Abstract
Marek's disease virus (MDV) is an oncogenic alphaherpesvirus and the causative agent of Marek's disease (MD), characterized by immunosuppression, paralysis, nerve enlargement and induction of T-cell lymphomas in chickens. Despite widespread usage of vaccines since the 1970s to control MD, more virulent field strains of MDV have emerged that overcome vaccinal protection, necessitating the development of new and more protective MD vaccines. The ∆Meq virus, a recombinant Md5 strain MDV lacking the viral oncogene Meq, is one candidate MD vaccine with great potential but unfortunately it also causes bursal–thymic atrophy (BTA) in maternal antibody negative chickens, raising concerns that impede commercial use as a vaccine. Previously, we identified a point mutation within UL5 that reduced in vivo replication in attenuated viruses. We proposed that introduction of the UL5 point mutation into the ∆Meq virus would reduce in vivo replication and eliminate BTA yet potentially retain high protective abilities. In birds, the ∆Meq+UL5 recombinant MDV had reduced replication compared to the original ∆Meq virus, while weights of lymphoid organs indicated that ∆Meq+UL5 did not induce BTA, supporting the hypothesis that reduction of in vivo replication would also abolish BTA. Vaccine trials of the ∆Meq+UL5 virus compared to other ∆Meq-based viruses and commercial vaccines show that, while the ∆Meq+UL5 does provide vaccinal protection, this protection was also reduced compared to the original ∆Meq virus. Therefore, it appears that a very delicate balance is required between levels of replication able to induce high vaccinal protection, yet not so high as to induce BTA.
Introduction
Marek's disease (MD) is an economically significant disease of chickens that costs the worldwide poultry industry $1–2 billion a year (Morrow & Fehler, Citation2004). The causative agent of MD is the oncogenic herpesvirus known as Gallid herpesvirus 2, commonly referred to as Marek's disease virus (MDV). Characteristic signs of MD include depression, transient paralysis, nerve lesions, tumours and death of affected birds. The primary method used to successfully control MD since the 1970's is vaccination. The first vaccine against MD was the attenuated strain of MDV known as HPRS-16, which was shortly followed by the introduction of another vaccine utilizing the closely related turkey herpesvirus known as HVT (Churchill et al., Citation1969; Purchase & Okazaki, Citation1971).
While vaccines have been used to successfully prevent MD and reduce losses in the poultry industry for over 40 years ago, more virulent strains have evolved requiring the introduction of a successive line of new vaccines (Witter, Citation2001). Disease breaks within HVT-vaccinated flocks resulted in the need to introduce bivalent vaccines, which combined two vaccines that interacted synergistically, such as HVT and another avirulent virus such as SB-1, to yield a vaccine that was more protective than either vaccine alone (Witter & Lee, Citation1984). Bivalent vaccines controlled losses due to MD until the evolution of more virulent field strains led to the introduction of the CVI988-Rispens (Rispens) vaccine in the USA (Rispens et al., Citation1972; Witter, Citation1997). Presently, the Rispens vaccine is considered the gold-standard vaccine against MD and provides the highest level of protection commercially available.
Despite the level of success in controlling MD, there is concern that more virulent strains of MDV are evolving able to overcome even Rispens vaccinal protection (Gimeno, Citation2008). Currently a recombinant cosmid-based Md5 strain MDV in which the Meq oncogene has been deleted, known as rMd5∆Meq, has shown great potential in several trials. Unfortunately, rMd5∆Meq causes bursal–thymus atrophy (BTA) in maternal antibody negative birds and, as a result, has not been licenced for commercial use as a vaccine. Attempts to eliminate BTA of rMd5∆Meq via repeated serial passage in vitro to attenuate the virus resulted in viruses that did not cause BTA in antibody negative birds (Lee et al., Citation2012), but failed to replicate and lost the superior protective qualities of the initial rMd5∆Meq virus in commercial antibody positive birds (Lee et al., Citation2013). It is postulated that BTA seen in the otherwise avirulent rMd5∆Meq virus is the result of vigorous in vivo replication of the virus within the bursa and thymus, leading to atrophy of those lymphoid organs (Dunn & Silva, Citation2012; Lee et al., Citation2013).
