Abstract
Marek's disease virus (MDV; also known as Gallid herpesvirus 2, MDV-1) causes oncogenic disease in chickens producing clinical signs that include lymphomas, visceral tumours, nerve lesions, and immunosuppression. MDV vaccines are widely used and mostly produced using primary cells: chicken embryo fibroblast cells, duck embryo fibroblast cells, chicken embryo kidney cells or chicken kidney cells. An immortalized cell line that can be used to manufacture the virus has long been desired. In this report, we demonstrate that QM7 cells were susceptible to infection with either MDV or herpesvirus of turkey (HVT; also known as Meleagrid herpesvirus 1, MDV-3). Polymerase chain reaction analysis with primers amplifying selected MDV genes revealed that QM7 cells did not contain these sequences. However, MDV genes were detected in QT35 cells, which have been reported to harbour latent MDV virus. Transfection of naked MDV DNA initiated efficient infection of QM7 cells. In addition, QM7 cell lysate, clarified supernatant, and QM7 cell pellet infected with MDV were negative for reverse transcriptase activity, indicating absence of endogenous retrovirus. QM7 cells were also found to be free of other avian pathogens in a chick embryo inoculation test. In vivo studies of MDV growing in QM7 cells showed the virus retained its pathogenicity and virulence. In ovo experiments demonstrated that both HVT and MDV propagated in QM7 cells did not interfere with hatchability of injected eggs, and viruses could be re-isolated from hatched chicks. The results suggest that QM7 could be a good host cell line for growing both MDV and HVT.
Introduction
Marek's disease in chickens is a common cause of death and condemnation in broilers. It is an acutely oncogenic disease, which causes lymphomas, visceral tumours, nerve lesions, and immunosuppression. The aetiologic agent, Marek's disease virus (MDV), is a member of the family Herpesviridae, subfamily of Alphaherpesvirus, genus Mardivirus. MDV (also named Gallid herpesvirus 2, MDV-1) includes all pathogenic strains and their attenuated derivatives. A close relative, Gallid herpesvirus 3 (MDV-2) consists of non-oncogenic chicken viruses. A third relative, herpesvirus of turkeys (HVT; also named Meleagrid herpesvirus 1, MDV-3) includes avirulent turkey viruses that are capable of replication in chickens (Cho, Citation1981; Osterrieder et al., Citation2006; Davison, Citation2010; Parvizi et al., Citation2010; Nair, Citation2013; Zelník et al., Citation2013).
The ability to grow large quantities of MDV for vaccine production has been hampered by the need for primary cell cultures. In addition to chicken embryo fibroblast (CEF) cells, other primary avian cells that have been used to grow MDV include duck embryo fibroblast (DEF) cells, chicken kidney cells and chicken embryo kidney cells. QT35, a chemically transformed quail cell line that supports the growth of MDV-2 and HVT (Cho, Citation1981), was also used for vaccine production. However, virulent MDV was rescued from HVT-infected QT35 cells due to the presence of latent MDV genome in the QT35 cells (Yamaguchi et al., Citation2000; Majerciak et al., Citation2001). A chemically transformed CEF cell line, CHCC-OU2, was reported to have the ability to be latently infected with MDV (Ogura et al., Citation1984; Ogura & Fujiwara, Citation1987; Abujoub & Coussens, Citation1995, Citation1997). However, long periods of MDV adaptation to the cell culture were necessary, and changes in genetics or antigenicity could occur during the adaptation period. Similarly, a continuous Vero cell line was reported to produce low titres of MDV virus after long adaptation (Jaikumar et al., Citation2001). A spontaneously immortalized CEF cell line, DF-1 (Himly et al., Citation1998; Schaefer-Klein et al., Citation1998), was shown to support growth of virulent MDV (Niikura et al., Citation2011).
QM7 cells are quail muscle myoblasts, derived from the QT6 fibrosarcoma cell line (Moscovici et al., Citation1977; Antin & Ordahl, Citation1991). However, QM7 cells seem to be significantly different from QT6 and QT35 fibrosarcoma cell lines. The cell morphology and growth characteristics of QM7 are very distinct from those of QT6 and QT35 cells, according to the reports above. QT35 cells were also previously shown to be resistant to MDV infection (Cho, Citation1981), consistent with subsequently finding the presence of latent MDV genome in these cells (Yamaguchi et al., Citation2000; Majerciak et al., Citation2001). Schumacher et al. (Citation2000, Citation2001, Citation2002) reported that constitutive expression of MDV gE protein in QM7 cells was required to support sufficient growth of MDV. We investigated the susceptibility of QM7 cells to infection with both MDV and HVT. We report efficient infection of QM7 cells with both MDV and HVT when compared with DEF or CEF cells. Both viruses grow in QM7 cells with high titres that were comparable with those of virus grown in DEF. Transfection of naked MDV DNA initiated efficient infection of QM7 cells. We also found that QM7 cells were negative for MDV genome by polymerase chain reaction (PCR).
