Abstract
We analysed the effects of cold stress (19 ± 1°C, 6 h /day, from the first to the seventh day of life) applied to specific pathogen free (SPF) chickens. On experimental Day 1 (ED1), chicks were divided into four groups: C (not infected and kept under thermoneutral condition); CS (not infected and cold stressed); PC (Salmonella Heidelberg (SH) infected and kept under thermoneutral condition) and PCS (SH infected and cold stressed). High concentrations of corticosterone were found in the cold stressed birds on ED7 and ED21, with a greater increase in birds of the PCS group. Stress or non-stressed SH-infected birds had high levels of norepinephrine on ED21. On ED21, an increased percentage and number of SH were found in birds of the PCS group. On ED7, a decrease in macrophages presenting MHCII, CD8+ and CD8+ γδ cells was observed in the chickens of the CS group. Decrease was observed in CD3+ cells in the birds of the PCS group and increase in macrophages presenting MHCII cells and of the CD4+/CD8+ ratio in chickens of the CS group on ED21. There was a decrease in CD8+ γδ cells in birds of the CS group on ED21 and in the CD3+ and CD8+cell numbers in chickens of the PCS group on ED21. Our results suggest that cold stress applied to chickens in the first 7 days of life increases both the hypothalamus pituitary adrenal axis and the sympathetic nervous system activities, leading to long-term immune cell dysfunction, thus allowing increased SH invasion and persistence within the birds’ body.
Introduction
In birds, particularly in poultry, Salmonella causes mostly sub-clinical infections. Thus, the bacteria can easily spread between birds within a flock because the infected birds might become intermittent or persistent healthy carriers. The prevalence of Salmonella in poultry populations is considered a main risk factor for the presence of the bacteria within table eggs and poultry meat (Hugas & Beloeil, Citation2014). Some zoonotic Salmonella enterica serovars are known to infect humans through contaminated food products of animal origin, and Salmonella enterica serovar Heidelberg (SH) has been implicated as an important causative agent linked to numerous human outbreaks, with invasive infections and even deaths (Foley et al., Citation2011). Contamination with SH has been reported in broilers, turkeys and laying hens in many countries, including Brazil (Borsoi et al., Citation2011).
The challenge imposed by Salmonella to poultry production is to reduce its prevalence in the birds; this includes good animal management in the field. Stressful situations, including inadequate housing conditions, overcrowding, extremes of environmental temperature, nutritional deprivation and others (Dawkins et al., Citation2004; Hoerr, Citation2010; Quinteiro-Filho et al., Citation2010; Lay et al., Citation2011), have been reported to enhance the risk of infectious diseases and to modify Salmonella infection severity (Glaser & Kiecolt-Glaser, Citation2005). The responses to the stressors, known as the stress response, are linked to behavioural and autonomic nervous system function alterations and to multiple hormones (Carrasco & Van de Kar, Citation2003). The major neural pathways activated by stressors are the hypothalamus pituitary adrenal axis and the sympathetic nervous system (SNS), which might lead to immune deregulation with significant health consequences, such as host resistance to viral infections, chronic autoimmune diseases, and tumour growth and metastasis (Costa-Pinto & Palermo-Neto, Citation2010; Powel et al., Citation2013).
Among environmental stressors, cold temperature represents a significant threat for poultry production; cold stress has been reported to affect birds’ health and welfare. Indeed, newly hatched chicks undergo a gradual temperature control change during their first days of life, that is, from poikilothermic to homoeothermic conditions (Collier et al., Citation1997). Thus, chicks are dependent on outside artificial heat sources in their first days of life because they are unable to regulate their internal body temperature (Hill, Citation2011). We thus designed the present study to analyse the effects of cold stress on bacterial colonization and immune system status in SPF chickens experimentally infected with Salmonella Heidelberg.
Materials and Methods
Bacterial preparation
The Salmonella Heidelberg strain SH23 (Borsoi et al., Citation2009) was retrieved from frozen medium and cultured overnight at 37°C in BHI broth (Oxoid®, Basingstoke, Hampshire, UK). The culture was then streaked on xylose-lysine-deoxycholatetergitol (XLT4) agar (Oxoid®) and incubated at 37°C for 24 h. A single colony was selected and transferred to BHI broth medium (Oxoid®) and incubated at 37°C at 180 rpm shaking and until an optical density of 0.1 was achieved at 600 nm (Thermo Spectronic Biomate 3, Thermo Fisher Scientific, Madison, WI, USA.). The incubation resulted in 3.5 × 109 colony forming units (cfu)/ml; dilutions were performed to adjust the inoculum to 3.5 × 104 cfu/ml.
Chicks
Specific pathogen free (SPF) day-old White Leghorn chicks were housed at the Experimental Center of Avian Pathology, School of Veterinary Medicine, University of São Paulo in four equal isolators (150 cm length × 100 cm width × 160 cm height – Alesco, São Paulo, Brazil) equipped with HEPA filters operating under negative pressure. The sterile conditions of the isolators were confirmed in our laboratory. Water and feed, free from antimicrobials and animal protein, were provided ad libitum to the animals. The birds were maintained and used in accordance with the guidelines of the Committee on the Care and Use of Laboratory Animal Resources of the School of Veterinary Medicine, University of São Paulo, Brazil (Protocol Number 2976/2013).