During previous efforts to identify the driving factors for MDV attenuation at a genetic level, we identified a point mutation within UL5, the helicase primase subunit, that resulted in significant reduction of in vivo replication of the virus, while significantly reducing MD incidence to 0–11% as well as providing protection against challenge with virulent MDV (Hildebrandt et al., Citation2014). Considering the hypothesis that loss of BTA seen in the high-passage rMd5∆Meq resulted from considerable reduction in replication after passage, we predicted that addition of the UL5 point would reduce replication of ∆Meq to low levels seen in the UL5 point mutant, while simultaneously eliminating BTA characteristic of low passage ∆Meq viruses, but still confer vaccinal protection.
A second Meq deletion mutant generated using an infectious MDV BAC construct, known as Md5B40BAC∆Meq, has previously been created and shown to be as protective as rMd5∆Meq, while still equaling, if not surpassing, protection of Rispens (Silva et al., Citation2010). Therefore, use of the BAC-cloned MDV allowed us to use Red-mediated recombineering to create a double mutant of Md5B40BAC∆Meq+UL5 (Hildebrandt et al., Citation2014). We compared in vivo replication and ratios of lymphoid organ weights for low passage ∆Meq, high passage ∆Meq (p41), and the double mutant, Md5B40BAC∆Meq+UL5, against uninfected birds. Furthermore, we tested the three preceding ∆Meq-based viruses as well as two commercially approved vaccines, CVI988 and bivalent (HVT+SB-1), for vaccinal protection against challenge with 648A, a very virulent plus (vv+) strain of MDV.
Materials and Methods
Viruses and tissue culture
Md5B40∆Meq BAC generated and characterized by Silva et al. (Citation2010) was used for incorporation of the UL5 point mutation using Red-mediated recombineering protocols and mutational primers as previously described (Hildebrandt et al., Citation2014). The resulting Md5B40BAC∆Meq+UL5 (∆Meq+UL5) recombinant mutant contained deletion of both copies of Meq and the nonsynonymous point mutation of amino acid I682R within UL5. Viral stocks of Md5B40∆Meq BAC (∆Meq BAC) at p5 and ∆Meq+UL5 p4 were used for birds trials, while rMd5∆Meq p39 (Lee et al., Citation2012, Citation2013) was plated and passed through chicken embryo fibroblast for one additional passage to amplify viral stocks before use of the resulting rMd5∆Meq p40 (∆Meq p40) for bird trials. The very virulent plus (vv+) MDV 648A (p7) (Witter, Citation1997) strain was used as the challenge virus. The two MD vaccines compared were (1) bivalent vaccine consisting of HVT (p10) and SB-1 (p14) strains and (2) Rispens (p43); viruses originated from ADOL stocks. Cells and viruses were cultured in a 1:1 mixture of Leibovitz's L-15 and 100 McCoy's 5A (LM) media containing 1–4% of foetal bovine serum with antibiotics as previously described (Hildebrandt et al., Citation2014).
In vivo replication and bursal–thymic atrophy (BTA)
Twenty ADOL 15I5 × 71 maternal antibody negative chicks were vaccinated with 2000 PFU of ∆Meq BAC p5, ∆Meq p40 or ∆Meq+UL5 p4 at day of hatch and housed in Horsfall–Bauer (HB) unit isolators. In addition to vaccinated birds, an isolator of unvaccinated birds was maintained for negative controls. Birds were bled at days 6, 13 and 20 days post vaccination (dpv) and peripheral blood lymphocytes (PBL) isolated using Histopaque-1077 density gradient separation (Sigma-Aldrich, St. Louis, MO, USA). DNA extracted from PBLs was used for qPCR analysis to compare in vivo replication among vaccines. The Taqman Fast Universal PCR kit (Applied Biosciences, Foster City, CA, USA) and primers for amplification of chicken GAPDH and MDV gB were used to calculate the relative ratio of the number of MDV genomes versus chicken genome numbers as previously described (Gimeno et al., Citation2008; Hildebrandt et al., Citation2014).
