Abstract
Influenza A viruses (IAVs) and avian paramyxoviruses (APMVs) are important pathogens of poultry worldwide, and both commonly occur in wild waterfowl, especially ducks in the family Anatidae. Although wood ducks (Aix sponsa) are members of the Anatidae, their behaviour differs from most other species in this family, which could affect the transmission of IAVs and APMVs. We collected cloacal and oropharyngeal swab and blood samples from more than 700 wood ducks across nine states in the eastern United States of America. No IAVs were isolated, and based on blocking enzyme-linked immunoassay ELISA results, antibodies to IAVs were only detected in 0.2% of samples. In contrast, 23 (3%) APMVs were isolated (22 Newcastle disease virus and 1 APMV-6), and antibodies to multiple serotypes of APMVs were detected in more than 60% of the samples. After-hatch-year birds were more likely to be antibody positive for APMV-4 and APMV-6 compared to hatch-year birds. Female birds were more likely to be antibody positive for APMV-4 than were male birds. Our results indicate that wood ducks are probably not an important host for IAV but are frequently infected with APMVs.
Introduction
Influenza A viruses (IAVs) and avian paramyxoviruses (APMVs) are important pathogens of poultry worldwide. Wild birds in the family Anatidae are known to play a role in the epidemiology of infections with IAVs and some serotypes of APMVs in wild birds (Hinshaw et al., Citation1985; Olsen et al., Citation2006). However, the contribution of some individual waterfowl species in the maintenance of IAVs and APMVs in wild bird populations is not well understood.
Although wood ducks are members of the family Anatidae, their behaviour differs from most other ducks in this family. In the eastern portion of North America wood ducks breed from Newfoundland, Canada, to Florida, USA, and they utilize forested habitats for cavity nesting, roosting and brood rearing (Bellrose & Holm, Citation1994). Wood ducks also have a different feeding ecology from other North American waterfowl species, primarily feeding on acorns at certain times of the year (Gilmer et al., Citation1978; Folk & Hepp, Citation2003). The differences in behaviour and habitat use probably affect their exposure to IAVs and APMVs.
Several studies have reported negative results for IAV detection in wood ducks, but most of these studies included fewer than 60 individuals (Rosenberger et al., Citation1974; Bahl et al., Citation1975; Webster et al., Citation1976; Kocan et al., Citation1979; Nettles et al., Citation1985; Hinshaw et al., Citation1986; Slemons et al., Citation1991). Three studies have attempted to isolate virus from more than 100 wood ducks, and two of these studies reported a low prevalence (less than 2.5%) of viral shedding compared to other dabbling duck species (Deibel et al., Citation1985; Goekjian et al., Citation2011; Wilcox et al., Citation2011). These studies suggest that wood ducks do not contribute to the maintenance of IAVs in wild bird populations; however, results from three other studies contrast with these studies. A study from Canada detected IAVs in 68% (71/104) of wood ducks using reverse transcription polymerase chain reaction (RT-PCR) (Parmley et al., Citation2008). Two additional studies reported virus isolations from 11.9% of wood ducks sampled during a 1-week period on the eastern shore of Maryland, USA, and 8% of wood ducks sampled in Alberta, Canada; however, the total number of wood ducks sampled was not given (Hinshaw et al., Citation1985; Slemons et al., Citation2003). Finally, most of these studies only tested cloacal swabs. However, it has since been reported that wood ducks predominantly shed IAVs via the oropharyngeal route (Costa et al., Citation2011), indicating that the role wood ducks play in the epidemiology of infections with IAVs may be spatially dependent or poorly defined due to sampling focused on cloacal swabs.
Two studies have reported IAV antibody test results for wood ducks, and in both cases no antibodies were detected (Kocan et al., Citation1979; Brown et al., Citation2010). One of these studies only tested serum from three wood ducks using a blocking ELISA (CitationBrown et al., 2010), and the other study did not report sample size, but sera were tested by the agar gel immunodiffusion assay, which has been shown to perform poorly in waterfowl species (Bahl et al., Citation1975; Brown et al., Citation2009). Experimentally infected wood ducks have developed an antibody response that has been detected up to 21 days after exposure to IAVs (Brown et al., Citation2009; Costa et al., Citation2011).
