Abstract
In order to investigate the pancreatic lesions caused by the infection with either H7N1 or H7N3 low-pathogenicity avian influenza viruses, 28 experimentally infected turkeys were submitted for histopathology, immunohistochemistry, haematobiochemistry and real-time reverse transcriptase polymerase chain reaction after different days post-infection (DPI). The localization of viral antigen and the measurement of insulin and glucagon expression in the pancreas were assessed to verify the progression from pancreatitis to metabolic disorders, such as diabetes. At the early infection phase (4–7 DPI), a severe acute necrotizing pancreatitis was recognized. During the intermediate phase (8–17 DPI), a mixed acute/chronic change associated with regenerative ductular proliferation was observed. A loss of pancreatic islets was detected in most severe cases and viral antigen was found in the pancreas of 11/28 turkeys (4–10 DPI) with the most severe histological damage. In turkeys euthanized at 39 DPI (late phase), a chronic fibrosing pancreatitis was observed with the reestablishment of both the exocrine and the endocrine pancreas. Insulin and glucagon expression manifested a progressive decrease with subsequent ductular positivity. Haematobiochemistry revealed increased lipasemia in the first week post-infection and hyperglycaemia in the second, with a progressive normalization within 21 DPI. This study allowed the identification of progressive virus-associated exocrine and endocrine pancreatic damage, suggesting that influenza virus might be responsible for metabolic derangements. Moreover, it highlighted a remarkable post-damage hyperplastic and reparative process from a presumptive common exocrine/endocrine precursor. This potential regeneration deserves further investigation for its relevance in a therapeutic perspective to replace lost and non-functional cells in diabetes mellitus.
Introduction
Influenza viruses originating from birds are called avian influenza viruses (AIVs). Avian influenza is a very contagious disease which may cause illness and death in domestic poultry and wild birds. Two pathotypes of AIV are known, the highly pathogenic pathotype (HPAIV) which causes high mortality rates in gallinaceous birds, and the low pathogenicity pathotype (LPAIV) that may be asymptomatic in most avian species or cause a mild to moderate-severe illness, the latter particularly in turkeys. However, following the acute phase and convalescence, and in the absence of severe secondary infections, these birds fully recovered, to the point of reaching in some cases standard zootechnical performances at slaughter (Terregino, personal communication). To date, AIVs affecting avian species are serologically classified into subtypes according to the antigenic properties of the two main surface glycoproteins: the haemagglutinin and the neuraminidase (Alexander & Brown, Citation2009). In the past decade, increasing interest on AIV infections has been mainly attributed to the recurrent epidemics of H5 and H7 HPAIVs in poultry, worldwide. These two subtypes are related to severe cases of influenza in poultry with massive mortalities, representing also a potential threat to humans (1997–2013 outbreaks) (Poovorawan et al., Citation2013). Outbreaks of H5 and H7 LPAIVs continue to occur in poultry, resulting in direct economic losses as well as trade restrictions (Molesti et al., Citation2012).
Necrotizing pancreatitis is a common finding in wild and domestic birds infected with HPAIV (Tanimura et al., Citation2006; Teifke et al., Citation2007; Bertran et al., Citation2011); LPAIV infections of chickens and turkeys are accompanied by pancreatic damage as well (Capua & Mutinelli, Citation2001a; Capua et al., Citation2013). The metabolic consequences of such infection, potentially contributing to temporary reduction in zootechnical performances and secondary economic loss, have not been thoroughly investigated.
There are many other viral infections responsible for the development of acute or chronic pancreatitis in birds aside from AIVs, such as avian paramyxovirus (Johnston & Key, Citation1992; Nakamura et al., Citation2008), Psittacid herpesvirus 1 (Phalen et al., Citation2007), coronavirus (Qian et al., Citation2006), and West Nile virus (Palmieri et al., Citation2011). In all these cases, the pathological features of pancreatitis range from an acute necrotizing form to a chronic multifocal lymphocytic pancreatitis, with or without secondary involvement of the endocrine part (Johnston & Key, Citation1992; Qian et al., Citation2006; Phalen et al., Citation2007; Nakamura et al., Citation2008; Palmieri et al., Citation2011). No information on the metabolic consequences of this damage is currently available.