Experimental design and cold protocol
On experimental Day 1 (ED1), 100 SPF chicks were allocated into four different groups: control group (Group C; n = 18 birds), positive Salmonella Heidelberg control group (Group PC; n = 25 birds); negative Salmonella Heidelberg cold stressed group (Group CS; n = 25 birds), and positive Salmonella Heidelberg cold stressed group (Group PCS. n = 32). The PC and PCS chicks were inoculated orally on ED1 with 0.5 ml of BHI broth containing 3.5 × 104 cfu/ml of Salmonella Heidelberg, whereas those of the C and CS groups received 0.5 ml of BHI broth (Oxoid®, Basingstoke, Hampshire, UK).
From ED1 to ED21, the chickens from the C and PC groups were kept under conditions of thermal comfort. Birds of the cold stressed groups (CS and PCS groups) were subjected to a temperature of 19 ± 1°C for 6 h/day from ED1 to ED7 (from 32°C, the time to achieve 19°C was 1 h, and it took 1 h to return to 32°C); from ED8 to ED21 the chickens of these groups were kept under conditions of thermal comfort. The temperature and humidity inside the isolators were monitored daily and recorded with the help of a digital thermo-hygrometer (ITHT 2210 – Instrutemp, São Paulo, SP, Brazil) (Supplemental Tables 1 and 2). At ED7 and ED21, eight chickens per group had their heart blood collected and were euthanized afterwards for liver and caecum collections.
Bacterial isolation and quantification
To examine caecal colonization, the entire caeca were removed, placed in a sterile bag, weighed and processed (1:10) in a sterile solution of 0.1% buffered peptone in water (BPW) (Oxoid®, Basingstoke, Hampshire, UK). To perform the direct agar plate counting, the samples were diluted 10-fold in BPW 0.1% up to 10-4, and 100 µl and then streaked in duplicate into XLT4 agar plates with a Drigalski hook and incubated for 24 h at 37°C. The Salmonella cfu were counted, and the results were expressed according to the Plate Count Procedure recommended by the Brazilian Ministry of Livestock and Food Supply (MAPA, Citation2003). All samples were inoculated in tetrathionate (TT) broth (Oxoid®), incubated for 48 h at 37°C and streaked onto XLT4 agar plates. To perform liver bacterial quantification and isolation, the samples were removed, placed in a sterile bag and the same methodologies were performed for caecal content. One typical colony per plate was biochemically and antigenically screened to confirm the presence of Salmonella.
Corticosterone determination
Eight birds per group were randomly selected, and blood samples were collected from their hearts. To avoid circadian rhythm influences, blood was taken from the birds between 08:00 am and 10:00 am on both ED7 and ED21. The corticosterone concentrations were determined in the plasma using the Corticosterone Enzyme Immunoassay Kit (Arbor Assays®, Ann Arbor, MI, USA) according to the manufacturer's instructions, with an adaptation for 100 µl of plasma dilution (1:100). The plates were read in a Power Wave HT Microplate Spectrophotometer (BioTeK®, Winooski, VT, USA).
Catecholamine determination
Plasma samples stored at –80°C were retrieved; 500 µl of each sample, kept at room temperature, was added to 300 µl of 0.2 M perchloric acid solution plus the internal standard dihydroxybenzylamine (DHBA) (Sigma Aldrich, São Paulo, Brazil) and then centrifuged for 5 min at 3000×g. Immediately after, 1 ml of TRIS–EDTA (pH 8.6) and 30 mg of neutral alumina (Al2O3, Sigma Aldrich) were added to the samples, which were again centrifuged as described above. The supernatant was discarded and two washes were performed using 1 ml of ultrapure water followed by new centrifugations; 150 µl of the supernatant was then taken for the catecholamine assay. Epinephrine (EPI) and norepinephrine (NOR) were measured by high-pressure liquid chromatography (Shimadzu, model 6A, Kyoto, Japan) using a C – 18 column (Shimpak, ODS, Kyoto, Japan). An electrochemical detector (model 6A, Shimadzu), a sample injector (15 and 20 Al valve), and an integrator (Chromatopac, Shimadzu, Kyoto, Japan) were used. The detection limits for both EPI and NOR were 2 pg. Recovery values were higher than 80% for both substances, the coefficients of variation were less than 15%, and the linearity of the obtained curves was higher than 0.9.
Flow cytometry
Blood samples were collected directly from the heart at both ED7 and ED21; 10 µl of blood was taken and 100 µl of PBS was added to each sample before staining and lyses. The markers used for immunophenotyping are presented in ; 1 µl of each marker was added to the blood, except for CD3+ measurement, where 2 µl of marker was used. The samples were kept in the dark and at room temperature for 1 h. Immediately after, they were washed with 1 ml of PBS and centrifuged for 5 min at 400×g. The supernatants were discarded, and the tubes with the primary antibody biotin were conjugated with streptavidin in the dark and at room temperature for 45 min; the samples were then washed as described above. Samples were lysed by adding 990 µl of a lysing solution 1:10 (FACS Lysing Solution (BD Biosciences, San Jose, CA, USA) and incubated at 37°C for 10 min. After lysis, two washes were performed with 200 µl of PBS each. Flow cytometry acquisitions were performed on a BD FACSCalibur (BD Biosciences). For each sample, 20,000 events were saved. Titration of all antibodies was performed prior to the experiment to determine the optimal staining concentrations, and the multicolour panel was evaluated using fluorescence minus one controls and isotype controls for each fluorophore. The analyses were performed using the software FlowJo v10.0.7 (FlowJo, LLC, OR, USA) (Supplemental Figures 1–3).