Eight APMV serotypes, Newcastle disease virus (NDV) as classified by the International Committee on Classification of Viruses and synonymous with APMV-1, as classified by the World Organization for Animal Health (OIE), APMV-2, -3, -4, -6, -7, -8 and -9, have been isolated from wild waterfowl, with NDV, APMV-4, -6 and -8 being most commonly reported (Alexander et al., Citation1983; Hinshaw et al., Citation1985). What little information is known about IAVs and APMVs in wood ducks indicates that APMVs are isolated more frequently than IAVs (Goekjian et al., Citation2011). Antibodies to APMV in ducks have been reported in four studies (Kocan et al., Citation1979; Vickers & Hanson, Citation1982; Maldonado et al., Citation1995; Stanislawek et al., Citation2002). Two of these were conducted in North America, but they did not sample wood ducks and only tested for antibodies to NDV (Kocan et al., Citation1979; Vickers & Hanson, Citation1982).
Currently there are limited and sometimes conflicting results on the prevalence of IAV in wood ducks and a paucity of information on APMV prevalence and serotype diversity in this species. The objective of this study was to sample wood ducks in the eastern USA and use virus isolation and serology to detect viral shedding and exposure to IAVs and APMVs in order to determine if wood ducks in this area play a role in the epidemiology of infections with these viruses in waterfowl.
Materials and Methods
Sample collection
Cloacal and oropharyngeal swabs (n = 724) and whole-blood samples (n = 727) were collected from wood ducks captured by rocket nets and dip nets in eight states (Florida, Kentucky, Maryland, North Carolina, Pennsylvania, South Carolina, Vermont and Virginia) between 16 July and 19 September 2009, and adult female wood ducks were collected by hand during nest box surveys in Maine. Sample collections took place during bird banding programmes conducted by the United States Fish and Wildlife Service and several state agencies (see Acknowledgements). Cloacal and oropharyngeal swab samples were placed in the same tube with brain heart infusion broth (BD Biosciences, Franklin Lanes, New Jersey, USA) supplemented with penicillin G (1000 units/ml), streptomycin (1 mg/ml), gentamycin (50 mg/ml), kanamycin (50 mg/ml) and amphotericin B (0.025 mg/ml) and were either kept on ice for less than 1 day or refrigerated (4°C) for no more than 2 days before overnight shipping. In addition, some samples were stored in liquid nitrogen in the field until transported to the Southeastern Cooperative Wildlife Disease Study (University of Georgia, Athens, Georgia, USA). Swab samples were stored at –80°C until testing. Blood samples were collected from the brachial vein, placed in Vacutainer® serum separator tubes (BD Biosciences), allowed to clot and stored on ice until serum collection; serum was stored at –20°C until serological testing.
Virus isolation and identification
Virus isolations were attempted in specific pathogen-free embryonated chicken eggs as previously described (Alexander & Senne, Citation2008). Allantoic fluid that agglutinated chicken red blood cells (CRBCs) was further tested by rRT-PCR for IAV. Viral RNA was extracted from allantoic fluid using QIAmp® Viral RNA Mini Kit (QIAGEN Inc., Valencia, California, USA) and tested for the presence of the IAV matrix gene by rRT-PCR (Spackman et al., Citation2002). In addition, all haemagglutinating allantoic fluid samples were screened for seven APMV serotypes (NDV, APMV-2, -4, -6, -7, -8 and -9) using a haemagglutination inhibition (HI) assay (Alexander & Senne, Citation2008). Reference antisera was obtained from the Poultry Diagnostic Research Center (PDRC; University of Georgia, Athens, GA, USA) for NDV and from the National Veterinary Service Laboratories (NVSL, United States Department of Agriculture, Ames, IA, USA) for APMV-4, -6 and -8. All samples positive by APMV HIs were then screened by RT-PCR with serotype-specific primers to the corresponding virus to verify HI results. For NDV, RT-PCR for the fusion cleavage site was performed as described (Aldous et al., Citation2003), and primers were designed for APMV-6 to amplify a region of the large polymerase gene: APMV6-11510 (5′-AAGGGCTATGCCAAAAAATGTG-3′) and APMV6-12014 (5′-GGAAAAATCAATGTCAAAAAGAA-3′). Conditions for the APMV-6 RT-PCR reaction were: 20 min at 42°C, then 2 min at 94°C followed by 94°C for 1 min, 1 min at 55°C and 1 min at 72°C 45 times, followed by 72°C for 10 min. The NDV fusion cleavage sites of four random isolates were bi-directionally sequenced (Georgia Genomics Facility, University of Georgia, Athens, GA, USA). In addition, the large polymerase gene amplicon from the one APMV-6 isolate was sequenced to confirm identification. NDVs used in this study were lentogenic viruses and would be classified as APMV-1 under the classification of the OIE.