Materials and Methods
Cells and viruses
QM7 and QT6 cells were obtained from the American Type Culture Collection (CRL-1962 and CRL-1708; ATCC, Rockville, MD, USA). QT35 cells were obtained from Amy Brown (Poultry Biologicals, Zoetis-VMRD, Kalamazoo, MI, USA). MDV-1 very virulent plus isolates 652 and 584A were obtained from Dr Dick Witter, USDA-Avian Disease and Oncology Lab, East Lansing, MI, USA (Witter, Citation1997). HVT vaccine (MD-Vac; Zoetis, formerly Fort Dodge Animal Health, Fort Dodge, IA, USA) was purchased. Infected and uninfected cells were grown at 37oC and 5% carbon dioxide in humidified incubators in Dulbecco's modified Eagle's medium (DMEM)/F-12 (Invitrogen, Life Technologies, Grand Island, NY, USA) supplemented with antibiotics and the appropriate amount of foetal bovine serum (FBS; 3% for infected cells and 10% for uninfected cells). Titration of virus was determined by calculating plaque-forming units (PFU) per 2 × 106 cells. The visible viral plaque foci were confirmed by immunofluoresence staining with specific antibodies.
Transfection of cells
Transfection of cells was completed according to the instructions included with the CaPO4 transfection Kit (ClonTech, Mountain View, CA, USA).
Extraneous avian pathogen test
Detection of extraneous avian pathogens was performed by SPAFAS Inc. (Preston, CT, USA). In the test, 0.1 ml QM7 cell suspension was inoculated into chick embryo (embryonic days 7 to 11, 20 embryos total) on the chorioallantoic membrane and an additional 0.1 ml in the allantoic sac. Embryo viability was monitored by candling the eggs daily for 7 days. The presence of any extraneous avian virus would lead to the death or abnormality of the chick embryo.
Immunofluoresence assay
QM7, DEF or CEF cells were infected with MDV strain 652 or HVT vaccine and incubated for 7 days in DMEM/F12 with 3% FBS. The infected cells were then fixed with 80% acetone and stained with a mixture of monoclonal antibodies H19 (anti-pp38 of MDV) and 1AN86 (anti-gB of both MDV and HVT) to detect MDV infection and with L78 only for detecting HVT infection. The monoclonal antibodies were gifts from Dr Lucy Lee, USDA-Avian Disease and Oncology Lab, East Lansing, MI, USA (Lee et al., Citation1983).
In vivo pathogenicity test
QM7 cells infected with MDV 652 were tested for their ability to cause Marek's disease in chickens. Seven groups of 30 specific pathogen free (SPF) chicks at 1 day old were inoculated intraperitoneally either with uninfected QM7 plus DEF cells, with QM7 cells infected with MDV 652 at three different doses (12.5, 125 and 1250 PFU), or with DEF cells infected with MDV 652 at three different doses (20, 200 and 2000 PFU). Birds were observed for MDV-related key clinical signs including wing and leg paralysis. Birds that died during the study were necropsied and examined for signs of MDV infection, including microscopic nerve lesions and nodules on the internal organs (Sharma et al., Citation1973; Calnek et al., Citation1989). The kidney, spleen, liver and brain were harvested at day 14 post infection for histological evaluation from surviving birds. All procedures in the study were approved and conducted in compliance with the Animal Welfare Act Regulations (9CFR Parts 1, 2 and 3) and the “Guide for the Care and Use of Laboratory Animals”, as well as with all internal bioethical guideline and policies.