To monitor BTA, five birds from each of the four lots (∆Meq BAC p5, ∆Meq p40, ∆Meq+UL5 p4 or unvaccinated) were sacrificed at 15 dpv, the time when atrophy is typically most severe (Dunn & Silva, Citation2012), and their total body weight (g) and weights of bursa and thymus (mg) measured. The ratio of lymphoid organs over total body weight was calculated to compare against unvaccinated controls to assay atrophy of lymphoid organs in vaccinated birds. Ratios of lymphoid organs over total body weight were analysed using ANOVA to determine differences between vaccinated lots and unvaccinated controls. Bird experiments to determine in vivo replication and BTA were conducted in two sequential studies designated as Trials 1 and 2.
Vaccinal protection
To assay vaccinal protection of Meq-deleted viruses, we compared the three Meq-deleted viruses previously described against two MD vaccines: Rispens/CVI988 and bivalent (HVT+SB-1). Sixteen to 18 Maternal antibody negative, ADOL line 15I5 × 71 chicks were vaccinated with 2000 PFU of ∆Meq BAC p5, ∆Meq p40, ∆Meq+UL5 p4, bivalent (HVT+SB-1) or Rispens at day of hatch and housed in HB units. After 5 dpv, vaccinated birds were challenged with 500 pfu vv+ 648A strain of MDV. As positive controls, one lot of unvaccinated birds was challenged with 500 PFU of 648A at 5 days of age. Unvaccinated and uninfected negative control birds were also housed and maintained with the experimental lots in HB units for 8 weeks. Birds that died before 8 weeks were examined via necropsy for cause of death, while after 8 weeks all surviving birds were terminated and examined via necropsy for signs of MD. To determine vaccinal protection, we calculated protective index (PI) of vaccines as follows; PI = [(% MD incidence in unvaccinated) –(% MD incidence in vaccinated)] / [% MD incidence in unvaccinated]. To compare protection of vaccines, analysis using Fisher's exact test was performed to identify statistically significant differences in MD among vaccinated groups. Bird experiments to determine vaccinal protection were conducted in two sequential studies designated as Trials 1 and 2.
All experiments were approved by the USDA, ADOL Animal Care and Use Committee (ACUC). The ACUC guidelines established and approved by the ADOL ACUC (April 2005) and the Guide for the Care and Use of Laboratory Animals by the Institute for Laboratory Animal Research (2011) were followed throughout the experiments.
Results
In vivo replication
Unvaccinated birds and birds vaccinated with either ∆Meq+UL5 p4 or ∆Meq p40 all had very low levels of detectable MDV at all three time points (). ∆Meq challenged birds had high levels of viral DNA at 6 dpv. Similarly, virulent 648A had high virus levels at 13 days post challenge. There were statistically significant differences in replication at 6 dpv (P < 0.01), in which the low passage ∆Meq BAC p5 had high levels of replication at 6 dpv, which then dropped at 13 and 20 dpv to levels comparable to unvaccinated birds and the two other Meq-based viruses. Unvaccinated birds challenged with 648A had slightly higher levels of replication at 6 dpv, but significant differences in levels of replication were apparent at 13 dpv compared to the unvaccinated controls and ∆Meq-based viruses. No data are available at 20 days post challenge for 648A infected birds due to the high levels of mortality of nearly all birds within 20 days after challenge.
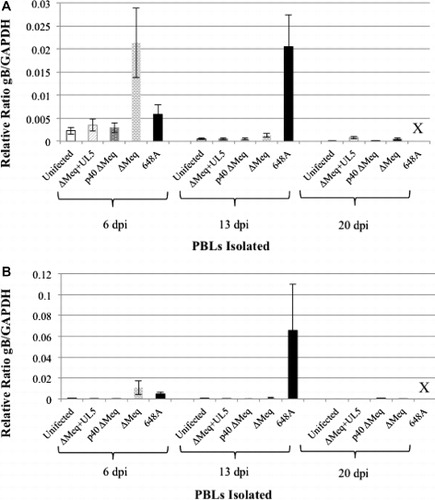
BTA of Meq-based viruses
Ratios comparing the weights of lymphoid organs, either individual bursa and thymus weights or total lymphoid organs, to the total body weight of birds at 15 dpv showed a significant difference in the weights of lymphoid organs in birds vaccinated with low passage ∆Meq BAC p5 () compared to unvaccinated birds (P < 0.001 in all trial comparisons). Individual bursa and thymus weights, or total lymphoid organ to body weight ratios of birds vaccinated with either ∆Meq p40 or ∆Meq+UL5 p4 were not significantly different from unvaccinated (ANOVA, P > 0.15 in all trial comparisons).