The avian pancreas lies between the ascending and descending duodenum. The endocrine portion, in comparison with the exocrine portion, is larger in birds than in mammals and secretes its hormones in the portal vein, loaded with absorbed nutrients in the postprandial period (Hazelwood, Citation2000). In birds there are three types of islets described: the dark islets, the light islets, and mixed islets. Dark islets are composed of A and D cells, light islets are composed of B and D cells, and mixed islets contain all three cell types: A, B, and D cells (Hazelwood, Citation2000; Ritchie & Pilny, Citation2008). A cells synthesize and release glucagon (G); B cells synthesize and release insulin (I); D cells secrete somatostatin. A fourth type, named F or PP cell, produces and releases the pancreatic polypeptide. Avian I is an anabolic hormone, more powerful than mammalian I in stimulating glycogenesis and controlling glycaemia; avian G is a catabolic hormone and circulates at levels up to eight times higher than in humans (Hazelwood, Citation2000; Ritchie & Pilny, Citation2008). The mechanism of glucose homeostasis is the same as in mammals: a raise in blood glucose stimulates pancreatic B cell activity, leading to the release of I, while a decrease in glycaemia triggers the release of G (Pilny, Citation2008). However, some authors believe that in granivorous birds, I might play a minor role in glucose regulation and that diabetes may be caused by the dysregulation of G levels (Pilny, Citation2008). The normal level of plasma glucose in different species of birds ranges from 180 to 350 mg/dL (Hazelwood, Citation2000; Ritchie & Pilny, Citation2008). As in other species, birds may develop transient hyperglycaemia when under acute stress (Hazelwood, Citation2000; Ritchie & Pilny, Citation2008). In case of pancreatic damage, a transient (or reversible) hyperglycemic condition is observed in granivorous birds, in which normal glycaemia can be reestablished by regeneration (Laurent & Mialhe, Citation1978; Hoffmann & Solter, Citation2008).
The present study was developed to i) investigate the tissue damage in the pancreas after experimental infection with two LPAIVs of the H7 subtype in young turkeys; ii) analyse the localization of the viral antigen within the different subtypes of pancreatic cells; and iii) measure I and G expression in the pancreas during the infection in order to characterize the progression from pancreatitis to metabolic disorders associated with LPAIVs infections.
Materials and Methods
In vivo experiment
The study was performed in accordance with the relevant national and local animal welfare bodies [Convention of the European Council no. 123 and National Guidelines (Legislative Decree 116/92)]. The protocol was approved by the Committee on the Ethics of Animal Experiments of the Istituto Zooprofilattico Sperimentale delle Venezie (IZSVe) (permit number CE.IZSVE.022012). For the specific purpose of this study, we analysed 28 pancreases collected from young female turkeys infected with LPAIVs. Two weeks before infection, birds were housed in negative-pressure, HEPA-filtered isolation cabinets (Montair® HM 1900 Kronenberg, the Netherlands) to allow adaptation and were kept there for the whole duration of the experimental trial, receiving food and water ad libitum.
Three-week-old birds were infected via the oro-nasal route with two LPAIVs isolated during the epidemics in Italy. Seventeen and 11 subjects were challenged via the oral-nasal route with 0.1 mL of allantoic fluid containing 106.8 EID50 of the A/turkey/Italy/3675/1999 (H7N1) virus and 106.4 EID50 of the A/turkey/Italy/2962/2003 (H7N3) virus, respectively (). In a previous study, both viruses had been associated with pancreatic lesions in infected birds (Capua et al., Citation2013). Negative controls (two birds) received 100 µl of negative allantoic fluid by the same route. All birds were observed twice a day for clinical signs. Blood was collected from the brachial veins on 0, 7, 14, and 21 days post-infection (DPI) using heparinized syringes to determine glucose and lipase levels in plasma, and non-heparinized syringes to calculate the time frame for seroconversion. Tracheal and cloacal swabs were collected from all birds on 2, 3, and 4 DPI to evaluate viral replication and confirm active infection.
Table 1. Turkeys included in the experimental infection with AIVs and results of blood biochemistry, virus detection, and histology and IHC on pancreas.