In ovo experiments
Hubbard-Peterson broiler chicken eggs were obtained from a commercial supplier and incubated in Jamesway AVN setters. Non-viable embryos were detected by candling at 10 days of incubation and removed. On day 18 of incubation, approximately 70 embryonated eggs per treatment group were injected with 100 µl, containing 5000 PFU of either MDV (652) or HVT grown in QM7 or DEF cells. At hatch, chicks were separated by treatment and placed in isolator cages with 30 to 40 chicks per isolator. On days 3, 6, and 13 post hatch, blood was collected from 10 birds in each group, following which birds were humanely euthanized and spleens aseptically removed and placed in DMEM with antibiotic. On day 13, all remaining birds were euthanized and examined for lesions characteristic of Marek's disease as described previously. All bird procedures were approved by the Institutional Animal Care and Use Committee.
The ability of 652 viruses to amplify in the hatched chicks after in ovo inoculation was tested as follows. Spleen cells and peripheral blood mononuclear cells (PBMCs; buffy coat of blood) were isolated from chicks at days 3, 6 and 13 post hatch, and plated onto DEF cells. One week later, flasks were split 1:5 and cultured for 4 days prior to indirect immunofluorescent antibody staining for detection of virus.
Polymerase chain reaction
PCR primer sequences were as described in Yamaguchi et al. (Citation2000) for the following genes: ICP4, LAT, ICP22, ICP27, meq, pp38, VP16, ORF L1, and gB. The primers for GAPDH are 5′-GGATTTGGCCGTATTGG-3′ (upper primer) and 5′-GATGATGTTCTGGGCAGCACCTCT-3′ (lower primer). The PCR was carried out with 2 × JumpStart RED Taq (catalogue number 90,982; Sigma-Aldrich, St. Louis, MO, USA)] with the following conditions: denaturation at 94°C for 2 min, followed by 35 cycles of denaturation at 94°C for 15 sec, annealing at 55°C for 30 sec, and extension at 68°C for 1 min. Another extension was then done at 68°C for 7 min. The amplified fragments were resolved by electrophoresis in 2% agarose E-Gels (Invitrogen).
Reverse transcriptase assay
The presence of reverse transcriptase was detected using the Non-Radioactive Reverse Transcriptase Assay Kit (Boehringer Mannhein, Ingelhein am Rhein, Germany).
Results
MDV and HVT infection of QM7 is similar to infection of DEF or CEF cells
DEF and chicken kidney cells were reported to be optimal to grow MDV (Sharma, Citation1998; Schat, Citation2005). We therefore prepared our MDV (652) stock in DEF cells. QM7 cells were infected with 652/DEF at cell culture passage 11. Productive infection of the 652 isolate was monitored by quantifying formation of cytopathic effect (CPE) foci () and by detecting MDV viral protein (gB and pp38) expression using immunofluoresence (). Large and intensely stained infected foci were detected. The infection was maintained by inoculating 652/QM7 infected cells into seeded (50 to 60% confluence) uninfected QM7 cells. The QT6 fibrosarcoma cell line was also tested for its ability to support a productive MDV infection. However, no apparent CPE was visible and immunofluoresence with MDV monoclonal antibodies did not show any specific staining (data not shown).
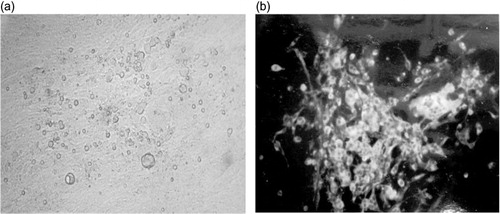
To further characterize and find the best conditions for MDV infection of QM7 cells, two serum concentrations (3% and 10%) and three culture split ratios (1:2, 1:5 and 1:10) were tested. Different split ratios were tested to assess the impact of cell density on the efficiency of infection. The number of PFU per 2 × 106 infected cells was calculated for each growth condition (). Higher titres were observed when serum concentration of 3% was used than with 10% serum. The splitting ratio of 1:5 and 1:10 resulted in higher titres than 1:2 ().
Table 1. Titration of viral titres for 652/QM7.
We also tested the ability of QM7 cells as indicator cells for 652 viral titration. A stock of 652/DEF was titrated on either DEF or QM7 cells at the same time. The titration plates were stained with monoclonal antibodies and PFU per 2 × 106 cells were determined. The titre on DEF cells was 2.2 × 105 PFU per 2 × 106 cells, while the titre on QM7 cells was 1.2 × 105 PFU per 2 × 106 cells. This result suggested that QM7 cells can be used as substrate cells for 652 titration.