Table 1. Markers used for blood cell immunophenotyping.
Statistical analyses
The statistical analyses were performed using the Biostat 2010 Software Analyst (Soft Inc., Alexandria, VA, USA) for parametric tests (stress and Salmonella factor interaction analyses) and/or a one-way ANOVA followed by a Tukey–Kramer test. P < 0.05 was considered significant for all of the comparisons. The data are presented as the mean ± standard deviation.
Results
Plasma corticosterone
As illustrated in (a) and 1(b), the cold stressed birds analysed on ED7 and ED21 had increased concentrations of corticosterone in the plasma compared to those of the control group (C group). These figures also showed that the SH-infected birds submitted to cold stress (PCS group) had higher levels of plasma corticosterone compared to those of the PC group, that is, birds infected and kept in a thermal comfort environment.
Figure 1. Effects of cold stress (19 ± 1°C, 6 h/day, from ED 1 to ED7) on corticosterone plasma levels (ng/ml) in SPF chickens studied at 7 (ED7) (a) and 21 (ED21) (b) days old that were infected (Group PCS) or not infected (Group CS) with Salmonella Heidelberg. Data are presented as the mean ± standard deviation (n = 8/group). *P < 0.05 compared to Control (Group C) and positive Salmonella Heidelberg control (Group PC) groups (two-way ANOVA followed by Tukey–Kramer test).
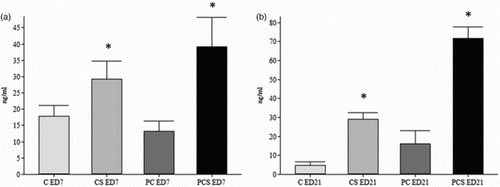
Plasma Epinephrine and Norepinephrine
No changes were found in catecholamine concentrations on ED7. However, and regardless of the presence of SH infection, the cold stressed birds had alterations in the plasma concentrations of NOR, but not in those of EPI, on ED21. Indeed, as given in , the birds of the SH-infected groups (PC and PCS) had increased NOR levels at ED21 compared to their respective control groups (C). Further analysis performed with ED21 data showed that the NOR levels of the birds of group PCS were higher than those of the PC group.
Table 2. Plasma concentrations of EPI and NOR in SPF chickens at ED7 and ED21 challenged or not with cold stress and/or Salmonella Heidelberg (SH).
Bacterial isolation and quantification
SH was not found in the tissues of the uninfected birds (C and CS) during the entire trial (data not shown). Livers positive for SH were found in 100% of the birds of the PC group and in 87% of the birds of the cold stressed (PCS) group at ED07. At ED21, the data on liver SH positivity in the chickens of the PC group decreased from 100% to 62%, while those of the cold stressed group remained at 87% positivity. Direct plating of liver tissues showed no statistical difference on SH counting comparing PC (ED7 2.0 log10/g and Ed21 0.85 log10/g) and PCS (ED7 2.3 log10/g and Ed21 0.88 log10/g) groups. As depicted in , all birds from the PC and PCS groups were positive for SH in the caeca, a finding recorded at both ED7 and ED21. SH quantification in the birds of the cold stressed group (PCS) was higher (4.34 log10 cfu/g) than that measured in the non-stressed (PC) group (3.6 log10 cfu/g) on ED21.
Figure 2. Effects of cold stress (19 ± 1°C, 6 h /day, from ED 1 to ED7) on Salmonella Heidelberg caecal counts in SPF chickens at 7 (ED7) and 21 (ED21) days of age in both infected groups, with PC kept at a thermoneutral temperature during the entire trial versus the cold stressed (PCS) group. *P < 0.05 compared with the PC and PCS groups (ANOVA followed by Tukey–Kramer test). The mortality of the birds was 20% in the PC group and 40.6% in the PCS group. There was no mortality during the entire trial in the C and CS groups.
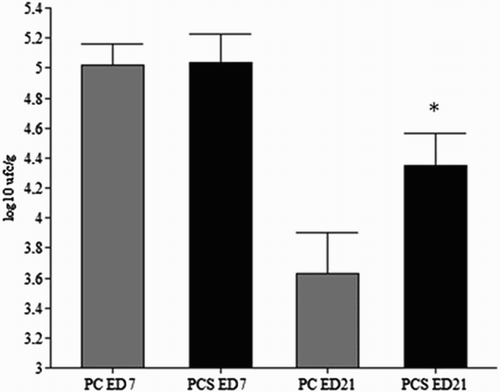
Flow cytometry
As given in , significant differences were observed on ED7 for Kul01/MHCII+, CD8+ and CD8−TCRI+ cells: a decreased percentage of cells was found in the birds of the cold stressed (CS) group in relation to birds of the C group. For the infected groups, significant differences were found for CD3+ cells; indeed, a decreased percentage of CD3+ cells was measured in the cold stressed birds (PCS group) in relation to those of the PC group. presents the data taken on ED21, that is, two weeks after the end of the cold stress application. An increase in Kul01/MHCII+, CD4+ cells and the CD4+/CD8+ ratio and a decrease in CD8+TCRI+ cell numbers were found in birds of the CS group compared to those of the C group. Considering the infected groups, the cold stressed birds (PCS group) had a decreased percentage of CD3+ and CD8+ cells compared to the unstressed group (PC group). A decreased percentage of CD3+ cells was observed during the entire trial in the infected and cold stressed birds (PCS group) in relation to their respective controls (PC group).