Protective efficacy of vaccines
Both trials showed severe mortality in unvaccinated birds challenged with 648A (). Due to infection with this vv+ strain of MDV, over 90% of unvaccinated birds challenged with 648A were deceased within 2–3 weeks post challenge. In Trial 1, all birds vaccinated with ∆Meq p40, bivalent, or Rispens prior to challenge survived the full 8 week experiment, while ∆Meq BAC p5 had 1 death out of 15 birds (7% mortality) and 4 out of 14 birds died when vaccinated with ∆Meq+UL5 p4 (29% mortality; ). In Trial 2, similar trends were also observed in which 80–90% of birds vaccinated with either ∆Meq+UL5 p4, ∆Meq BAC p5, ∆Meq p40 or Rispens survived the full 8 week experiment compared to unvaccinated birds challenged with 648A, which were all deceased by 3 weeks post challenge ().
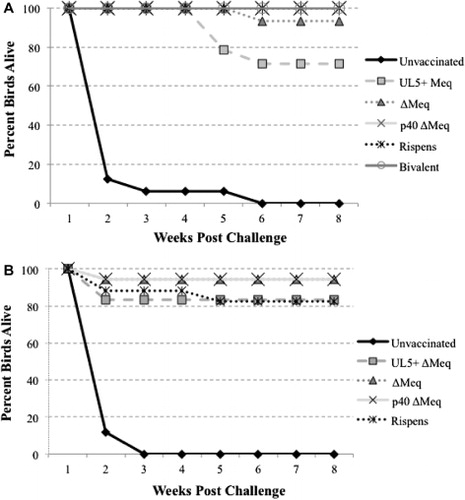
Examination via necropsy to determine MD incidence revealed very high protection following vaccination with ∆Meq BAC p5, ∆Meq p40 and Rispens. Of these highly protective vaccines, ∆Meq BAC p5 had the lowest PI of 93, while ∆Meq p40 and Rispens had no MD positive birds, resulting in PIs of 100 for both viruses in Trial 1 (). The ∆Meq+UL5 p4 and bivalent viruses had higher levels of MD incidence in birds vaccinated with these viruses, resulting in PIs of 57–43 or 87–73 for each respective virus, showing they did not confer as strong of protection as the previous three viruses, but they still provided significant protection against challenge with vv+ MDV. Results of Trial 2 also supported these conclusions in which ∆Meq BAC p5, ∆Meq p40 and Rispens all provided high protection against MD (PIs of 94, 94 and 88, respectively), while lower levels of protection were provided by vaccination with ∆Meq+UL5 p4 (PI 44).
Table 1. Vaccinal protection against challenge with 648A strain MDV.
Discussion
While low passage ∆Meq is a highly effective vaccine able to protect against vv+ MDV strains, it also induces BTA in maternal antibody negative chickens, inhibiting commercial licencing of the virus as a vaccine (Dunn & Silva, Citation2012). In addition to BTA, another trait characteristic of low passage ∆Meq is the vigorous in vivo replication of ∆Meq during early cytolytic replication. This characteristic was previously described and is apparent in this study as well (Lupiani et al., Citation2004; Dunn & Silva, Citation2012). Due to loss of vigorous in vivo replication following serial passage of ∆Meq, it was postulated this high replication within lymphoid organs was the cause of BTA. We hypothesized that addition of a point mutation within UL5 shown to cause greatly reduced in vivo replication would abrogate BTA in low passage ∆Meq, resulting in an avirulent virus that does not induce tumours and replicates at very low levels as to not cause BTA. Comparing replication via qPCR of PBLs and the weight of lymphoid organs of the three ∆Meq-based viruses compared to unvaccinated birds supported the hypothesis that reducing in vivo replication in the ∆Meq+UL5 p4 virus also resulted in a low passage ∆Meq-based virus that failed to cause BTA. In addition to the anticipated phenotypic changes in regard to in vivo replication and lymphoid organ weight, addition of the UL5 point mutation also affected vaccinal protection of the ∆Meq+UL5 p4 compared to the original ∆Meq BAC p5 virus. This illustrates the fine interplay between even a single point mutation intended to reduce in vivo replication and broader implications on vaccinal protection, suggesting a delicate balance between vaccinal protection, in vivo replication and BTA.
