & Bagust, Citation2003). Likewise, invasion of ILTV through the BM of the trachea and conjunctiva clearly confirms that ILTV is able to breach the BM. Indeed, Zhao et al. (Citation2013) detected ILTV in most of the internal organs of infected chickens.
Although speculative, a hypothesis can be formed to explain the restricted ILTV invasion through the BM in chickens compared with the invasion of other alphaherpesviruses in their respective species. Viral structural components and BM composition should be considered. The structural glycoproteins present on the surface of alphaherpesviruses and alphaherpesvirus-infected cells, together with up-regulated cellular proteases, are putative candidates for helping the virus to cross the BM. Several proteolytic enzymes, including trypsin-like proteases, have been shown to break down the BM and extracellular matrix, and trypsin-like serine protease was found to be involved in SHV1 invasion through the BM (Barrett et al., Citation2003; Glorieux et al., Citation2011). A rapid breakdown of BM components is most probably facilitating SHV1 invasion. Alphaherpesviruses use the glycoprotein E/glycoprotein I (gE/gI) complex to spread directly from cell to cell through cell junctions (Devlin et al., Citation2006). In the case of BHV1, the gE/gI complex also efficiently mediates invasion through the BM (Steukers, Citation2013). The involvement of trypsin-like serine proteases and/or gE/gI complex in ILTV invasion needs to be addressed. The characteristics of the BM may also play a role in the restricted ILTV invasion. The heterogeneous components in the BM interact and form a sheet of fibres. These intermolecular interactions in the BM might be stronger in chickens compared with mammals and prevent the entry of pathogens more effectively (Mayne & Zettergren, Citation1980; Fitch et al., Citation1982; Mayne et al., Citation1982; LeBleu et al., Citation2007). Several questions regarding why ILTV has difficulty in crossing the BM are still not answered. Do certain components or the total thickness of the BM play a role in its barrier function against ILTV invasion (Steukers et al., Citation2012); or did ILTV not develop a mechanism during evolution to enable it to invade quickly through the BM? TOC and COC explants are ideal tools to address these questions.
The effect of ILTV on cell viability of the tracheal and conjunctival mucosa was studied using a TUNEL assay. A large number of epithelial cells were ILTV-positive, but only a few ILTV-infected cells were TUNEL-positive, even at 72 h p.i. Our studies are consistent with previous reports demonstrating that low levels of apoptosis were observed in cells infected with other alphaherpesviruses, HSV2, SHV1 and HSV1 (Asano et al., Citation1999; Alemañ et al., Citation2001; Glorieux et al., Citation2011). Furthermore, TUNEL-positive cells were predominantly restricted to the vicinity of ILTV-infected cells. These TUNEL-positive cells might be local leukocytes that respond to the productive ILTV infection, as seen with SHV1 and HSV2 (Asano et al., Citation1999; Alemañ et al., Citation2001), or the apoptotic cells may be uninfected bystander cells present in the vicinity of infected cells (Alemañ et al., Citation2001). ILTV-infected cells may release some pro-apoptotic components or cytokines to induce apoptosis. This could be an ILTV strategy to sustain infection in the mucosa. Several publications have shown that alphaherpesviruses (HSV1, HSV2, SHV1 and BHV1) evade the immune system by inhibiting apoptosis of infected cells (Galvan & Roizman, Citation1998; Asano et al., Citation1999; Jerome et al., Citation1999; Winkler et al., Citation1999; Alemañ et al., Citation2001). More specifically, alphaherpesviruses usually evade natural killer cell-dependent and T-lymphocyte-dependent antiviral pathways and maintain the viability of infected cells (Winkler et al., Citation1999; Favoreel et al., Citation2000; Alemañ et al., Citation2001; Orange et al., Citation2002; Han et al., Citation2007; Murugin et al., Citation2011). There are reports that ILTV suppresses apoptosis of infected primary cells (Burnside & Morgan, Citation2011; Lee et al., Citation2012). However, to our knowledge there are no reports on the effect of ILTV on apoptosis in infected cells of the tracheal and conjunctival mucosae. Our current in vitro study therefore indicates that ILTV blocks apoptosis in these cells. Furthermore, it may induce apoptosis of neighbouring uninfected cells. Usually viruses alter host immune responses to their benefit. This may protect infected cells against an attack by the immune system, allowing infected epithelial cells to survive long enough to support replication. Furthermore, surviving epithelial cells may ensure that virus can breach the BM, reach the lamina propria and finally enhance mucosal invasion in the infection process. By doing this, ILTV may easily reach sensory nerves and the trigeminal ganglion, the site of latency. All of these mechanisms help ILTV to sustain life-long infection. The exact viral factors involved in the inhibition of apoptosis of infected cells and the activation of apoptosis in the surrounding cells will be identified in the near future.