Serology
IAV serology was conducted using a commercial blocking ELISA (IDEXX Laboratories, Westbrook, Maine, USA) to detect antibodies to the nucleoprotein following the manufacturer's instructions (Brown et al., Citation2009). Serology for the APMVs was conducted using an HI assay against the four APMV serotypes (NDV, APMV-4, -6 and -8) most commonly isolated from wild ducks as described by Alexander and Senne (Citation2008). Briefly, viruses were cultured in 9-day-old embryonated chicken eggs, and allantoic fluids were inactivated with a final concentration of 0.1% β-propiolactone. The pH of the antigens was adjusted to 7 with 10% sodium bicarbonate solution. Viruses used for antigen preparation were MADU/US(MN)/AI07-4044 for NDV, MADU/US(MN)/AI07-3119 for APMV-4, MADU/US(MN)/AI07-4783 for APMV-6. These viruses were isolated at the Southeastern Cooperative Wildlife Disease Study (University of Georgia, Athens, GA, USA) and serotyped at NVSL. The antigen for APMV-8 was obtained from PDRC. The HI assay was performed as previously described, using eight haemagglutination units of virus/25 µl/well (Alexander & Senne, Citation2008). A sample was considered antibody positive if the titre was equal to or greater than 32. In addition, 663 wood duck serum samples were tested for non-specific agglutination of CRBCs. Positive control reference antisera were obtained from the PDRC for NDV and the NVSL for APMV-4, APMV-6 and APMV-8.
Statistical analysis
All statistical analyses were conducted using R v3.0.2. We used population-averaged generalized estimating equations (GEEs) logistic regression to compare differences in antibody prevalence estimates across all four APMV serotypes by age and sex using the Zelig v4.2 package in R (Lam, Citation2014). Significance was based on the 95% confidence intervals of the odds ratio. We used population-averaged GEE to account for clustering of samples at locations and dates (Hanley et al., Citation2003). Age and gender data were not available for all samples, and those samples were not included in these analyses. Interaction between age and sex was examined for all models, and significance was analysed using a Wald test; non-significant interactions were removed from the model.
Results
Virus isolation
We detected haemagglutinating agents in 3% (23/724) of the wood duck swab samples. Allantoic fluid harvested from these positive samples was negative for IAV by matrix gene RT-PCR. Using the HI assay, 22 of the samples were identified as NDV and one sample as APMV-6 (). The HI results were confirmed by RT-PCR for all 23 isolates. Sequenced analysis of the fusion cleavage sites of five NDV isolates (two from NC, and one each from PA, MD and VT) did not contain additional basic amino acids and were consistent with avirulent NDV (110-GGERQERL-117; GenBank KM974721-KM974725). All five isolates were class I, whilst the MD isolate was genotype 5; one NC isolate was genotype 7, and the remaining three were genotype 2. The large polymerase gene segment amplified from the one APMV-6 isolate was 91% similar to APMV-6/duck/HongKong/18/199/77 (GenBank # EU622637). All isolates were from hatch-year birds. Age data were not available for seven of the NDV-positive birds.
Table 1. Results of attempted virus isolation for IAVs and APMVs in wood ducks and antibody prevalence as determined by a blocking ELISA.