Previous studies have explored the interaction between MD vaccines and replication. Studies comparing vaccinal protection of serially passed and fully attenuated MDV strains relative to earlier passages still containing residual levels of virulence, designated as partially attenuated viruses, showed that partially attenuated viruses had higher viral loads in PBLs and conferred significantly higher levels of protection than the fully attenuated passages of the viruses (Witter, Citation2002). Further studies exploring the interaction between protection and replication of candidate vaccines compared a set of several vaccine candidates categorized as high protective or low protective vaccines in order to identify characteristics that appeared common among high or low protective vaccines (Gimeno et al., Citation2004). One trait the authors noted that distinguished high from low protective vaccines was in vivo replication. Looking at viral load of high protective viruses revealed higher viral loads in all tissues quantified, with significantly higher levels in lymphoid organs such as the bursa, thymus and spleen.
These studies collectively suggest a correlation of greater vaccinal protection with high in vivo replication. Unfortunately it is not uncommon to find that highly protective, high replicating MD vaccine candidates also induce BTA. One such example is the virus known as RM1, which was generated by cocultivation of MDV and reticuloendotheliosis virus, leading to the integration of retroviral insertions into the MDV genome. RM1 was determined to be attenuated and replicated efficiently in vivo, yet failed to cause MD and was typified by severe BTA (Jones et al., Citation1996). Further characterization revealed that not only was the attenuated RM1 virus very protective as a vaccine, but also exhibited high tissue tropism for early dissemination and replication to the thymus (Gimeno et al., Citation2011). Among several vaccine viruses examined, RM1 showed the earliest and highest replication within lymphoid organs, specifically the thymus, compared to the vaccine viruses that did not cause BTA.
Additional studies looking at in vivo replication within the thymus comparing both RM1 and low passage ∆Meq against in vivo replication of viruses that do not induce BTA, such as the lower replicating Rispens vaccine, show both RM1 and low pass ∆Meq have significantly higher levels of replication within the thymus (Dunn & Silva, Citation2012). This supports the hypothesis that increased replication, specifically within lymphoid organs such as the bursa and thymus, leads to atrophy of these sensitive organs. A confounding factor for vaccine development is that despite the negative effects causing BTA due to high levels of in vivo replication, increased replication appears to be one factor impacting higher degrees of vaccinal protection conferred by viruses. Therefore, in vivo replication and the protection qualities of candidate vaccines appear to be highly interconnected, although not the only factor believed to play a role in protection, leading to complications when trying to eliminate BTA by altering excessive replication while still producing a protective vaccine.
Modulation of in vivo replication has been a method proposed for generation of attenuated vaccines by increasing replication fidelity of the viral polymerase in a polio mouse model. High-fidelity polio vaccines exhibiting altered tissue tropism resulted in attenuated viruses with diminished transmission and shed of virus (Vignuzzi et al., Citation2008). These high-fidelity replication variant viruses were also able to generate excellent immune responses and induce antibody levels comparable to, or even exceeding, current Sabin polio vaccines. Considering the trend that moderate-to-high levels of replication tends to result in more protective MD vaccines than their low replicating counterparts, it may be advantageous to try to direct replication and limit tissue tropism for these vaccine candidates. Considering that viruses such as RM1 and low passage ∆Meq replicate at high levels in lymphoid organs, and those tissues suffer atrophy as a result of this vigorous replication, it may be beneficial to restrict the tissue tropism of prolific replication to avoid BTA. By diverting replication, this may allow low passage ∆Meq viruses to maintain their high levels of replication in tissues other than the bursa and thymus and prevent BTA, while also provoking a strong immune response due to vigorous early cytolytic replication. Considering that MDV is a highly cell-associated virus whose lifecycle involves preferential infection and replication within B and T cells, it may be difficult to directly apply this approach with MDV, but it is clear that alternative approaches besides simply reducing in vivo replication should be considered for development of protective candidate vaccines.