Acknowledgements
The authors acknowledge Magda De Keyzer and Lieve Sys for their excellent technical assistance, Thierry van den Berg for providing the virus, and Zeger Van den Abeele, Loes Geypen and Bart Ellebaut for their help with the handling and humane killing of the chickens.
Funding
This research was supported by the Indian Council of Agricultural Research under International fellowship (ICAR, Pusa, New Delhi-110012 [29-1/2009-EQR/Edn]) and Ghent University (Concerted Research Action 01G01311). Vishwanatha R. A. P. Reddy, Alain Vanderplasschen and Hans J. Nauwynck are members of the BELVIR consortium (IAP, phase VII) sponsored by the Belgian Science Policy Office (BELSPO).
Additional information
Funding
References
- Alemañ, N., Quiroga, M.I., López-Peña, M., Vázquez, S., Guerrero, F.H. & Nieto, J.M. (2001). Induction and inhibition of apoptosis by pseudorabies virus in the trigeminal ganglion during acute infection of swine. Journal of Virology, 75, 469–479. 10.1128/JVI.75.1.469-479.2001
- Anderton, T.L., Maskell, D.J. & Preston, A. (2004). Ciliostasis is a key early event during colonization of canine tracheal tissue by Bordetella bronchiseptica. Microbiology, 2843–2855. 10.1099/mic.0.27283-0
- Asano, S., Honda, T., Goshima, F., Watanabe, D., Miyake, Y., Sugiura, Y. & Nishiyama, Y. (1999). US3 protein kinase of herpes simplex virus type 2 plays a role in protecting corneal epithelial cells from apoptosis in infected mice. Journal of General Virology, 80, 51–56.
- Bagust, T.J. (1986). Laryngotracheitis (gallid-1) herpesvirus infection in the chicken 4. Latency establishment by wild and vaccine strains of ILT virus. Avian Pathology, 15, 581–595. 10.1080/03079458608436317
- Bang, B.G. & Bang, F.B. (1967). Laryngotracheitis virus in chickens a model for study of acute nonfatal desquamating rhinitis. Journal of Experimental Medicine, 125, 409–428. 10.1084/jem.125.3.409
- Barrett, A.J., Tolle, D.P. & Rawlings, N.D. (2003). Managing peptidases in the genomic era. Biological Chemistry, 384, 873–882. 10.1515/BC.2003.098
- Burnside, J. & Morgan, R. (2011). Emerging roles of chicken and viral microRNAs in avian disease. BMC Proceedings, 5, S2.10.1186/1753-6561-5-S4-S2
- Darbyshire, J.H., Cook, J.K.A. & Peters, R.W. (1976). Organ culture studies on the efficiency of infection of chicken tissues with avian infectious bronchitis virus. British Journal of Experimental Pathology, 57, 443–454.
- Davison, A.J. (2010). Herpesvirus systematics. Veterinary Microbiology, 143, 52–69. 10.1016/j.vetmic.2010.02.014
- Devlin, J.M., Browning, G.F. & Gilkerson, J.R. (2006). A glycoprotein I- and glycoprotein E-deficient mutant of infectious laryngotracheitis virus exhibits impaired cell-to-cell spread in cultured cells. Archives of Virology, 151, 1281–1289. 10.1007/s00705-005-0721-8
- Favoreel, H.W., Nauwynck, H.J. & Pensaert, M.B. (2000). Immunological hiding of herpesvirus-infected cells. Archives of Virology, 145, 1269–1290. 10.1007/s007050070090
- Fitch, J.M., Gibney, E., Sanderson, R.D., Mayne, R. & Linsenmayer, T.F. (1982). Domain and basement membrane specificity of a monoclonal antibody against chicken type IV collagen. Journal of Cell Biology, 95, 641–647. 10.1083/jcb.95.2.641
- Freshney, R.I. (2005). In Chapter 3: Culture of Animal Cells: A Manual of Basic Technique (5th edn., pp. 31–42). Hoboken: Wiley-Blackwell.