As further comparisons, we titrated our two viral stocks, 652/DEF and 652/QM7 p6, in the same assay using DEF cells. The 652/DEF stock was found to have a titre of 1.3 × 106 PFU per 2 × 106 cells, and the 652/QM7 p6 stock had a titre of 3.2 × 106 PFU per 2 × 106 cells. We observed that the titre of 652/QM7 increased with each passage and peaked at passages 6 to 8. The viral stock prepared at passage 10 had lower viral titre when compared with passage 8 (data not shown). These results suggest that MDV replicates efficiently in the QM7 cells, and the titres seem comparable with DEF primary cells.
In one experiment, we infected QM7 with MDV 584A and the 584A/QM7 stock had a titre of 8 × 104 PFU per 2 × 106 cells.
To further confirm the ability of MDV to replicate in QM7 cells, DNA was isolated from 652/DEF-infected cells and transfected into uninfected QM7 cells. After 7 days of incubation, many infected foci () could be seen on the transfection plates. These foci were visually and immunohistochemically (, staining with monoclonal antibodies anti-pp38 and anti-gB) indistinguishable from the foci established by co-cultivation. These results rule out the possibility that the CPE and infection could be caused by contaminated cell inocula and demonstrate unequivocally that MDV can replicate and grow productively in QM7 cells.
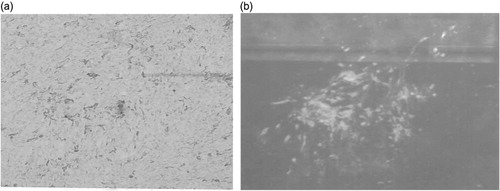
QM7 cells were also efficiently infected with HVT commercial vaccines. HVT/QM7 infected CPE foci were very large and clearly visible (), and confirmed by detecting HVT viral proteins gB expression using an immunofluorescence assay (). One stock of HVT/QM7 had titre of 2 × 105 PFU per 2 × 106 cells. In the same experiment, a HVT/CEF stock was determined to be 3 × 105 PFU per 2 × 106 cells.
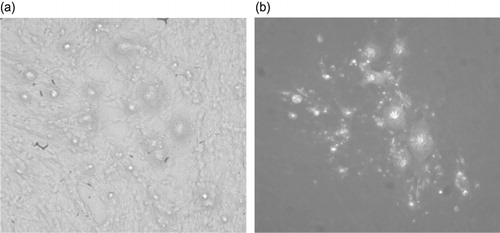
Absence of retrovirus and other avian pathogens in QM7 cells
QM7 cells were tested for reverse transcriptase activity. Cell lysate, clarified supernatant, and cell pellet were prepared from DEF, 652/DEF, QM7 and 652/QM7 and a positive control cell line, NYU-97 (a gift from Dr Thorbecke of New York University, USA). Ultracentrifuged pellet and cell lysates obtained from positive control cells produced strong positive results for reverse transcriptase activity. QM7, 652/QM7, DEF and 652/DEF cells were clearly negative for reverse transcriptase activity in this assay, which is sensitive to at least 20 pg. These results indicate that the presence of retroviral particles above the level of sensitivity in any of the four cell lines is unlikely.
QM7 cells were also tested for the presence of any avian pathogens that are lethal or cause abnormality of embryos. In the test, QM7 cell suspension was inoculated into chick embryo (embryonic days 9 to 11). At the end of the 7-day observation period, no death, discolouration or deformation of the chick embryos was observed, suggesting an absence of any extraneous avian virus that is lethal or causes abnormality of embryos in QM7 cells.
In vivo pathogenicity of 652/QM7
To test whether MDV 652 growing in QM7 cells retains its pathogenicity, 652/QM7-infected cells were tested for their ability to cause Marek's disease in chickens. Seven groups of 1-day-old SPF chicks were inoculated with uninfected cells, with 652/QM7 at 12.5, 125 and 1250 PFU, or with 652/DEF at 20, 200 and 2000 PFU. MDV lesions were found in birds only from the infected groups. No such lesions were seen in birds from the cell control groups (). In addition to isolate 652, another very virulent plus isolate, 584A, was also grown in QM7 cells and both 1500 and 150 PFU doses caused mortality and MDV lesions for 100% or 93.3% of birds (30 total), respectively. This in vivo experiment demonstrated that MDV (both 652 and 584A strains) grown in QM7 cells retains pathogenicity, and probably also antigenicity.
Table 2. Different doses of 652/QM7 and 652/DEF that cause in vivo pathogenicity.