Table 3. Peripheral blood flow cytometry from SPF chickens at 7 days of age (ED7) challenged with cold stress and/or Salmonella Heidelberg (SH) groups or control groups.
Table 4. Peripheral blood flow cytometry from SPF chickens at 21 days of age (ED21) challenged with cold stress and/or Salmonella Heidelberg (SH) groups or control groups.
Discussion
Environmental stressors are a common occurrence in poultry production. Particularly relevant is the presence of cold stress in newly hatched chicks. Indeed, the chicks are still not able to regulate their body temperature when exposed to cold environmental conditions that may cause hypothermia. Environmental stressors were shown to negatively affect the bird's immune system, compromising its performance and ability to overcome bacterial infections (Humphrey, Citation2006; Dunkley et al., Citation2007; Burkholder et al., Citation2008).
Previous data on environmental stressor effects in poultry obtained in our laboratories showed that heat stress decreased broiler performance parameters and macrophage activity, induced intestinal injury and increased the amount of Salmonella Enteritidis recovered from the spleens of the stressed birds (Quinteiro-Filho et al., Citation2012). We also showed that broilers kept in an overcrowding stress situation had intestinal inflammation with mucosal immune cell infiltration, decreased macrophage activity and decreased plasma levels of immunoglobulin G in relation to non-stressed birds. These latter results were considered responsible for the increased amount of Salmonella Enteritidis recovered from the livers of the overcrowding-stressed and infected chickens (Quinteiro-Filho et al., Citation2012). In both studies, increased levels of serum corticosterone were found in the birds kept under environmental stress conditions, clearly indicating HPA-axis activation and participation in the immune data reported.
Stressors can activate the HPA-axis, leading to increased plasma concentrations of glucocorticoids (Glaser & Kiecolt-Glaser, Citation2005; Tachibana et al., Citation2007; Couto-Moraes et al., Citation2009). In the present study, cold stress (19°C ± 1 applied during 6 h a day from ED1 to ED7) increased the plasma levels of corticosterone in the birds of the CS group at both ED7 and ED21. Similarly, increased levels of corticosterone were reported in our previous studies after the end of the heat and overcrowding stressor application (Quinteiro-Filho et al., Citation2012; Gomes et al., Citation2014). In the present trial, increased concentrations of corticosterone were found in the birds of the CS group on ED21, that is, two weeks after the end of the cold stress period, suggesting a long-term dysfunction of the bird's HPA-axis induced by cold stress applied early in life. HPA-axis activation is commonly known to be initiated by the corticotrophin-releasing factor from the hypothalamus, leading to hypothalamic CRF over-expression that contributes to HPA-axis hyperactivity. Evidence has shown that glucocorticoid hormones participate in stress activation via feed-forward mechanisms at the level of the amygdala (Herman et al., Citation2012). Recent work of our group showed that heat stress and Clostridium perfringens infection produced significant differential responses in chickens’ behaviour and in c-fos expression in the paraventricular nucleus of the hypothalamus, central amygdala, preoptic medial area and globus pallidus of chickens (Calefi et al., personal observations, 2015). However, it should not be forgotten that rather than having a single defined feedback switch, the control of the stress response also requires a wide-reaching feedback network mechanism (Flandreau et al., Citation2012) that together coordinate HPA-axis activity to suit the overall needs of the multiple body systems.
An increased concentration of corticosterone was presently observed in the birds of the SH infected (PC and PCS groups), with higher levels in the infected and cold stressed birds (PCS group). Environmental stressors have been reported to impair broiler's intestinal integrity (Quinteiro-Filho et al., Citation2012; Gomes et al., Citation2014), allowing bacterial translocation from the gut to the host organism. Ando et al. (Citation2000) reported increased concentrations of corticosterone and IL-1 in mouse plasma in response to SH-derived LPS administration. Cytokines, such as IL-1 and IL-6, released during inflammatory processes were shown to increase the levels of systemic corticosterone (Besedovsky et al., Citation1986; Besedovsky & Del Rey, Citation2000). Thus and accordingly, it seems feasible to suggest that SH infection was a cornerstone for the increased plasma concentrations of corticosterone found in this study. Petrella et al. (Citation2014) reported that alteration in the HPA-axis responsiveness is recognized as a relevant component of stress-induced inflammatory gastrointestinal disorders. These authors also suggested that long-term HPA-axis activation was strictly associated with intestinal inflammation and visceral pain.