Serology
We detected antibodies to the IAV nucleoprotein in 0.3% (2/727) of sampled wood ducks (). In contrast, antibody prevalence was more than 66% for NDV, APMV-4, -6 and -8, except for APMV-4 in Maine, Vermont and Virginia, and APMV-8 in Vermont and Virginia (). In samples that were tested for antibodies to all four APMV serotypes, we detected antibodies to them all in 48% (312/647); to three in 31% (135/647); to two in 27% (175/647); and to one serotype in 3.4% (22/647). The distribution of antibody titres to these viruses is shown in . We did not detect any interaction between age and gender in our population-averaged GEE logistic regression model (Wald test P > 0.05). However, we did detect differences in antibody prevalence associated with age and gender. After-hatch-year birds had increased likelihood of exposure to APMV-4 and APMV-6 compared to hatch-year birds, and females had increased chance of exposure to APMV-4 compared to males (). Although not significant, after-hatch-year birds were more likely to have been exposed to NDV compared to hatch-year birds (). We did not detect any non-specific agglutination of CRBCs in the 663 wood duck serum samples tested.
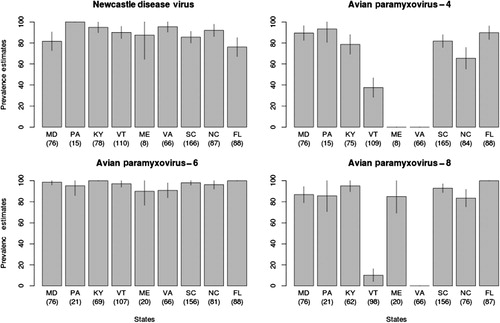
Table 2. Results from logistic regression utilizing GEEs for antibodies to APMV in wood ducks comparing after-hatch-year to hatch-year and female to male.
Discussion
Consistent with previous studies (Kocan et al., Citation1979; Goekjian et al., Citation2011) we detected limited evidence of IAV circulation among wood duck populations. These results are supported by the very low antibody prevalence we observed across a very broad geographical area, including northern latitudes where IAVs are more likely to be detected in waterfowl (Hinshaw et al., Citation1985). As a comparison, antibody prevalence in mallards (Anas platyrhynchos) and Canada geese (Branta canadensis) sampled during these same time periods can exceed 50% and 20%, respectively (Brown et al., Citation2010; Kistler et al., Citation2012). Although, the duration of the detectable IAV antibody response is not well defined for wood ducks, and may partially explain the low prevalence, antibodies to IAV nucleoprotein have been detected for more than 1 year in naturally exposed mallards (Fereidouni et al., Citation2010) and for 21 days in experimentally infected wood ducks and mallards (Costa et al., Citation2011). These results suggest that wood ducks are probably spillover hosts of IAVs, and it is possible that previously reported isolations of IAV from this species reflect such spillover (Deibel et al., Citation1985; Hinshaw et al., Citation1985; Wilcox et al., Citation2011). Two of the three studies that reported the isolation of IAV from wood ducks were performed in northern latitudes, during peak IAV shedding in waterfowl (late summer early fall) and in aquatic habitats with large numbers of mallards, a known IAV reservoir species (Deibel et al., Citation1985; Hinshaw et al., Citation1985; Wilcox et al., Citation2011). Another study that detected a high prevalence of IAV (68%) using RT-PCR was also conducted under similar conditions across Canada (Parmley et al., Citation2008). Additionally, many of the samples examined in these studies were collected from baiting ducks which could increase transmission of IAV among waterfowl species (Soos et al., Citation2012).
Four APMV serotypes, NDV, APMV-4, -6 and -8, are the APMVs most frequently isolated from waterfowl (Alexander et al., Citation1983; Hinshaw et al., Citation1985), but only NDV and APMV-4 have been reported in wood ducks (Goekjian et al., Citation2011). We isolated NDV and APMV-6 during this study, but our serological results provide evidence that wood ducks are commonly exposed to other APMV serotypes. Our APMV virus isolation prevalence was lower than in two previous studies that sampled wood ducks during the same time of year. One study conducted in North Carolina detected viral shedding in approximately 9% of wood ducks, and the second study conducted in New York reported isolation of APMVs from more than 8% of wood ducks (Deibel et al., Citation1985; Goekjian et al., Citation2011). In comparison with our study, wood ducks in these two studies were sampled from the same areas for an extended period of time (more than 1 month), whilst we only sampled for approximately 2 days at each location. Our short sampling period makes it possible that we missed the peak of virus circulation. In wood ducks, seasonal peaks in NDV prevalence (between approximately 15% and 40%) that subsided rapidly were reported from birds sampled in North Carolina; however, a high prevalence could not be detected 7–10 days after these peaks (Goekjian et al., Citation2011).