Pathological study
A pre-established number of birds belonging to the infected groups were humanely sacrificed on 4 (n = 8), 7 (n = 5), 8 (n = 2), 9 (n = 3), 10 (n = 2), 17 (n = 2), and 39 (n = 6) DPI (see ). Subjects were grouped in three phases of infection as early (4 and 7 DPI), intermediate (8, 9, 10, and 17 DPI), and late (39 DPI). The two controls were humanely sacrificed on days 10 and 17 post-infection (PI). Pancreas was sampled for histopathology and immunohistochemistry (IHC) immediately after death; pancreas samples of subjects sacrificed at 4, 7, 8, and 10 DPI were collected also for the detection of viral RNA.
Gross and histological examination
Necropsies were performed according to standards and major lesions of visceral organs were recorded. Formalin-fixed, paraffin-embedded samples of pancreas were prepared and stained with haematoxylin and eosin (HE). For collagen deposits and extracellular matrix evaluation, additional sections from pancreatic samples were stained with Masson's thrichrome; Congo-red staining was performed on selected cases to eventually identify amyloid deposits.
Immunohistochemistry
All pancreatic samples were stained with an automated immunostainer (Benchmark, Ventana Medical Systems Inc. Tucson, AZ, USA) for I (mouse anti-human insulin E2E3 NB120–9569, Novus Biologicals Littleton, CO, USA; 1:50, 2 h at 37°C) and G (rabbit anti-human glucagon, Dakocytomation (Dako Products, Carpinteria, CA, USA); 1:800, 32 min at room T°) expression and by manual IHC for AIV (mouse anti-influenza A NP subtype A, clones EVS 238, European Veterinary Laboratory; 1:100, 1 h at room T°). Sections (4 µm) were mounted on Superfrost Plus microscope slides (Menzel GmbH, Braunschweig, Germany) and dried at 37°C for 30 min. The immunostainer operations included dewaxing and rehydration, antigen retrieval for I (30 min at 95°C, CC1 reagent, Ventana Medical System Inc.), primary antibody incubation, antigen detection with ultraView™ Universal DAB kit (Ventana Medical Systems), and counterstaining with Mayer's haematoxylin. Primary antibody dilutions were performed using a commercial antibody diluent (Ventana Medical Systems) and negative controls were obtained omitting the primary antibody that was replaced by the same diluent. The manual protocol for AIV expression was performed as previously reported (Capua et al., Citation2013). Finally, all slides were manually dehydrated through a graded series of alcohols and mounted using Eukitt mounting medium (Electron Microscopy Sciences, Fort Washington, PA, USA).
Section evaluation
Two operators evaluated all sections blind using an Olympus BX-40 light microscope (Olympus,Tokyo, Japan). At histopathology, a scoring system of morphological changes reflecting the severity of acute and chronic pancreatitis, respectively, was applied according to the semi-quantitative system of De Cock and co-authors () (De Cock et al., Citation2007). The evidence of islets of Langerhans as detectable at histology was also recorded. Immunohistochemical expression was assessed as brown discoloration of nuclei and cytoplasm for the viral protein and only of cytoplasm for I and G. A semi-quantitative analysis was performed for I and G expression and the area of positive cells was measured for each section by a semi-automatic system. The ratio of the positive total area for each marker and the total area of the tissue was also registered (D-sight, Menarini Diagnostic, Florence, Italy).
Table 2. De Cock scoring system used to classify acute and chronic pancreatitis.
Statistical analysis
Data regarding morphological changes and IHC expressions were analysed by the SPSS system (IBM Corporation Armonk, NY, USA) applying the Kruskal–Wallis, the Wilcoxon, and the Mann–Whitney tests. The level of significance was P < 0.05.
Blood biochemistry and serological assay
Blood samples were collected in Gas Lyte 23 G paediatric syringes containing lyophilized lithium heparin in experimental time points stated above in this section. At each sampling time, 300 µl of blood was collected and refrigerated at 4°C until processing. To obtain plasma, samples were immediately centrifuged at 1500 × g for 15 min at 4°C. To determine the levels of glucose and lipase in plasma, commercially available kits were applied to the computerized system Cobas c501 as previously described elsewhere (Capua et al., Citation2013). A commercial competitive ELISA (ID Screen® influenza A Antibody Competition Multi-Species IDvet, Grabels, France) and the haemagglutination inhibition (HI) test (OIE, Citation2014) were applied to detect type- and subtype-specific antibodies.