Ongoing development of more protective MD vaccines clearly illustrates the difficulty in generating vaccines that are not only more protective, but also do not cause undesirable secondary reactions, as seen with low pass ∆Meq or RM1 viruses which induce BTA. Attempts to generate new vaccine candidates have yielded many viruses which confer vaccinal protection equaling that currently provided by Rispens, yet it has been difficult to surpass the protection of Rispens (Witter & Kreager, Citation2004). Two candidate vaccines, RM1 and low passage ∆Meq, both met or exceeded vaccinal protection commonly seen by Rispens, but both viruses also induce BTA in maternal antibody negative birds, prohibiting their approval as commercial vaccines (Gimeno et al., Citation2004). Attempts to eliminate BTA in low passage ∆Meq in previous studies by extensive serial passage resulted in a virus with reduced in vivo replication and which did not cause BTA, but also reduced protection compared to the original low passage ∆Meq (Lee et al., Citation2013). This study aiming to eliminate BTA in low passage ∆Meq by reducing in vivo replication via addition of the UL5 point mutation showed similar results as previous trials, in which BTA was lost at the expense of vaccinal protection.
Clearly there is a fine balancing act between generating a highly protective vaccine that elicits a strong immune response with mild residual pathogenicity, compared to viruses that are completely avirulent, yet may not induce as vigorous immune response as the virus’ partially virulent counterpart. As shown in this study and supported by previous work, it appears easier to eliminate undesirable BTA characteristics of a virus such as ∆Meq, but a far more complicated task to do so without also simultaneously reducing vaccinal protection. Perhaps new routes must be considered to address this problem of BTA in highly protective viruses, such as trying to restrict the tissue tropism of replication, instead of simply reducing replication to eliminate BTA, which has been shown to be an unsatisfactory method to address BTA without also undermining high vaccinal protection.
Acknowledgement
We would like to thank Laurie Molitor for assistance with birdwork, Spencer Jackson for assistance in PBL isolation, DNA extraction and qPCR preparation, as well as Lonnie Milam for excellent technical advice.
Additional information
Funding
References
- Churchill, A.E., Chubb, R.C. & Baxendale, W. (1969). The attenuation, with loss of oncogenicity, of the herpes-type virus of Marek's disease (strain HPRS-16) on passage in cell culture. Journal of General Virology, 4, 557–564.10.1099/0022-1317-4-4-557
- Dunn, J.R. & Silva, R.F. (2012). Ability of MEQ-deleted MDV vaccine candidates to adversely affect lymphoid organs and chicken weight gain. Avian Diseases, 56, 494–500.10.1637/10062-011812-Reg.1
- Gimeno, I.M. (2008). Marek's disease vaccines: a solution for today but a worry for tomorrow? Vaccine, 26, C31–C41.10.1016/j.vaccine.2008.04.009
- Gimeno, I.M., Cortes, A.L. & Silva, R.F. (2008). Load of challenge Marek's disease virus DNA in blood as a criterion for early diagnosis of Marek's disease tumors. Avian Diseases, 52, 203–208.10.1637/8089-081407-Reg.1
- Gimeno, I.M., Witter, R.L., Cortes, A.L. & Reed, W.M. (2011). Replication ability of three highly protective Marek's disease vaccines: implications in lymphoid organ atrophy and protection. Avian Pathology, 40, 573–579.10.1080/03079457.2011.617725
- Gimeno, I.M., Witter, R.L., Hunt, H.D., Reddy, S.M. & Reed, W.M. (2004). Biocharacteristics shared by highly protective vaccines against Marek's disease. Avian Pathology, 33, 57–66.10.1080/0307945031000163264
- Hildebrandt, E., Dunn, J.R., Perumbakkam, S., Niikura, M. & Cheng, H.H. (2014). Characterizing the molecular basis of attenuation of Marek's disease virus via in vitro serial passage identifies de novo mutations in the helicase-primase subunit gene UL5 and other candidates associated with reduced virulence. Journal of Virology, 88, 6232–6242.10.1128/JVI.03869-13
- Jones, D., Brunovskis, P., Witter, R. & Kung, H.J. (1996). Retroviral insertional activation in a herpesvirus: transcriptional activation of US genes by an integrated long terminal repeat in a Marek's disease virus clone. Journal of Virology, 70, 2460–2467.