In ovo test
All groups of eggs injected with virus had hatch rates within the normal range (≥85%). Indirect immunofluorescent antibody staining results () showed that virus could be re-isolated from both spleen and PBMCs on days 3 and 6 post hatch, suggesting amplification of the three virus stocks in the hatched chicks. In addition, Marek's lesions were observed for 652/QM7 and 652/DEF. Virus was not detected on day 13 in chicks receiving HVT, and no lesions were observed in these birds.
Table 3. Virus re-isolation and pathogenicity following in ovo injection.
Absence of MDV genome in QM7 cells
The potential presence of latent MDV genome in the QM7 cells was investigated. The QT35 fibrosarcoma cell line, which was generated the same way as QT6 from which QM7 was derived (Moscovici et al., Citation1977), was found to harbour the MDV genome, and virulent MDV virus could be rescued from QT35 cells with HVT infection (Yamaguchi et al., Citation2000; Majerciak et al., Citation2001). Various primers that amplify major MDV genes were used in PCR analysis for DNA isolated from QM7 cells, and DNA of QT35 and 652/DEF was used as positive control. The results suggest that while MDV DNA can be amplified from QT35 and 652/DEF for ICP27, ORFL1, VP16, LATs-2, ICP22 and Meq genes, no MDV DNA was amplified from QM7 for the above genes ().
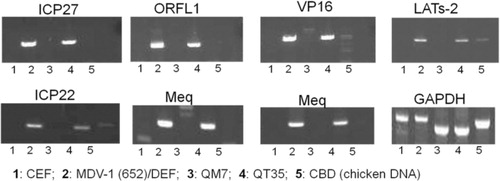
Discussion
This work demonstrated that QM7 cells can be infected with MDV (652), MDV (584A), and HVT without the constitutive expression of gE from QM7 cells. The viral titre for isolate 652, after a few passages and growth optimization, was comparable with the titre obtained from infecting DEF cells. This is different from a previous report that constitutive expression of gE was required for efficient growth of MDV in QM7 cells (Schumacher et al., Citation2002). We noted that culture and infection conditions, such as types of medium, serum concentration, and the split ratio, affect the viral production yield. The clonal variations of QM7 cells may also play important roles in the different infection efficiencies seen in this study and earlier studies. For example, we observed viral infection of cells grown with 10% FBS had a lower viral titre than cells grown with 3% serum concentration (). In addition, different strains or different sources of MDV used in the infection study might contribute to the differences as well. Schumacher et al. (Citation2001, Citation2002) used a reconstituted infectious BAC20 clone, 584Ap80C. We used strain 652 and 584A virus directly without any manipulation. It is conceivable that the 584Ap80C BAC clone behaved differently from the original 584A virus. It was reported previously that the BAC clone of MDV-2 vaccine pSB-1 had lower viral replication and shedding than the original SB-1 virus (Singh et al., Citation2010). The titre obtained with one stock of 652/QM7 (3.2 × 106 PFU per 2 × 106 cells, after a few passages and growth optimization) in this study seems comparable with the titre obtained by others with gE-transfected QM7 cells (1.8 × 106 PFU per 107 cells; Schumacher et al., Citation2002). The titre in QM7 cells without gE transfection was not reported by Schumacher et al. (Citation2002). Our one experiment with 584A/QM7 had a titre of 8 × 104 PFU per 2 × 106 cells. It should be noted that no growth optimization and passages were done with this strain as was done with isolate 652, and the titre may be higher afterwards. Whether additional expression of exogenous gE protein in QM7 cells can further enhance the infection efficiency in our conditions was not explored.
We observed that as few as 20 PFU MDV (652) were sufficient to induce pathogenicity in SPF chicks (). In the in ovo experiment, MDV 652 (5000 PFU) caused pathogenicity when examined at day 13 post hatch (). However, all groups of chicks were hatched normally, whether MDV 652 was present or not. The results suggested that the maternal antibodies present in the commercial broiler eggs that were used in the in ovo experiment might inhibit the 652 pathogenicity during hatching. It would be interesting to determine the hatch rate of SPF eggs inoculated with the same amount of MDV 652.
