Abstract
Muscovy duck parvovirus (MDPV) infection is a highly contagious and fatal disease of Muscovy ducklings. The infectious clone methodology is a valuable tool to study the pathogenic mechanisms of viruses, but no infectious clone of MDPV is yet available. In this study, a plasmid clone containing the full-length genome of MDPV was constructed using the TA cloning methodology. This MDPV clone was found to be infectious after transfection of primary Muscovy duck embryo fibroblast cells and passage in embryonated Muscovy duck eggs. Site-directed mutagenesis showed that the K75N mutation in the VP1 protein of MDPV resulted in the partial attenuation of the virus. The availability of an MDPV infectious clone can facilitate investigation of the pathogenic mechanisms of MDPV and development of vaccines against diseases caused by MDPV.
Introduction
Waterfowl parvovirus causes a disease of high mortality and morbidity in geese and ducks (Palya, Citation2013). Studies using sequencing analysis and virus neutralization tests show that waterfowl parvoviruses are divided into two groups: the goose parvovirus (GPV) group and the Muscovy duck parvovirus (MDPV) group (Le Gall-Reculé & Jestin, Citation1994; Zadori et al., Citation1994, Citation1995; Brown et al., Citation1995; Chang et al., Citation2000). GPV differs from MDPV in that GPV causes the disease in both geese and Muscovy ducks, whereas MDPV causes the disease in Muscovy ducks but not in geese (Glávits et al., Citation2005). Both GPV and MDPV contain a single-stranded DNA genome of approximately 5.1 kb in length (Zadori et al., Citation1995). This genome contains two major open reading frames, the first encoding the non-structural protein involved in viral replication and the second encoding three capsid proteins: VP1, VP2 and VP3. The three capsid proteins are derived from the same gene, and the entire amino acid sequences of VP2 and VP3 are contained within the C-terminal portion of the VP1 protein (Zadori et al., Citation1995).
Little is known about the genetic determinants of the virulence of GPV and MDPV. Shien et al. reported that amino acid sequences near the putative receptor binding site of the VP1 protein might be responsible for the attenuation of GPV (Shien et al., Citation2008). Tatár-Kis et al. reported the presence of a K75N mutation in the VP1 protein of European vaccine strains of GPV (Tatár-Kis et al., Citation2004). Wang et al. reported that a total of 11 amino acid substitutions were found in the VP1 protein of the Chinese vaccine strain of GPV (Wang et al., Citation2014). However, no site-directed mutagenesis data are yet available to verify whether any of the amino acid changes identified in these studies is responsible for the attenuation of GPV.
The infectious clone methodology is a useful tool to investigate the pathogenic mechanisms of viruses. To date, an infectious clone from GPV, but not MDPV, is available (Qiu et al., Citation2005). Further, the infectious clone of GPV was constructed by restriction enzyme digestion and ligation of the GPV DNA, which is time-consuming (Qiu et al., Citation2005; Wang et al., Citation2014). The TA cloning methodology provides a simple and efficient way for cloning DNA. This methodology takes advantage of the ligation between 3′-A overhangs of the insert DNA and 5′-T overhangs of the vector, and thus circumvents the need for restriction enzyme digestion (Holton & Graham, Citation1991). In this study, we report a method for constructing an infectious clone of MDPV by TA cloning. The cloned MDPV DNA was found to be infectious. Moreover, the VP1 gene of the MDPV clone was mutated to investigate the effect of the K75N mutation on the virulence of MDPV.
Materials and Methods
Virus propagation and DNA purification
MDPV field strain 90-215 (Chang et al., Citation2000) was used in this study. This strain was propagated in 11-day-old embryonated Muscovy duck eggs, which were collected from a flock that had no previous history of GPV or MDPV infections. Allantoic fluid from the infected eggs was harvested after the embryos died (normally 4–6 days post-inoculation). About 50 ml of MDPV containing allantoic fluid was extracted twice with an equal volume of chloroform. The upper aqueous phase containing the virus was collected and subjected to ultracentrifugation at 80,000 g for 2.5 h at 4°C. The virus-containing pellet was solubilized in 1 ml of phosphate-buffered saline (pH 7.0). The viral DNA in the solution was purified using the GeneJET Genomic DNA Purification Kit (Thermo Scientific, Waltham, MA, USA). The purified DNA was eluted with 100 μl of TE buffer (10 mM Tris, pH 8.0, 1 mM EDTA).