- Fuchs, W., Veits, J., Helferich, D., Granzow, H., Teifke, T.P. & Mettenleiter, T.C. (2007). Molecular biology of avian infectious laryngotracheitis virus. Veterinary Research, 38, 261–279. 10.1051/vetres:200657
- Galvan, V. & Roizman, B. (1998). Herpes simplex virus 1 induces and blocks apoptosis at multiple steps during infection and protects cells from exogenous inducers in a cell-type-dependent manner. Proceedings of the National Academy of Sciences, 95, 3931–3936. 10.1073/pnas.95.7.3931
- Glorieux, S., Van den Broeck, W., Van der Meulen, K.M., VanReeth, K., Favoreel, H.W. & Nauwynck, H.J. (2007). In vitro culture of porcine respiratory nasal mucosa explants for studying the interaction of porcine viruses with the respiratory tract. Journal of Virological Methods, 142, 105–112. 10.1016/j.jviromet.2007.01.018
- Glorieux, S., Favoreel, H.W., Meesen, G., de Vos, W., Van den Broeck, W. & Nauwynck, H.J. (2009). Different replication characteristics of historical pseudorabies virus strains in porcine respiratory nasal mucosa explants. Veterinary Microbiology, 136, 341–346. 10.1016/j.vetmic.2008.11.005
- Glorieux, S., Bachert, C., Favoreel, H.W., Vandekerckhove, A.P., Steukers, L., Rekecki, A., Van den Broeck, W., Goossens, J., Croubels, S., Clayton, R.F. & Nauwynck, H.J. (2011). Herpes simplex virus type 1 penetrates the basement membrane in human nasal respiratory mucosa. PLoS One, 6, e22160.10.1371/journal.pone.0022160
- Guy, J.S. & Bagust, T.J. (2003). Laryngotracheitis. In Y.M. Saif, H.J. Barnes, J.R. Glisson, A.M. Fadly, L.R. McDougald, & D.E. Swayne (Eds.). Diseases of Poultry (11th edn., pp. 121–134). Ames: Blackwell.
- Han, J.Y., Sloan, D.D., Aubert, M., Miller, S.A., Dang, C.H. & Jerome, K.R. (2007). Apoptosis and antigen receptor function in T and B cells following exposure to herpes simplex virus. Virology, 359, 253–263. 10.1016/j.virol.2006.09.038
- Ide, P.R. (1978). Sensitivity and specificity of the fluorescent antibody technique for detection of infectious laryngotracheitis virus. Canadian Journal of Comparative Medicine, 42, 54–62.
- Jerome, K.R., Fox, R., Chen, Z., Sears, A.E., Lee, H.Y. & Corey, L. (1999). Herpes simplex virus inhibits apoptosis through the action of two genes, Us5 and Us3. Journal of Virology, 73, 8950–8957.
- Jones, B.V. & Hennion, R.M. (2008). The preparation of chicken tracheal organ cultures for virus isolation, propagation and titration. Methods in Molecular Biology, 454, 103–107.
- Jones, R.C. (2010). Viral respiratory diseases (ILT, aMPV infections, IB): are they ever under control? British Poultry Science, 51, 1–11. 10.1080/00071660903541378
- Kirkpatrick, N.C., Mahmoudian, A., Colson, C.A., Devlin, J.M. & Noormohammadi, A.H. (2006). Relationship between mortality, clinical signs and tracheal pathology in infectious laryngotracheitis. Avian Pathology, 35, 449–453. 10.1080/03079450601028803
- LeBleu, V.S., Macdonald, B. & Kalluri, R. (2007). Structure and function of basement membrane. Experimental Biology and Medicine, 232, 1121–1129. 10.3181/0703-MR-72
- Lee, J., Bottje, W.G. & Kong, B.W. (2012). Genome-wide host responses against infectious laryngotracheitis virus vaccine infection in chicken embryo lung cells. BMC Genomics, 13, 143.10.1186/1471-2164-13-143
- Mayne, R. & Zettergren, J.G. (1980). Type IV collagen from chicken muscular tissues. Isolation and characterization of the pepsin-resistant fragments. Biochemistry, 19, 4065–4072. 10.1021/bi00558a025
- Mayne, R., Wiedemann, H., Dessau, W., Von der Mark, K. & Bruckner, P. (1982) Structural and immunological characterization of type IV collagen isolated from chicken tissues. European Journal of Biochemistry, 126, 417–423. 10.1111/j.1432-1033.1982.tb06796.x
- Meulemans, G. & Halen, P. (1978). Some physico-chemical and biological properties of a Belgian strain (u76/1035) of infectious laryngotracheitis virus. Avian Pathology, 7, 311–315. 10.1080/03079457808418283
- Murugin, V.V., Zuikova, I.N., Murugina, N.E., Shulzhenko, A.E., Pinegin, B.V. & Pashenkov, M.V. (2011). Reduced degranulation of NK cells in patients with frequently recurring herpes. Clinical and Vaccine Immunology, 18, 1410–1415. 10.1128/CVI.05084-11
- Orange, J.S., Fassett, M.S., Koopman, L.A., Boyson, J.E. & Strominger, J.L. (2002). Viral evasion of natural killer cells. Nature Immunology, 11, 1006–1012. 10.1038/ni1102-1006
- Reemers, S.S., Groot Koerkamp, M.J., Holstege, F.C., Van Eden, W. & Vervelde, L. (2009). Cellular host transcriptional responses to influenza A virus in chicken tracheal organ cultures differ from responses in in vivo infected trachea. Veterinary Immunology and Immunopathology, 132, 91–100. 10.1016/j.vetimm.2009.04.021
- Russell, W.M.S. & Burch, R.L. (1959). The Principles of Humane Experimental Technique. London: Methuen & Co. Ltd.