The QT35 fibrosarcoma cell line, which was generated the same way as QT6 from which QM7 was derived, was found to harbour the MDV genome, and virulent MDV virus could be rescued from QT35 cells with HVT infection (Yamaguchi et al., Citation2000; Majerciak et al., Citation2001). QM7 and QT35 DNA was carefully analysed with PCR primers that amplify major genes of the MDV genome. Although the selected MDV genes were not detected in QM7 DNA, QT35 DNA was consistently positive for these MDV genes. These results are in agreement with an earlier report that MDV genes were undetectable in QM7 cells in Southern blot analysis (Schumacher et al., Citation2000). In addition, QT35 cells were reported to be resistant to MDV infection (Cho, Citation1981). This is consistent with QT35 cells harbouring latent MDV genome. We show that QM7 cells were easily infected by MDV and produce productive infection. These results are consistent with the finding that QM7 cells do not harbour latent MDV genome.
QM7 cells were found to be free of exogenous avian viruses that are lethal or cause abnormality of embryos and possibly endogenous retrovirus due to an undetectable level of reverse transcriptase activities. Uninfected QM7 cells were found to be safe, at 13 to 14 days post hatch necropsy, in vivo or by intraperitoneal injection, oral application or in ovo application. Normal hatch rates were observed after in ovo inoculation. No disease was observed among inoculated chicks and no tumours were detected at 13 to 14 days post hatch necropsy. This is consistent with a previous observation that QM7 cells do not cause tumours in chickens following 12-week observation and post-mortem examination (Schumacher et al., Citation2002). Furthermore, MDV viruses growing in QM7 cells retain their pathogenicity. These results suggest QM7 cells are a useful tool to study MDV and HVT infection and might be used for MDV vaccine production.
Acknowledgements
The authors wish to thank Dr Pedro Villegas for helping with the in vivo pathogenicity studies. The authors also wish to thank Tracy Brown, Jenny Cai, Mary LeMay and Kay Genteman for technical help.
References
- Abujoub, A. & Coussens, P.M. (1995). Development of a sustainable chick cell line infected with Marek's disease virus. Virology, 214, 541–549. 10.1006/viro.1995.0065
- Abujoub, A. & Coussens, P.M. (1997). Evidence that Marek's disease virus exists in a latent state in a sustainable fibroblast cell line. Virology, 229, 309–321. 10.1006/viro.1997.8444
- Antin, P.B. & Ordahl, C.P. (1991). Isolation and characterization of an avian myogenic cell line. Developmental Biology, 143, 111–121. 10.1016/0012-1606(91)90058-B
- Calnek, B.W., Lucio, B., Schat, K.A. & Lillehoj, H.S. (1989). Pathogenesis of Marek's disease virus – induced local lesions. 1. Lesion characterization and cell line establishment. Avian Diseases, 33, 291–302. 10.2307/1590847
- Cho, B.R. (1981). A simple in vitro differentiation between turkey herpesvirus and Marek's disease virus. Avian Diseases, 25, 839–846. 10.2307/1590058
- Davison, A.J. (2010). Herpesvirus systematics. Veterinary Microbiology, 143, 52–69. 10.1016/j.vetmic.2010.02.014
- Himly, M., Foster, D.N., Bottoli, I., Iacovoni, J.S. & Vogt, P.K. (1998). The DF-1 chicken fibroblast cell line: transformation induced by diverse oncogenes and cell death resulting from infection by avian leukosis viruses. Virology, 248, 295–304. 10.1006/viro.1998.9290
- Jaikumar, D., Read, K.M. & Tannock, G.A. (2001). Adaptation of Marek's disease virus to the Vero continuous cell line. Veterinary Microbiology, 79, 75–82. 10.1016/S0378-1135(00)00346-1
- Lee, L.F., Liu, X. & Witter, R.L. (1983). Monoclonal antibodies with specificity for three different serotypes of Marek's disease viruses in chickens. Journal of Immunology, 130, 1003–1006.
- Majerciak, V., Valkova, A., Szabova, D., Geerligs, H. & Zelnik, V. (2001). Increased virulence of Marek's disease virus type 1 vaccine strain CV1988 after adaptation to QT35 cells. Acta Virologica, 45, 101–108.