Construction of an infectious plasmid clone of MDPV by TA cloning
As MDPV is known to encapsidate both positive- and negative-sense DNA into the viron (Le Gall-Reculé & Jestin, Citation1994), the purified MDPV DNA was annealed to the double-stranded form via heating at 95°C for 5 min and 55°C for 1 h. The 3′-A overhangs were added to the annealed DNA using Taq DNA polymerase. The reaction mixture contained 16.6 μl of annealed viral DNA, 2 μl of 10× Taq buffer, 0.4 μl of dATP (10 mM) and 1 μl of Taq DNA polymerase. The reaction mixture was incubated at 72°C for 10 min. The viral DNA with 3′-A overhangs was ligated into the pGEM-T Easy vector using the TA cloning kit (Promega, Madison, WI, USA) according the manufacturer's instructions. Briefly, 3 μl of annealed viral DNA was mixed with 5 μl of 2× ligation buffer, 1 μl of pGEM-T vector (50 ng) and 1 μl of T4 DNA ligase. The ligation mixture was incubated at room temperature for 1 h, and the ligated DNA was transformed into the Escherichia coli SURE strain (Stratagene Corporation, La Jolla, CA, USA) by chemical transformation. Plasmids from the transformants were purified using the QIAprep Spin Miniprep kit (Qiagen, Hilden, Germany). The presence of MDPV DNA in the plasmid was verified by restriction enzyme digestion and sequencing analysis using pUC/M13 forward and reverse sequencing primers. The sequencing analysis was conducted using the ABI PRISM® dGTP BigDye™ Terminator v3.0 Ready Reaction Cycle Sequencing Kit (Applied Biosystems, Foster City, CA, USA) in an automatic sequencer (ABI-3730XL DNA Analyzer). The plasmid containing the full-length MDPV DNA was designated as pMDPV.
Construction of mutant clones
Two mutant clones were constructed in this study; the first contained a non-sense mutation of the tyrosine at position 12 (Y12X) of the VP1 protein, and the second contained a lysine to asparagine change at position 75 (K75N) of the VP1 protein. For site-directed mutagenesis, a 3.3-kb DNA fragment containing the VP1 sequence was PCR-amplified from pMDPV using primers MDPV1330(+) and MDPV4630(−) () with a high-fidelity Taq polymerase (SuperTaq XL, Bio-Genesis Technologies, Inc., Taipei, Taiwan). The 3.3-kb fragment was cloned into the pGEM-T Easy vector to construct the plasmid pGEM-VP1. The mutagenesis of the VP1 gene in pGEM-VP1 was performed using the QuikChange II Site-Directed Mutagenesis Kit (Agilent Technologies, Santa Clara, CA, USA) according to the manufacturer's instructions. Two primer pairs, VP1-Y12X(+)/VP1-Y12X(−) and VP1-K75N(+)/VP1-K75N(−) (), were designed to introduce mutations in residues 12 and 75 of the VP1 protein. Mutations were verified by sequencing analysis using primer MDPV2075(+) (). The 3.3-kb DNA fragment containing the mutated VP1 genes was released from pGEM-VP1 by digestion with AvaI and Acc65I. The released fragment was used to replace the corresponding fragment containing the wild-type sequence in plasmid pMDPV. The resulting mutant plasmid clones were designated as pMDPV-Y12X and pMDPV-K75N, respectively.
Table 1. Primers used for PCR amplification in this study.