Most of the NDVs we isolated came from hatch-year birds, which is consistent with other reports of APMVs in waterfowl (Deibel et al., Citation1985). Virulent NDV is an important pathogen in poultry and is characterized by the addition of basic amino acids at the fusion protein cleavage site (Collins et al., Citation1993). In waterfowl NDV isolates usually have fusion protein cleavage sites consistent with avirulent NDV (Jindal et al., Citation2009). Our fusion cleavage site analysis supports this. APMV-6 has been commonly reported from various waterfowl species (Hinshaw et al., Citation1985; Goekjian et al., Citation2011), but this is the first reported isolation from a wood duck.
We detected a high prevalence of antibodies to NDV, APMV-4, -6 and -8 serotypes in this study. These data suggest that wood ducks are frequently exposed to four serotypes of APMVs. The lower level of antibody detection (less than 50%) to APMV-4 and APMV-8 in some states cannot be explained, but these prevalence estimates are based on only one or two sampling locations per state and differences may reflect local spatial variation. In Canada geese, significant variation of IAV antibody prevalence has been reported at sample locations within 10 km of each other (Kistler et al., Citation2012). Only low prevalence estimates of NDV antibodies in North American waterfowl (less than 16%) have previously been reported (Kocan et al., Citation1979; Vickers & Hanson, Citation1982), and to our knowledge, there have been no reports of testing for antibodies to other APMVs in North American waterfowl. We detected antibodies to more than one APMV serotype in most of the wood duck sera tested against all four serotypes. Others have reported antibodies to several APMV subtypes in waterfowl, however, they did not state how many were positive for multiple subtypes (Maldonado et al., Citation1994; Stanislawek et al., Citation2002). This high prevalence of antibodies to multiple APMVs serotypes may be due to cross reactivity among serotypes. Cross reactivity between APMV subtypes has been reported (Lipkind & Shihmanter, Citation1986). We used a high titre cut off (>32) compared to the OIE recommendation (>8) to reduce the potential for false-positive results and cross reaction (Office International des Epizooties, Citation2012). In addition, we did not detect any cross reaction greater than 16 in testing the antigen used against the antisera we used for the study.
The antibody prevalence to all APMVs was high in hatch-year birds, indicating that infection probably occurred during the summer. Transmission of NDV in wood duck populations during the summer has been reported previously (Goekjian et al., Citation2011). We did detect differences in antibody prevalence by age and gender for APMV-4, or by age for APMV-6; however, these results should be interpreted with caution. The lower confidence limits for the estimated odds ratios are very close to one. In addition, we did not confirm infection of wood ducks with either APMV-4 or APMV-8 by virus isolation. Although our serological data support the suggestion of wood ducks playing a significant role in the epidemiology of multiple serotypes of APMVs, there is insufficient historical virus isolation data to support this. Part of this relates to the fact that most APMV isolates are a by-product of IAV-targeted surveillance and research. These studies have most frequently taken place in late summer and fall, and this seasonality may not correspond to times of peak APMV shedding (Hinshaw et al., Citation1985; Wilcox et al., Citation2011). This situation will not be improved by the use of molecular screening techniques for IAV detection, which will for the most part eliminate the possibility of APMV detection.
Acknowledgements
The authors would like to thank the following for field and lab assistance: Barbara Shock, Josh Parris, Andrea Howey-Newsome and Andrew Allison [Southeastern Cooperative Wildlife Disease Study (SCWDS)]; John Dunn, John Morgan and Walt Cottrell (Pennsylvania Game Commission); Larry Hindman and Denny Price (Maryland Department of Natural Resources); Gary Costanza (Virginia Department of Game and Inland Fisheries); Bill Crenshaw (Vermont Fish and Wildlife Department); Judy Sefchick Edwards (Mississquoi National Wildlife Refuge in Vermont); Kelsey Sullivan (Maine Department of Inland Fisheries and Wildlife); Andrew Fanning (Florida Fish and Wildlife Conservation Commission); Doug Howell (North Carolina Wildlife Resource Commission); Haven Barnhill (Santee National Wildlife Refuge in South Carolina); Erin Harper (Kentucky Department of Fish and Wildlife Resources); Roy Berghaus for statistical analysis assistance; and Drs Egbert Mundt and Patti Miller for providing reference antigen and antisera. The findings and conclusions in this article are those of the authors and do not necessarily represent the views of the U.S. Fish and Wildlife Service.