- Steukers, L., Vandekerckhove, A.P., Van den Broeck, W., Glorieux, S. & Nauwynck, H.J. (2011). Comparative analysis of replication characteristics of BoHV-1 subtypes in bovine respiratory and genital mucosa explants: a phylogenetic enlightenment. Veterinary Research, 42, 33.10.1186/1297-9716-42-33
- Steukers, L., Vandekerckhove, A.P., Van den Broeck, W., Glorieux, S. & Nauwynck, H.J. (2012). Kinetics of BoHV-1 dissemination in an in vitro culture of bovine upper respiratory tract mucosa explants. Institute for Laboratory Animal Research Journal, 53, E43–E54. 10.1093/ilar.53.1.43
- Steukers, L. (2013). Unraveling Herpesvirus Mucosal Invasion in an Ex Vivo Organ Culture ( Doctoral dissertation). Ghent University, Faculty of Veterinary Medicine, Merelbeke.
- Tully, T.N. Jr. (1995). Avian respiratory diseases: clinical overview. Journal of Avian Medicine and Surgery, 9, 162–174.
- Vandekerckhove, A., Glorieux, S., Broeck, W.V., Gryspeerdt, A., van der Meulen, K. & Nauwynck, H.J. (2009). In vitro culture of equine respiratory mucosa explants. Veterinary Journal, 181, 280–287. 10.1016/j.tvjl.2008.03.027
- Vandekerckhove, A.P., Glorieux, S., Gryspeerdt, A.C., Steukers, L., Duchateau, L., Osterrieder, N., Van de Walle, G.R. & Nauwynck, H.J. (2010). Replication kinetics of neurovirulent versus non-neurovirulent equine herpesvirus type 1 strains in equine nasal mucosal explants. Journal of General Virology, 91, 2019–2028. 10.1099/vir.0.019257-0
- Vandekerckhove, A.P., Glorieux, S., Gryspeerdt, A.C., Steukers, L., Van Doorsselaere, J., Osterrieder, N., Van de Walle, G.R. & Nauwynck, H.J. (2011). Equine alphaherpesviruses (EHV-1 and EHV-4) differ in their efficiency to infect mononuclear cells during early steps of infection in nasal mucosal explants. Veterinary Microbiology, 152, 21–28. 10.1016/j.vetmic.2011.03.038
- Winkler, M.T., Doster, A. & Jones, C. (1999). Bovine herpesvirus 1 can infect CD4(+) T lymphocytes and induce programmed cell death during acute infection of cattle. Journal of Virology, 73, 8657–8668.
- Zhang, S., Jian, F., Zhao, G., Huang, L., Zhang, L., Ning, C., Wang, R., Qi, M. & Xiao, L. (2012). Chick embryo tracheal organ: A new and effective in vitro culture model for Cryptosporidium baileyi. Veterinary Parasitology, 188, 376–381. 10.1016/j.vetpar.2012.03.049
- Zhao, Y., Kong, C., Cui, X., Cui, H., Shi, X., Zhang, X., Hu, S., Hao, L. & Wang, Y. (2013). Detection of infectious laryngotracheitis virus by real-time PCR in naturally and experimentally infected chickens. PLoS One, 8, e67598.10.1371/journal.pone.0067598