However and importantly, in response to the cold stress applied to the chicks in this study, not only was the HPA-axis activated but also the SNS. Indeed, presents increased levels of plasma NOR in the birds of the stressed groups (CS and PCS) on ED21. Most research data support the contention that intact or augmented central NA function is necessary to cope successfully with a variety of stress responses (Glavin, Citation1985; Haylley et al., Citation2001). NOR neurons within the CNS interact with those of the HPA-axis, with the latter system being responsible for the major neuroendocrine effects observed during the stress response. As such, activation of the central NOR pathways in the cold stressed chicks of the present work might have played an important integrative function in coping with the bird's adaptation to stress, as suggested before in a different experimental context by Pardon et al. (Citation2002). These authors, using the same cold stress model (7 days, 4 h /day, 4°C) applied to two different strains of rats, showed a huge activation of the HPA-axis and also of the stress-induced NOR release from neurons within the lateral bed nucleus of the stria terminalis. This functional change may be one mechanism whereby exposure to repeated or severe stress may induce pathologic dysregulation of the stress response in susceptible individuals (Tsigosa & Chrousos, Citation2002; Pardon et al., Citation2003) as in the birds of the present study.
SH infection was induced in the present work. Indeed, the infected birds (PC and PCS groups) had a high bacterial load within their caeca (100% of positivity). Furthermore, an increased positivity for SH was found in both livers and caeca of the cold stressed birds (PCS group) at ED21. These data indicate that cold stress-challenged birds had increased bacterial loads during the entire trial, suggesting a decreased capacity of these stressed birds to overcome the induced infection. These findings are supported by data reported elsewhere, that is, showing that corticosterone impairs broilers’ intestinal integrity, thus allowing bacterial translocation (Quinteiro-Filho et al., Citation2012; Gomes et al., Citation2014). Furthermore and importantly, SNS activation and NOR peripheral release might have also contributed to the present set of data. According to Lyte et al. (Citation2011), enteric bacteria respond directly to stress-related neurochemical mediators and notably to catecholamines, that is, bacterial infectivity might be influenced by NOR via a non-immunological manner. Pullinger et al. (Citation2010) showed that NOR and related catecholamines facilitate the supply of iron to Gram-negative bacteria under iron-limiting conditions. It may also be that virulent gene expression induction might result from a direct effect of catecholamines on bacteria via specific receptors, such as the presence of a non-alpha, non-beta, primitive adrenergic receptors (Roshchina, Citation2010).
Regarding stress effects on immune cells, our data suggested that (1) glucocorticoid seem to be the common link between the effects of stress on immunity; in fact, many of the effects on circulating leukocytes described during stress may be ascribed to increased levels of this hormone (Costa-Pinto & Palermo-Neto, Citation2010); (2) despite the well-known immunosuppressive properties of glucocorticoids, it is known that these hormones also possess opposing effects that emphasize a bimodal hormonal regulation of the immune responses (Petrella et al., Citation2014); (3) a growing body of evidence indicates that the SNS modulates the immune system functions; however, the effect of NOR on immune cells appears to be more complex, as NOR can have either a stimulatory or inhibitory effect depending on the type of immune cell, their activation status, the duration of NOR exposure and the dose of NOR employed (Slota et al., Citation2015).
The analysis of the peripheral immune cells undertaken after the last day of cold stress (ED7) might have been influenced by the high levels of corticosterone found in the birds of the stressed groups; the results taken at ED21, however, might have been influenced also by plasma NOR. The cold stressed birds (CS group) had a low Kul01/MHCII+ ratio on ED7, namely to the avian macrophage cells stained; thus, cold stress might have impaired at least one part of the innate immune system that is considered the first line of an animal's host defence to pathogens and antigen exposure (Kin & Sanders, Citation2006). In addition to that, the percentage of CD8+ and CD8− TCRI+ (γδ) cells was also smaller in the birds of the CS group. Chickens belong to a group of animals that have high frequencies of γδ T cells compared to mice and humans. Frequencies of γδ Tcells in chickens can reach up to 50% of peripheral lymphoid cells. As in mammals, the functional role of γδ T cells has not been clearly elucidated. However, the broad tissue distribution of γδ T cells suggests that they have important immune surveillance functions. While the biological functions of avian TCR γδ T cells are still unclear, in vitro studies have demonstrated that they are cytotoxic (Viertlboeck & Göbel, Citation2008).
Low percentages of CD3+ cells were found in the SH-infected and cold stressed birds (PCS group) at ED07. These data should be considered related to the immunosuppressive effects induced by corticosterone released during the cold stress. However, there have been cases in which immunity was altered during stress in the absence of changes in corticosterone concentrations, pointing again towards a possible role of catecholamines on immunity (Costa-Pinto & Palermo-Neto, Citation2010).
According to Zorrilla et al. (Citation2001), stressors are likely to induce the following effects: (1) an overall leukocytosis, manifesting as an absolute lymphocytosis; (2) alterations in cytotoxic lymphocyte levels, increased CD4+/CD8+ ratios, and natural killer cell cytotoxicity with the direction of change depending on the chronicity of the stressor; (3) a relative reduction of T-cell levels; (4) a reduced lymphocyte proliferative response and proportion of IL-2r bearing cells following mitogenic stimulation; and (5) increased leukocyte adhesiveness. In agreement with these statements, the birds of the CS group of the present study showed a high CD4+/CD8+ ratio on ED21 compared to those of the C group with increased percentage of CD4+ lymphocytes. The increased number of CD4+ lymphocytes found in this work in the cold stressed birds (CS group) might also be linked to the increased plasma levels of NOR found in these birds. Data from Kin and Sanders (Citation2006) showed that a purified population of naive CD4+ T cells exposed to NOR had decreased production of IL-2 compared to unexposed cells; they also reported that this IL-2 decrease was abrogated by 2AR antagonist addition to the culture medium. These results strongly suggest that NOR and β2AR stimulation modify the ability of naive CD4+ T cells to produce cytokines, such as IL-2, that would allow the activated cells to expand in number.