Additional information
Funding
References
- Aldous, E.W., Mynn, J.K., Banks, J. & Alexander, D.J. (2003). A molecular epidemiological study of avian paramyxovirus type 1 (Newcastle disease virus) isolates by phylogenetic analysis of a partial nucleotide sequence of the fusion protein gene. Avian Pathology, 32, 237–255. 10.1080/0307945031000097831
- Alexander, D.J. & Senne, D.A. (2008). Newcastle diseases virus and other avian paramyxoviruses. In L. Dufour-Zavala, D.E. Swayne, J.R. Glisson, J.E. Pearson, W.M. Reed, M.W. Jackwood, & P.R. Woolcock (Eds.), Laboratory Manual for the Isolation, Identification, and Characterization of Avian Pathogens 5th edn (pp. 135–141). Athens, GA: American Association of Avian Pathologists.
- Alexander, D.J., Hinshaw, V., Collins, M. & Yamane, N. (1983). Characterization of viruses which represent further distinct serotypes (PMV-8 and PMV-9) of avian paramyxoviruses. Archives of Virology, 78, 29–36. 10.1007/BF01310856
- Bahl, A.K., Pomeroy, B.S., Easterday, B.C. & Mangundimedjo, S. (1975). Isolation of type A influenza viruses from the migratory waterfowl along the Mississippi Flyway. Journal of Wildlife Diseases, 11, 360–363. 10.7589/0090-3558-11.3.360
- Bellrose, F.C. & Holm, D.J. (1994). Ecology and Management of the Wood Duck (G.C. Sandersen, R.E. McCabe & A. Hodgins, Eds., 1st edn p. 588). Mechanicsburg, PA: Stackpole Books.
- Brown, J.D., Luttrell, M.P., Berghaus, R.D., Kistler, W., Keeler, S.P., Howey, A., Wilcox, B., Hall, J., Niles, L., Dey, A., Knutsen, G., Fritz, K. & Stallknecht, D.E. (2010). Prevalence of antibodies to type A influenza virus in wild avian species using two serologic assays. Journal of Wildlife Diseases, 46, 896–911. 10.7589/0090-3558-46.3.896
- Brown, J.D., Stallknecht, D.E., Berghaus, R.D., Luttrell, M.P., Velek, K., Kistler, W., Costa, T.P., Yabsley, M.J. & Swayne, D. (2009). Evaluation of a commercial blocking enzyme-linked immunosorbent assay to detect avian influenza virus antibodies in multiple experimentally infected avian species. Clinical and Vaccine Immunology, 16, 824–829. 10.1128/CVI.00084-09
- Collins, M.S., Bashiruddin, J.B. & Alexander, D.J. (1993). Deduced amino acid sequences at the fusion protein cleavage site of Newcastle disease viruses showing variation in antigenicity and pathogenicity. Archives of Virology, 128, 363–370. 10.1007/BF01309446
- Costa, T.P., Brown, J.D., Howerth, E.W. & Stallknecht, D.E. (2011). Variation in viral shedding patterns between different wild bird species infected experimentally with low-pathogenicity avian influenza viruses that originated from wild birds. Avian Pathology, 40, 119–124. 10.1080/03079457.2010.540002
- Deibel, R., Emord, D.E., Dukelow, W., Hinshaw, V.S. & Wood, J.M. (1985). Influenza viruses and paramyxoviruses in ducks in the Atlantic flyway, 1977–1983, including an H5N2 isolate related to the virulent chicken virus. Avian Diseases, 29, 970–985. 10.2307/1590450
- Fereidouni, S.R., Grund, C., Häuslaigner, R., Lange, E., Wilking, H., Harder, T.C., Beer, M. & Starick, E. (2010). Dynamics of specific antibody responses induced in mallards after infection by or immunization with low pathogenicity avian influenza viruses. Avian Diseases, 54, 79–85. 10.1637/9005-073109-Reg.1