Transfection of cells with MDPV plasmid
Primary Muscovy duck embryo fibroblast cells were cultured in a 25T flask (Thermo Scientific) and transfected with 2 μg of plasmid DNA using Lipofectamine® Transfection Reagent (Life Technologies, Carlsbad, CA, USA). The plasmid DNA was linearized with the restriction enzyme NotI before the transfection. Four days after transfection, the transfected cells were subjected to three freeze–thaw cycles to facilitate cell lysis. The rescued virus in the cell lysates was passaged four times in 11-day-old embryonated Muscovy duck eggs. The presence of rescued virus in the allantoic fluid of the eggs was verified by real-time PCR. The titre of the virus was determined using 11-day-old embryonated eggs and expressed as the medium embryo lethal dose (ELD50)/ml as previously described (Reed & Muench, Citation1938).
Real-time PCR
Two primers, designated as MDPV3062(+) and MDPV3230(−) (), were used for real-time PCR in this study. PCR with these two primers amplified a 169-bp fragment from the VP1 gene. The real-time PCR was conducted using the SYBR® Green PCR Reagents Kit (Life Technologies) and the reaction was performed using an Eco Real-Time PCR System (Illumina Inc., San Diego, CA, USA). The PCR conditions were one cycle of 95°C for 10 min, followed by 40 cycles of 95°C 10 s, 60°C for 30 s and 72°C for 30 s. The 169-bp PCR product was cloned into the pGEM-T Easy vector (Promega), and the resulting plasmid was used to establish the standard curve of the real-time PCR. The copy number of MDPV DNA was determined by the instrument software.
Virulence assays
The virulence of MDPV was determined using 11-day-old embryonated Muscovy duck eggs and one-day-old Muscovy ducklings. The eggs were inoculated with MDPV (109 copies per egg) by the allantoic cavity route, and the eggs were incubated at 37°C. The eggs were candled daily and the times of death of the embryos were recorded. Ducklings were infected by intraocular and intranasal inoculation with MDPV (109 or 108 copies per bird). The ducklings were examined twice daily for clinical signs and death. Birds deemed moribund were euthanized for welfare reasons. The animal experimental protocols were approved by the Institutional Animal Care and Use Committee of National Chung Hsing University.
Results
Extraction and the electrophoretic pattern of MDPV DNA
The purified MDPV DNA showed two major bands, with apparent sizes of 5.1 kb and 3.6 kb, in the agarose gel (). Because the size of the MDPV genome is about 5.1 kb (Zadori et al., Citation1995), the 5.1-kb band appeared to be the double-stranded form of the MDPV DNA. Because MDPV encapsidates both positive- and negative-sense DNA into the viron (Le Gall-Reculé & Jestin, Citation1994), the 3.6-kb band appeared to be the single-stranded form of the MDPV DNA. The 3.6-kb DNA could be annealed to the 5.1-kb DNA via heating at 95°C for 5 min and 55°C for 1 h ().
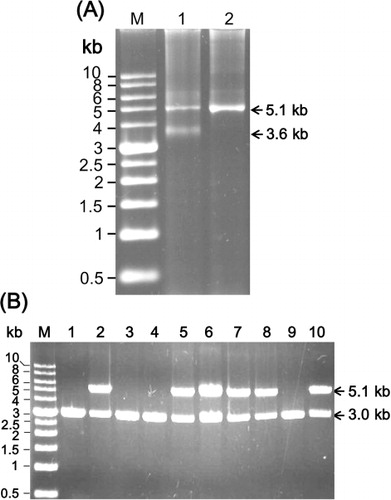
Construction of an infectious plasmid clone of MDPV by TA cloning
The 3′-A overhangs were added to the annealed MDPV DNA by Taq DNA polymerase. The resulting DNA was ligated into the pGEM-T Easy vector and the ligated products were transformed into E. coli. Plasmids were purified from the E. coli transformants and then digested with the restriction enzyme NotI, which cuts the sequences flanking the insertion site of the pGEM-T Easy vector. Digestion of the recombinant plasmid with NotI was expected to produce two DNA bands, one from the MDPV DNA (5.1 kb) and the other from the pGEM-T Easy vector (3.0 kb). As shown in , plasmids isolated from 60% of the tansformants contained insertions of the MDPV DNA. Sequencing analysis showed that the sequence of the 5′ and 3′ termini of MDPV DNA, as well as the entire coding region of non-structural and VP1 genes, was intact in the plasmid. These results showed that the full-length MDPV DNA was successfully cloned into the pGEM-T Easy vector. The plasmid clone containing the full-length MDPV genome was designated as pMDPV.