An increased percentage of circulating macrophages presenting major histocompatibility complex II (Kul01/MHCII+) was found in the cold stressed birds on ED21. One possible explanation for this finding includes NOR. Recently, Xiu et al. (Citation2013) examined the effects of NOR on the phenotype and functions of bone marrow-derived macrophages. They found that low NOR doses (<106 M) inhibited MHCII and CCR2 (chemokine receptor type II) expression and bone marrow-derived macrophage proliferation. On the contrary, high NOR doses (>108 M) slightly increased MHCII and CCR2 expressions but inhibited the proliferation and migration of bone marrow-derived macrophage. These results showed that NOR is also able to regulate macrophage differentiation, proliferation and function and may play a critical role in some dysfunctional immune responses, such as those under report.
The stressed and SH-treated birds (PCS group) still had a decreased number of CD3+ lymphocytes compared to PC group birds on ED21. A significant decrease in CD8+ cell numbers was also found on ED21 in birds of the PCS group of this study. Recent work using in vitro NOR treatment and CD8+ T cells (Slota et al., Citation2015) showed a catecholamine-induced reduction in the expression of IFN-γ and IL-2 in NOR treated memory CD8+ T cells; according to these authors, the decreased productions of IL-2 and IFN- γ might have contributed to the modest decrease in the immune cell number found in the NOR-treated cells. These findings suggest that NOR might play a pivotal role in altering the proliferation of memory CD8+ T cells. It seems relevant to remember that corticosterone concentrations were also higher in the cold stressed birds of this group on ED21 and, thus, both stress hormones might have influenced the immune cell data found in this work, as proposed elsewhere in different experimental contexts (Zorrilla et al., Citation2001; Carrasco & Van de Kar, Citation2003).
The impact of stress on immune function is undoubtedly complex. Our results suggest that the present model used, employing cold stress (19 ± 1°C, 6 h /day from ED1 to ED7), activates the HPA-axis and the SNS, leading to the chickens’ immune system dysfunction; these immune system changes would allow increased SH invasion from the gut to systemic circulation and, at the same time, to a decreased clearance of SH from the chickens’ tissue. Notwithstanding, our data also showed that the cold stress per se was able to impair chicken immunity and that SH infection per se might be taken as a stressor agent in chickens. Within this context, it seems that stress prevention is a relevant factor to achieve not only chicken welfare but also safe and profitable poultry production.
Supplemental data
Supplemental data for this article can be accessed at 10.1080/03079457.2015.1086976
Supplementary Data
Download Zip (106.8 KB)Funding
This work was supported by the São Paulo Research Foundation (FAPESP) [grant number 2012/22718-8].
References
- Ando, O., Suemoto, Y., Kurimoto, M., Horikawa, T. & Ichihashi, M. (2000). Deficient Th1-type immune responses via impaired CD28 signaling in ultraviolet B-induced systemic immunosuppression and the restorative effect of IL-12. Journal of Dermatological Science, 24, 190–202. doi: 10.1016/S0923-1811(00)00102-X
- Besedovsky, H.O. & Del Rey, A. (2000). The cytokine-HPA axis feed-back circuit. Zeitschriftfür Rheumatologie, 59, II26–II30. doi: 10.1007/s003930070014
- Besedovsky, H.O., Del Rey, A., Sorkin, E. & Dinarello, C.A. (1986). Immunoregualtory feedback between interleukin-1 and glucocorticoid hormones. Science, 233, 652–654. doi: 10.1126/science.3014662
- Borsoi, A., Santin, E., Santos, L.R., Salle, C.T.P., Moraes, H.L.S. & Nascimento, V.P. (2009). Inoculation of newly hatched broiler chicks with two Brazilian isolates of Salmonella Heidelberg strains with different virulence gene profiles, antimicrobial resistance, and pulsed field gel electrophoresis patterns to intestinal changes evaluation. Poultry Science, 88, 750–758. doi: 10.3382/ps.2008-00466
- Borsoi, A., Santos, L.R., Rodrigues, L.B., Moraes, H.L.S., Salle, C.T.P. & Nascimento, V.P. (2011). Behavior of Salmonella Heidelberg and Salmonella Enteritidis strains following broiler chick inoculation: evaluation of cecal morphometry, liver and cecum bacterial counts and fecal excretion patterns. Brazilian Journal of Microbiology, 42, 266–273. doi: 10.1590/S1517-83822011000100034