- Folk, T.H. & Hepp, G.R. (2003). Effects of habitat use and movement patterns on incubation behavior of female wood ducks (Aix sponsa) in southeast Alabama. The Auk, 120, 1159–1167. 10.1642/0004-8038(2003)120[1159:EOHUAM]2.0.CO;2
- Gilmer, D., Ball, I. & Cowardin, L. (1978). Natural cavities used by wood ducks in north-central Minnesota. Journal of Wildlife Management, 42, 288–298. 10.2307/3800266
- Goekjian, V.H., Smith, J.T., Howell, D.L., Senne, D.A., Swayne, D.E. & Stallknecht, D.E. (2011). Avian influenza viruses and avian paramyxoviruses in wintering and breeding waterfowl populations in North Carolina, USA. Journal of Wildlife Diseases, 47, 240–245. 10.7589/0090-3558-47.1.240
- Hanley, J.A., Negassa, A., Edwardes, M.E. & Forrester, J.E. (2003). Statistical analysis of correlated data using generalized estimating equations: an orientation. American Journal of Epidemiology, 157, 364–375. 10.1093/aje/kwf215
- Hinshaw, V.S., Nettles, V.F., Schorr, L.F., Wood, J.M. & Webster, R.G. (1986). Influenza virus surveillance in waterfowl in Pennsylvania after the H5N2 avian outbreak. Avian Diseases, 30, 207–212. 10.2307/1590635
- Hinshaw, V.S., Wood, J.M., Webster, R.G., Deibel, R. & Turner, B. (1985). Circulating influenza and paramyxoviruses in waterfowl from two different locations in North America. Bulletin of the World Health Organization, 63, 711–719.
- Jindal, N., Chander, Y., Chockalingam, A.K., de Abin, M., Redig, P.T. & Goyal, S.M. (2009). Phylogenetic analysis of Newcastle disease viruses isolated from waterfowl in the upper midwest region of the United States. Virology Journal, 6, 191–200. 10.1186/1743-422X-6-191
- Kistler, W.M., Stallknecht, D.E., Deliberto, T.J., Swafford, S., Pedersen, K., Van Why, K., Wolf, P.C., Hill, J.A, Bruning, D.L., Cumbee, J.C., Mickley, R.M., Betsill, C.W., Randall, A.R., Berghaus, R.D. & Yabsley, M.J. (2012). Antibodies to avian influenza viruses in Canada geese (Branta canadensis): a potential surveillance tool? Journal of Wildlife Diseases, 48, 1097–1101. 10.7589/2011-02-046
- Kocan, A., Shaw, M. & Morgan, P. (1979). Some parasitic and infectious diseases in waterfowl in Oklahoma. Journal of Wildlife Diseases, 15, 137–141. 10.7589/0090-3558-15.1.137
- Lam, P. (2007). logit.gee: general estimating equation for logistic regression. In K. Imai, G. King & O. Lau (Eds.), Zelig: Everyone’s Statistical Software. http://gking.harvard.edu/zelig
- Lipkind, M. & Shihmanter, E. (1986). Antigenic relationships between avian paramyxoviruses. Archives of Virology, 89, 89–111. 10.1007/BF01309882
- Maldonado, A., Arenas, A., Tarradas, M.C., Astorga, R., Perea, J.A. & Miranda, A. (1995). Serological survey for avian paramyxoviruses from wildfowl in aquatic habitats in Andalusia. Journal of Wildlife Diseases, 31, 66–69. 10.7589/0090-3558-31.1.66
- Maldonado, A., Arenas, A., Tarradas, M.C., Carranza, J., Luque, I., Miranda, A. & Perea, A. (1994). Prevalence of antibodies to avian paramyxoviruses 1, 2 and 3 in wild and domestic birds in southern Spain. Avian Pathology, 23, 145–152. 10.7589/0090-3558-31.1.66
- Nettles, V.F., Wood, J.M. & Webster, R.G. (1985). Wildlife surveillance associated with an outbreak of lethal H5N2 avian influenza in domestic poultry. Avian Diseases, 29, 733–741. 10.2307/1590665