Construction of mutant clones
Two mutant clones of MDPV, designated as pMDPV-Y12X and pMDPV-K75N, were constructed using site-directed mutagenesis. Clone pMDPV-Y12X contained a non-sense mutation of the tyrosine at position 12 (Y12X) of the VP1 protein, while clone pMDPV-K75N contained a lysine to asparagine change at position 75 (K75N) of the VP1 protein. The Y12X mutation was expected to be lethal because the VP1 protein is essential for the replication of MDPV. The Y12X mutant was constructed to rule out the possibility that the rescued virus originated from contamination. The K75N mutant was constructed to investigate whether this mutation might affect the virulence of MDPV, as a previous study found the K75N mutation in attenuated strains of GPV (Tatár-Kis et al., Citation2004).
Rescue of MDPV from the cloned DNA
Plasmids containing the wild-type and mutant clones of MDPV were transfected into primary Muscovy duck embryo fibroblast cells, and the rescued viruses were passaged four times in embryonated Muscovy duck eggs. The presence of the rescued viruses in the allantoic fluids of the eggs was verified by real-time PCR. The results showed that the rescued wild-type virus had a copy number of 107.2–107.4 copies/ μl of allantoic fluid, whereas the K75N mutant had a copy number of 107.3–107.6 copies/ μl of allantoic fluid. The titres of the rescued wild-type virus and K75N mutant were 105.7 and 105.3 ELD50/ml, respectively. Sequencing analysis showed that the K75N mutation was present in the rescued K75N virus, but not in the wild-type virus. This result indicated that both the wild-type and K75N mutant viruses could be rescued from the plasmid clones, and the two rescued viruses grew to similar titres in embryonated eggs. In contrast, the rescued Y12X mutant virus showed a copy number below the detection limit of the real-time PCR (about 102 copies/ μl allantoic fluid), indicating that the Y12X mutation was lethal to MDPV. Moreover, this indicated that the viruses rescued from the wild-type and K75N mutant clones were unlikely to originate from contamination.
Virulence assays
The virulence of the rescued wild-type and K75N mutant viruses was determined by lethality assays. Two types of lethality assays were conducted in this study. The first type used 11-day-old embryonated Muscovy duck eggs; the challenge dose was 109 copies of MDPV per egg. The second type used one-day-old Muscovy ducklings; the challenge dose was 109 or 108 copies of MDPV per bird. The results of the first type of assay showed that the mean death time (MDT) values for embryonated eggs infected with the wild-type virus and K75N mutant were 4.85 and 6.85 days, respectively (). The results of the second type of assay showed that when the challenge dose was 109 copies of MDPV per bird, the MDT values for ducklings infected with the wild-type virus and K75N mutant were 6.4 and >8.0 days, respectively (). Similarly, when the challenge dose was 108 copies of MDPV per bird, the MDT values were 6.8 and 8.6 days, respectively (). Taken together, these results indicated that the K75N mutant is less virulent than the wild-type virus. All dead birds showed clinical signs and gross lesions typical of MDPV infection. PCR amplification and sequence analysis showed that MDPV DNA with the wild-type and K75N mutant sequences were present in tissues of ducks infected with the corresponding virus. Note that the K75N mutant eventually killed 90–100% of the embryos or birds after a longer incubation time (), suggesting that the K75N mutant is only partially attenuated.