- Burkholder, K.M., Thompson, K.L., Einstein, M.E., Applegate, T.J. & Patterson, J.A. (2008). Influence of stressors on normal intestinal microbiota, intestinal morphology, and susceptibility to Salmonella Enteritidis colonization in broilers. Poultry Science, 87, 1734–1741. doi: 10.3382/ps.2008-00107
- Carrasco, A.G. & Van de Kar, L.D. (2003). Neuroendocrine pharmacology of stress. European Journal of Pharmacology, 463, 235–272. doi: 10.1016/S0014-2999(03)01285-8
- Collier, C.R., Hayne, H. & Collier, G. (1997). Behavioral thermoregulation in chicks: the best nest. Developmental Psychobiology, 31, 231–244. doi: 10.1002/(SICI)1098-2302(199712)31:4<231::AID-DEV1>3.3.CO;2-D
- Costa-Pinto, F.A. & Palermo-Neto, J. (2010). Neuroimmune interactions in stress. Neuroimmunomodulation, 17, 196–199. doi: 10.1159/000258722
- Couto-Moraes, R., Palermo-Neto, J. & Markus, R.P. (2009). The immune–pineal axis. Annals of the New York Academy of Sciences, 1153, 193–202. doi: 10.1111/j.1749-6632.2008.03978.x
- Dawkins, M.S., Donnelly, C.A. & Jones, T.A. (2004). Chicken welfare is influenced more by housing conditions than by stocking density. Nature, 427, 342–344. doi: 10.1038/nature02226
- Dunkley, C.S., McReynolds, J.L., Dunkley, K.D., Njongmeta, L.N., Berghman, L.R., Kubena, L.F., Nisbet, D.J. & Ricke, S.C. (2007). Molting in Salmonella Enteritidis-challenged laying hens fed alfalfa crumbles. IV. Immune and stress protein response. Poultry Science, 86, 2502–2508. doi: 10.3382/ps.2006-00401
- Flandreau, E.I., Ressler, K.J., Owens, M.J. & Nemeroff, C.B. (2012). Chronic overexpression of corticotropin-releasing factor from the central amygdala produces HPA axis hyperactivity and behavioral anxiety associated with gene-expression changes in the hippocampus and paraventricular nucleus of the hypothalamus. Psychoneuro endocrinology, 37, 27–38. doi: 10.1016/j.psyneuen.2011.04.014
- Foley, S.L., Nayak, R., Hanning, I.B., Johnson, T.J., Han, J. & Ricke, S.C. (2011). Population dynamics of Salmonella enterica serotypes in commercial egg and poultry production. Applied and Environmental Microbiology, 77, 4273–4279. doi: 10.1128/AEM.00598-11
- Glaser, R. & Kiecolt-Glaser, J.K. (2005). Stress-induced immune dysfunction: implications for health. Nature Reviews Immunology, 5, 243–251. doi: 10.1038/nri1571
- Glavin, G.B. (1985). Stress and brain noradrenaline: a review. Neuroscience & Behavioral Reviews, 9, 233–243. doi: 10.1016/0149-7634(85)90048-X
- Gomes, A.V.S., Quinteiro-Filho, W.M., Ribeiro, A., Ferraz-de-Paula, V., Pinheiro, M.L., Baskeville, E., Akamine, A.T., Astolfi-Ferreira, C.S., Ferreira, A.J.P. & Palermo-Neto, J. (2014). Overcrowding stress decreases macrophage activity and increases Salmonella Enteritidis invasion in broiler chickens. Avian Pathology, 43, 82–90. doi: 10.1080/03079457.2013.874006
- Hayley, S., Borowski, T., Merali, Z. & Anisman, H. (2001). Central monoamine activity in genetically distinct strains of mice following a psychogenic stressor: effects of predator exposure. Brain Research, 892, 293–300. doi: 10.1016/S0006-8993(00)03262-5
- Herman, J.P., McKlveen, J.M., Solomon, M.B., Carvalho-Netto, E. & Myers, B. (2012). Neural regulation of the stress response: glucocorticoid feedback mechanisms. Brazilian Journal of Medical and Biological Research, 45, 292–298. doi: 10.1590/S0100-879X2012007500041
- Hill, D. (2011). The early brooding period: Issues and a new technology solution. Retrieved from http://vet.uga.edu/images/uploads/pdrc/PIP%20July-Aug%2011%20Final.pdf
- Hoerr, F.J. (2010). Clinical aspects of immunosuppression in poultry. Avian Diseases, 54, 2–15. doi: 10.1637/8909-043009-Review.1
- Hugas, M. & Beloeil, P.A. (2014). Controlling Salmonella along the food chain in the European Union-progress over the last ten years. Eurosurveillance. Retrieved from http://www.eurosurveillance.org/ViewArticle.aspx?ArticleId=20804
- Humphrey, T. (2006). Are happy chickens safer chickens? Poultry welfare and disease susceptibility. British Poultry Science, 47, 379–391. doi: 10.1080/00071660600829084
- Kin, N.W. & Sanders, V.M. (2006). It takes nerve to tell T and B cells what to do. Journal of Leukocyte Biology, 79, 1093–1104. doi: 10.1189/jlb.1105625
- Lay, D.C., Fulton, R.M., Hester, P.Y., Karcher, D.M., Kjaer, J.B., Mench, J.A., Mullens, B.A., Newberry, R.C., Nicol, C.J., O'Sullivan, N.P. & Porter, R.E. (2011). Hen welfare in different housing systems. Poultry Science, 90, 278–294. doi: 10.3382/ps.2010-00962
- Lyte, M., Vulchanova, L. & Brown, D.R. (2011). Stress at the intestinal surface: catecholamines and mucosa–bacteria interactions. Cell and Tissue Research, 343, 23–32. doi: 10.1007/s00441-010-1050-0