- Office International des Epizooties. (2012). Newcastle disease. In Manual of Diagnostic Tests and Vaccines for Terrestrial Animals (pp. 555–573). http://www.oie.int/international-standard-setting/terrestrial-manual/access-online/
- Olsen, B., Munster, V.J., Wallensten, A., Waldenström, J., Osterhaus, A.D. & Fouchier, R.A.M. (2006). Global patterns of influenza A virus in wild birds. Science, 312, 384–388. 10.1126/science.1122438
- Parmley, E.J., Bastien, N., Booth, T.F., Bowes, V., Buck, P.A., Breault, A., Caswell, D., Daoust, P., Davies, J.C., Elahi, S.M., Fortin, M., Kibenge, F., King, R., Li, Y., North, N., Ojkic, D., Pasick, J., Pryor, S.P., Robinson, J., Rodrigue, J., Whitney, H., Zimmer, P. & Leighton, F.A. (2008). Wild bird influenza survey, Canada, 2005. Emerging Infectious Diseases, 14, 84–87. 10.3201/eid1401.061562
- Rosenberger, J.K., Krauss, W.C. & Slemons, R.D. (1974). Isolation of Newcastle disease and type-A influenza viruses from migratory waterfowl in the Atlantic flyway. Avian Diseases, 18, 610–613. 10.2307/1589019
- Slemons, R.D., Hansen, W.R., Converse, K.A. & Senne, D.A. (2003). Type A influenza virus surveillance in free-flying, nonmigratory ducks residing on the eastern shore of Maryland. Avian Diseases, 47, 1107–1110.
- Slemons, R.D., Shieldcastle, M.C., Heyman, L.D., Bednarik, K.E. & Senne, D.A. (1991). Type A influenza viruses in waterfowl in Ohio and implications for domestic turkeys. Avian Diseases, 35, 165–173.
- Soos, C., Parmley, E.J., McAloney, K., Pollard, B., Jenkins, E., Kibenge, F. & Leighton, F.A. (2012). Bait trapping linked to higher avian influenza virus detection in wild ducks. Journal of Wildlife Diseases, 48, 444–448. 10.7589/0090-3558-48.2.444
- Spackman, E., Senne, D.A., Myers, T.J., Bulaga, L.L., Garber, L.P., Perdue, M.L., Lohman, K., Daum, L.T. & Suarez, D.L. (2002). Development of a real-time reverse transcriptase PCR assay for type A influenza virus and the avian H5 and H7 hemagglutinin subtypes. Journal of Clinical Microbiology, 40, 3256–3260. 10.1128/JCM.40.9.3256-3260.2002
- Stanislawek, W.L., Wilks, C.R., Meers, J., Horner, G.W., Alexander, D.J., Manvell, R.J., Kattenbelt, J.A. & Gould, A.R. (2002). Avian paramyxoviruses and influenza viruses isolated from mallard ducks (Anas platyrhynchos) in New Zealand. Archives of Virology, 147, 1287–1302. 10.1007/s00705-002-0818-2
- Vickers, M.L. & Hanson, R.P. (1982). Newcastle disease virus in waterfowl in Wisconsin. Journal of Wildlife Diseases, 18, 149–158. 10.7589/0090-3558-18.2.149
- Webster, R.G., Morita, M., Pridgen, C. & Tumova, B. (1976). Ortho- and paramyxoviruses from migrating feral ducks: characterization of a new group of influenza A viruses. Journal of General Virology, 32, 217–225. 10.1099/0022-1317-32-2-217
- Wilcox, B.R., Knutsen, G.A., Berdeen, J., Goekjian, V., Poulson, R., Goyal, S., Sreevatsan, S., Cardona, C., Berghaus, R.D., Swayne, D.E., Yabsley, M.J. & Stallknecht, D.E.(2011). Influenza-A viruses in ducks in northwestern Minnesota: fine scale spatial and temporal variation in prevalence and subtype diversity. PLoS ONE, 6, e24010. 10.1371/journal.pone.0024010.s003