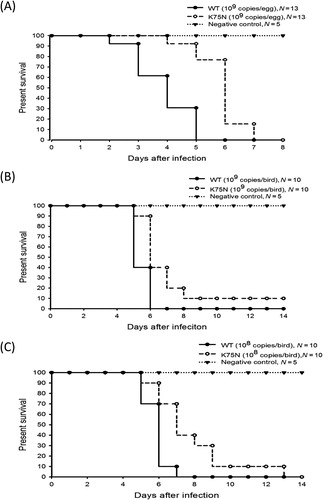
Discussion
Infectious plasmid clones of many parvoviruses have been established. Most of these clones were constructed by sub-cloning two or more DNA segments of the viral genome, and then combining these segments together by ligation to generate full-length infectious clones (Muramatsu et al., Citation1996; Soderlund-Venermo et al., Citation2001; Zhi et al., Citation2004; Qiu et al., Citation2005; Wang et al., Citation2014). Direct cloning of the full-length viral DNA into the plasmid vector was normally unsuccessful. This is because the double-stranded form of the viral DNA contains a blunt-end structure, and ligation of a blunt-ended DNA molecule into a plasmid vector is difficult. In the present study, the full-length MDPV DNA was directly ligated into the pGEM-T Easy vector using the TA cloning methodology, and the cloned MDPV DNA was found to be infectious. To the best of our knowledge, this is the first report on the construction of an infectious clone of a parvovirus using TA cloning. This methodology may also be useful for constructing infectious clones of GPV and other parvoviruses. The viruses rescued in this study are unlikely to originate from contamination because the mutated virus contains K75N mutation that is not found in the wild-type virus and no virus is rescued from the plasmid clone containing lethal mutation. However, an experiment that uses the plasmid only as a transfection control is required to further eliminate the possibility of contamination.
The infectious clone methodology is a valuable tool for studying the pathogenic mechanism of the viruses, as well as for developing vaccines against viral diseases. In Taiwan, the disease caused by GPV is controlled using a live vaccine strain, designated as 82-0321V. This vaccine strain was derived from the field strain 82-0321 by passage in Muscovy duck embryos (Tsai et al., Citation2004). However, a similar strategy failed to develop a safe, attenuated vaccine strain of MDPV, and thus no live vaccine strain of MDPV is available in Taiwan. A novel strategy for developing a vaccine strain of MDPV is via site-directed mutagenesis of an infectious clone. Data presented in this study showed that MDPV could be rescued from the K75N mutant clone, and that the rescued K75N virus is less virulent than the wild-type virus. Although the K75N mutant is only partially attenuated, this strain may serve as a parental strain for further mutagenesis work to develop a safe vaccine strain of MDPV. The potential targets for mutagenesis should include the amino acids that were found to differ between the virulent and vaccine strains of GPV developed in different countries (Tatár-Kis et al., Citation2004; Shien et al., Citation2008; Wang et al., Citation2014).
A previous study showed that the K75N mutation is present in vaccine strains and low-pathogenic field strains of GPV isolated in Europe (Tatár-Kis et al., Citation2004). However, no site-directed mutagenesis data were yet available to verify that the K75N mutation is responsible for the attenuation of GPV. Data presented in this study showed that the K75N mutation reduces the virulence of MDPV, indicating that this mutation is involved in the attenuation of MDPV and possibly GPV. The molecular mechanism underlying the attenuating effect of the K75N mutation remains unclear. However, as the K75N mutation is located in close proximity to the putative catalytic site (HDXXY) and Ca2+-binding site of the PLA2 domain (YXGXG; Zadori et al., Citation2001), it is possible that the K75N mutation may affect the PLA2 activity and subsequently reduce the virulence of GPV and MDPV.
In summary, an infectious plasmid clone of MDPV was constructed using TA cloning in this study, and the K75N mutation in the VP1 protein was verified to reduce the virulence of MDPV. The TA cloning methodology may be useful for constructing infectious clones of GPV and other parvoviruses. Furthermore, the infectious clone of MDPV should prove valuable to further elucidate the pathogenic mechanisms of waterfowl parvoviruses and to develop a safe, attenuated vaccine against diseases caused by MDPV.
Additional information
Funding
References
- Brown, K.E., Green, S.W. & Young, N.S. (1995). Goose parvovirus–an autonomous member of the dependovirus genus? Virology, 210, 283–291.