- Moscovici, C., Moscovici, M.G., Jimenez, H., Lai, M.M.C., Hayman, M.J. & Vogt, P.K. (1977). Continuous tissue culture cell lines derived from chemically induced tumors of Japanese quail. Cell, 11, 95–103. 10.1016/0092-8674(77)90320-8
- Nair, V. (2013). Latency and tumorigenesis in Marek's disease. Avian Diseases, 57, 360–365. 10.1637/10470-121712-Reg.1
- Niikura, M., Kim, T., Silva, R.F., Dodgson, J. & Cheng, H.H. (2011).Virulent Marek's disease virus generated from infectious bacterial artificial chromosome clones with complete DNA sequence and the implication of viral genetic homogeneity in pathogenesis. Journal of General Virology, 92, 598–607. 10.1099/vir.0.026864-0
- Ogura, H. & Fujiwara, T. (1987). Establishment and characterization of a virus-free chick cell line. Acta Medica Okayama, 41, 141–143.
- Ogura, H., Fujiwara, T. & Namba, M. (1984). Establishment of two chick embryo fibroblastic cell lines. Gann, 75, 410–414.
- Osterrieder, N., Kamil, J.P., Schumacher, D., Tischer, B.K. & Trapp, S. (2006). Marek's disease virus: from miasma to model. Nature Reviews Microbiology, 4, 283–294. 10.1038/nrmicro1382
- Parvizi, P., Abdul-Careem, M.F., Haq, K., Thanthrige-Don, N., Schat, K.A. & Sharif, S. (2010). Immune responses against Marek's disease virus. Animal Health Research Reviews, 11, 123–134. 10.1017/S1466252310000022
- Schaefer-Klein, J., Givol, I., Barsov, E.V., Whitcomb, J.M., VanBrocklin, M., Foster, D.N., Federspiel, M.J. & Hughes, S.H. (1998). The EV-O-derived cell line DF-1 supports the efficient replication of avian leukosis-sarcoma viruses and vectors. Virology, 248, 305–311. 10.1006/viro.1998.9291
- Schat, K.A. (2005). Isolation of Marek's disease virus: revisited. Avian Pathology, 34, 91–95. 10.1080/03079450500059289
- Schumacher, D., Tischer, B.K., Fuchs, W. & Osterrieder, N. (2000). Reconstitution of Marek's disease virus serotype 1 (MDV-1) from DNA cloned as a bacterial artificial chromosome and characterization of a glycoprotein B-negative MDV-1 mutant. Journal of Virology, 74, 11088–11098. 10.1128/JVI.74.23.11088-11098.2000
- Schumacher, D., Tischer, B.K., Reddy, S.M. & Osterrieder, N. (2001). Glycoproteins E and I of Marek's disease virus serotype 1 are essential for virus growth in cultured cells. Journal of Virology, 75, 11307–11318. 10.1128/JVI.75.23.11307-11318.2001
- Schumacher, D., Tischer, B.K., Teifke, J.-P., Wink, K. & Osterrieder, N. (2002). Generation of a permanent cell line that supports efficient growth of Marek's disease virus (MDV) by constitutive expression of MDV glycoprotein E. Journal of General Virology, 83, 1987–1992.
- Sharma, J.M. (1998). Marek's Disease. In D.E. Swayne, J.R. Glisson, M.W. Jackwood, J.E. Pearson, & W.M. Reed (Eds.), A Laboratory Manual for the Isolation and Identification of Avian Pathogens 4th edn (pp.116–124). Kennett Square, PA: American Association of Avian Pathologists.
- Sharma, J.M., Witter, R.L. & Burmester, B.R. (1973). Pathogenesis of Marek's disease in old chickens: lesion regression as the basis for age-related resistance. Infection and Immunity, 8, 715–724.
- Singh, S.M., Baigent, S.J., Petherbridge, L.J., Smith, L.P. & Nair, V.K. (2010). Comparative efficacy of BAC-derived recombinant SB-1 vaccine and the parent wild type strain in preventing replication, shedding and disease induced by virulent Marek's disease virus. Research in Veterinary Science, 89, 140–145. 10.1016/j.rvsc.2010.01.010
- Witter, R.L. (1997). Increased virulence of Marek's disease virus field isolates. Avian Diseases, 41, 149–163. 10.2307/1592455
- Yamaguchi, T., Kaplan, S.L., Wakenell, P. & Schat, K.A. (2000). Transactivation of latent Marek's disease herpesvirus genes in QT35, a quail fibroblast cell line, by herpesvirus of turkeys. Journal of Virology, 74, 10176–10186. 10.1128/JVI.74.21.10176-10186.2000
- Zelník, V., Lapuníková, B. & Kúdelová, M. (2013). Marek's disease: rapid progress in research with unclear biological implementations. Acta Virologica, 57, 265–270.