- Lee, L.F., Heidari, M., Zhang, H., Lupiani, B., Reddy, S.M. & Fadly, A. (2012). Cell culture attenuation eliminates rMd5ΔMeq-induced bursal and thymic atrophy and renders the mutant virus as an effective and safe vaccine against Marek's disease. Vaccine, 30, 5151–5158.10.1016/j.vaccine.2012.05.043
- Lee, L.F., Kreager, K., Heidari, M., Zhang, H., Lupiani, B., Reddy, S.M. & Fadly, A. (2013). Properties of a meq-deleted rMd5 Marek's disease vaccine: protection against virulent MDV challenge and induction of lymphoid organ atrophy are simultaneously attenuated by serial passage in vitro. Avian Diseases, 57, 491–497.10.1637/10388-092612-Reg.1
- Lupiani, B., Lee, L.F., Cui, X., Gimeno, I., Anderson, A., Morgan, R.W., Silva, R.F., Witter, R.L., Kung, H.-J. & Reddy, S.M. (2004). Marek's disease virus-encoded Meq gene is involved in transformation of lymphocytes but is dispensable for replication. Proceedings of the National Academy of Sciences, 101, 11815–11820.10.1073/pnas.0404508101
- Morrow, C. & Fehler, F. (2004). Marek's disease: a world-wide problem. In F. Davison & V. Nair (Eds.), Marek’s disease (pp. 49–61). San Diego, CA: Academic Press.
- Purchase, H.G. & Okazaki, W. (1971). Effect of vaccination with herpesvirus of turkeys (HVT) on horizontal spread of Marek's disease herpesvirus. Avian Diseases, 15, 391–397.10.2307/1588710
- Rispens, B.H., van Vloten, H., Mastenbroek, N., Maas, H.J.L. & Schat, K.A. (1972). Control of Marek’s disease in the Netherlands. I. Isolation of an avirulent Marek’s disease virus (strain CVI 988) and its use in laboratory vaccination trials. Avian Diseases, 16, 108–125.10.2307/1588905
- Silva, R.F., Dunn, J.R., Cheng, H.H. & Niikura, M. (2010). A MEQ-deleted Marek's disease virus cloned as a bacterial artificial chromosome is a highly efficacious vaccine. Avian Diseases, 54, 862–869.10.1637/9048-090409-Reg.1
- Vignuzzi, M., Wendt, E. & Andino, R. (2008). Engineering attenuated virus vaccines by controlling replication fidelity. Nature Medicine, 14, 154–161.10.1038/nm1726
- Witter, R.L. (1997). Increased virulence of Marek's disease virus field isolates. Avian Diseases, 41, 149–163.10.2307/1592455
- Witter, R.L. (2001). Protective efficacy of Marek's disease vaccines. In P.D.K. Hirai (Ed.), Marek’s Disease, Current Topics in Microbiology and Immunology (pp. 57–90). Berlin: Springer.
- Witter, R.L. (2002). Induction of strong protection by vaccination with partially attenuated serotype 1 Marek's disease viruses. Avian Diseases, 46, 925–937.10.1637/0005-2086(2002)046[0925:IOSPBV]2.0.CO;2
- Witter, R.L. & Kreager, K.S. (2004). Serotype 1 viruses modified by backpassage or insertional mutagenesis: approaching the threshold of vaccine efficacy in Marek's disease. Avian Diseases, 48, 768–782.10.1637/7203-050304R
- Witter, R.L. & Lee, L.F. (1984). Polyvalent Marek's disease vaccines: safety, efficacy and protective synergism in chickens with maternal antibodies. Avian Pathology, 13, 75–92.10.1080/03079458408418510