- Chang, P.C., Shien, J.H., Wang, M.S. & Shieh, H.K. (2000). Phylogenetic analysis of parvoviruses isolated in Taiwan from ducks and geese. Avian Pathology, 29, 45–49.
- Glávits, R., Zolnai, A., Szabo, E., Ivanics, E., Zarka, P., Mato, T. & Palya, V. (2005). Comparative pathological studies on domestic geese (Anser anser domestica) and Muscovy ducks (Cairina moschata) experimentally infected with parvovirus strains of goose and Muscovy duck origin. Acta Veterinaria Hungarica, 53, 73–89.
- Holton, T.A. & Graham, M.W. (1991). A simple and efficient method for direct cloning of PCR products using ddT-tailed vectors. Nucleic Acids Research, 19, 1156.
- Le Gall-Reculé, G. & Jestin, V. (1994). Biochemical and genomic characterization of Muscovy duck parvovirus. Archives of Virology, 139, 121–131.
- Muramatsu, S., Mizukami, H., Young, N.S. & Brown, K.E. (1996). Nucleotide sequencing and generation of an infectious clone of adeno-associated virus 3. Virology, 221, 208–217.
- Palya, V.J. (2013). Parvovirus infections of Waterfowl. In D.E. Swayne, J.R. Glisson, L.R. McDougald, L.K. Nolan, D.L. Suarez, & V.L. Nair. (2013). Diseases of Poultry 13th edn (pp. 444–463). Ames, IA: Blackwell Publishing.
- Qiu, J., Cheng, F., Yoto, Y., Zadori, Z. & Pintel, D. (2005). The expression strategy of goose parvovirus exhibits features of both the Dependovirus and Parvovirus genera. Journal of Virology, 79, 11035–11044.
- Reed, L.J. & Muench, H. (1938). A simple method of estimating fifty percent endpoints. American Journal of Hygiene, 27, 493–497.
- Shien, J.H., Wang, Y.S., Chen, C.H., Shieh, H.K., Hu, C.C. & Chang, P.C. (2008). Identification of sequence changes in live attenuated goose parvovirus vaccine strains developed in Asia and Europe. Avian Pathology, 37, 499–505.
- Soderlund-Venermo, M., Riley, L.K. & Pintel, D.J. (2001). Construction and initial characterization of an infectious plasmid clone of a newly identified hamster parvovirus. Journal of General Virology, 82, 919–927.
- Tatár-Kis, T., Mato, T., Markos, B. & Palya, V. (2004). Phylogenetic analysis of Hungarian goose parvovirus isolates and vaccine strains. Avian Pathology, 33, 438–444.
- Tsai, H.J., Tseng, C.H., Chang, P.C., Mei, K. & Wang, S.C. (2004). Genetic variation of viral protein 1 genes of field strains of waterfowl parvoviruses and their attenuated derivatives. Avian Diseases, 48, 512–521.
- Wang, J., Duan, J., Meng, X., Gong, J., Jiang, Z. & Zhu, G. (2014). Cloning of the genome of a goose parvovirus vaccine strain SYG61v and rescue of infectious virions from recombinant plasmid in embryonated goose eggs. Journal of Virological Methods, 200, 41–46.
- Zadori, Z., Erdei, J., Nagy, J. & Kisary, J. (1994). Characteristics of the genome of goose parvovirus. Avian Pathology, 23, 359–364.
- Zadori, Z., Stefancsik, R., Rauch, T. & Kisary, J. (1995). Analysis of the complete nucleotide sequences of goose and Muscovy duck parvoviruses indicates common ancestral origin with adeno-associated virus 2. Virology, 212, 562–573.
- Zadori, Z., Szelei, J., Lacoste, M.C., Li, Y., Gariepy, S., Raymond, P., Allaire, M., Nabi, I.R. & Tijssen, P. (2001). A viral phospholipase A2 is required for parvovirus infectivity. Developmental Cell, 1, 291–302.
- Zhi, N., Zadori, Z., Brown, K.E. & Tijssen, P. (2004). Construction and sequencing of an infectious clone of the human parvovirus B19. Virology, 318, 142–152.