- MAPA. (2003). Diario Oficial da Uniao. Instrução Normativa, SDA No. 62, de 26 de agosto de 2003. Retrieved from http://extranet.agricultura.gov.br/sislegis-consulta/consultarLegislacao.do?operacao=visualizar&id=2851
- Pardon, M.C., Gould, G.G., Garcia, A., Phillips, L., Cook, M.C., Miller, S.A., Mason, P.A. & Morilak, D.A. (2002). Stress reactivity of the brain noradrenergic system in three rat strains differing in their neuroendocrine and behavioral responses to stress: implications for susceptibility to stress-related neuropsychiatric disorders. Neuroscience, 115, 229–242. doi: 10.1016/S0306-4522(02)00364-0
- Pardon, M.C., Shuaike, M.A. & Morilak, D.A. (2003). Chronic cold stress sensitizes brain noradrenergic reactivity and noradrenergic facilitation of the HPA stress response in Wistar Kyoto rats. Brain Research, 971, 55–65. doi: 10.1016/S0006-8993(03)02355-2
- Petrella, C., Giuli, C., Agostini, S., Bacquie, V., Zinni, M., Theodorou, V., Broccardo, M., Casolini, P. & Improta, G. (2014). Maternal exposure to low levels of corticosterone during lactation protects against experimental inflammatory colitis-induced damage in adult rat offspring. PLoS One, 9, e113389. doi: 10.1371/journal.pone.0113389
- Powell, N.D., Tarr, A.J. & Sheridan, J.F. (2013). Psychosocial stress and inflammation in cancer. Brain, Behavior, and Immunity, 30, S41–S47. doi: 10.1016/j.bbi.2012.06.015
- Pullinger, G.D., Carnell, S.C., Sharaff, F.F., Van Diemen, P.M., Dziva, F., Morgan, E., Lyte, M., Freestone, P.E.P. & Stevens, M.P. (2010). Norepinephrine augments Salmonella enterica-induced enteritis in a manner associated with increased net replication but independent of the putative adrenergic sensor kinases QseC and QseE. Infection and Immunity, 78, 372–380. doi: 10.1128/IAI.01203-09
- Quinteiro-Filho, W.M., Gomes, A.V., Pinheiro, M.L., Ribeiro, A., Ferrazde-Paula, V., Astolfi-Ferreira, C.S., Ferreira, A.J. & Palermo-Neto, J. (2012). Heat stress impairs performance and induces intestinal inflammation in broiler chickens infected with Salmonella Enteritidis. Avian Pathology, 41, 421–427. doi: 10.1080/03079457.2012.709315
- Quinteiro-Filho, W.M., Ribeiro, A., Ferraz-de-Paula, V., Pinheiro, M.L., Sakai, M., Sa, L.R., Ferreira, A.J. & Palermo-Neto, J. (2010). Heat stress impairs performance parameters, induces intestinal injury, and decreases macrophage activity in broiler chickens. Poultry Science, 89, 1905–1914. doi: 10.3382/ps.2010-00812
- Roshchina, V.V. (2010). Evolutionary considerations of neurotransmitters in microbial, plant, and animal cells. In M. Lyte & P.P.E. Freestone (Eds.), Microbial Endocrinology, Interkingdom Signaling in Infectious Disease and Health (pp. 17–52). New York: Springer.
- Slota, C., Chi, A., Chen, G., Bevans, M. & Weng, N.P. (2015). Norepinephrine preferentially modulates memory CD8 T cell function inducing inflammatory cytokine production and reducing proliferation in response to activation. Brain, Behavior, and Immunity, 46, 168–179. doi: 10.1016/j.bbi.2015.01.015
- Tachibana, T., Oikawa, D., Takahashi, H., Boswell, T. & Furuse, M. (2007). The anorexic effect of alpha-melanocyte-stimulating hormone is mediated by corticotrophin-releasing factor in chicks. Comparative Biochemistry and Physiology Part A: Molecular & Integrative Physiology, 147, 173–178. doi: 10.1016/j.cbpa.2006.12.044
- Tetsuya, A., Brown, R.F., Berg, R.D. & Dunn, A.J. (2000). Bacterial translocation can increase plasma corticosterone and brain catecholamine and indoleamine metabolism. American Journal of Physiology - Regulatory Integrative Comparative Physiology, 279, 2164–2172.
- Tsigosa, C. & Chrousosb, G.P. (2002). Hypothalamic–pituitary–adrenal axis, neuroendocrine factors and stress. Journal of Psychosomatic Research, 53, 865–871. doi: 10.1016/S0022-3999(02)00429-4
- Viertlboeck, B. & Göbel, T.W.F. (2008). Avian T cells: Antigen recognition and lineages. In F. Davison, B. Kaspers & K.A. Schat (Eds.), Avian Immunology (1st ed., pp. 91–107). San Diego, CA: Academic Press Elsevier.
- Xiu, F., Stanojcic, M. & Jeschke, M.G. (2013). Norepinephrine inhibits macrophage migration by decreasing CCR2 expression. PLoS One, 8, e69167. doi: 10.1371/journal.pone.0069167
- Zorrilla, E.P., Luborsky, L., McKay, J.R., Rosenthal, R., Houldin, A., Tax, A., McCorkle, R., Seligman, D.A. & Schmidt, K. (2001). The relationship of depression and stressors to immunological assays: a meta-analytic review. Brain, Behavior, and Immunity, 15, 199–226. doi: 10.1006/brbi.2000.0597