Molecular tests
Viral RNA was extracted from 50 µl of phosphate-buffered saline (PBS) containing a swab suspension using the Ambion MagMax-96 AI-ND Viral RNA Isolation Kit (Thermo Scientific™, Milan, Italy) for the automatic extractor. One hundred fifty milligrams of homogenized pancreas tissues collected immediately after the death of the birds was centrifuged, and viral RNA was extracted from 100 µl of clarified suspension using the NucleoSpin RNA II kit (Macherey-Nagel, Düren, Germany). Real-time reverse transcriptase polymerase chain reaction (RRT-PCR) for the identification of the influenza virus matrix (M) gene was used in order to test pancreas for the presence of viral RNA. The one-step RRT-PCR was performed as previously described (Capua et al., Citation2013) applying the previously published primers and probes (Spackman et al., Citation2002).
Results
Clinical signs, gross and histopathological observations
Turkeys from both H7N1-challenged (A) and H7N3-challenged (B) groups showed clinical signs typical of LPAI infection such as reluctance to move, inappetence, and depression, accompanied by mild respiratory signs such as rales and snicking. Some birds developed mild dyspnoea and/or swelling of the infraorbital sinuses.
No differences were noted between the H7N1- and the H7N3-infected groups at any level (gross, histological, IHC, serological or molecular analyses), as already reported (Capua et al., Citation2013). At necropsy, pancreatic gross lesions were evident in 22/28 turkeys. The surface of the pancreas appeared non-homogeneous with a reddish discoloration in subjects sacrificed before 10 DPI. This was associated with fibrin deposition on the serosal surfaces. In samples collected after 10 DPI, more compact whitish multifocal degenerative/necrotic areas were noted. Pneumonia was also detected in the infected birds euthanized on 4, 9, and 10 DPI. None of the control turkeys showed evident gross lesions in visceral organs.
At histology, pancreatic lesions were confirmed in 22/28 infected animals, considering at least one of the assessed changes, and ranged from mild to severe (). Morphological changes of the pancreas () were significantly different according to the phase of infection ( and ). An acute necrotizing pancreatitis and fibrinous peripancreatitis ((a)) with heterophilic interstitial inflammation, oedema, and necrosis were especially evident in the early phase (4 and 7 DPI) in 8/13 birds. A chronic fibrosing pancreatitis with ductular hyperplasia ((b)) was observed predominantly in turkeys from 8 to 17 DPI (intermediate phase) (8/9 subjects) as confirmed also by moderate to severe fibrosis on Masson Trichrome staining. In addition, a striking feature of the intermediate phase was the diffuse presence of irregularly branching tubular-like ectatic and hyperplastic structures consistent with ductular hyperplasia ((b)). At 9 DPI, in one bird the lesions were associated with a massive deposition of an eosinophilic extracellular collagenous non-amyloid (Congo-red negative) matrix. Variable fibrosis with lymphoplasmacytic pancreatitis and areas of normal pancreatic tissue ((c)) were observed in turkeys euthanized in the late phase (39 DPI) (6/6 subjects).
Figure 1. Turkey, pancreas, histological lesions at different infection phases with AIVs. (a) The normal anatomy of the pancreas in a control turkey. During the early phase (4–7 DPI) (b) an acute necrotizing pancreatitis with heterophilic interstitial inflammation (*), oedema, and necrosis was evident, whereas the main lesions of the intermediate phase (8–17 DPI) (c) consisted of a chronic fibrosing pancreatitis with ductular hyperplasia (§). During the late phase (39 DPI), (d) fibrosis and lymphoplasmacytic infiltrate (@) were observed together with areas of normal pancreatic tissue. ((a) case no. 30, (b) case no. 8, (c) case no. 20, (d) case no. 26. HE). bar = 50 µm.
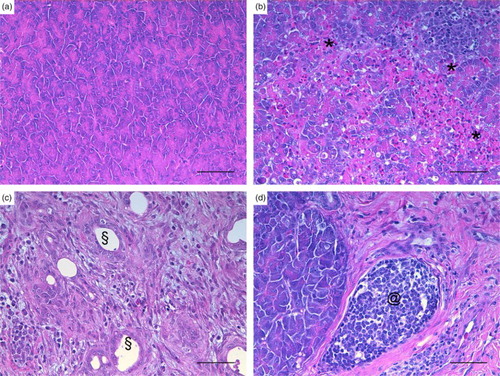
Figure 2. Main histological lesions at different infection phases with AIVs. Different letters show statistical significance (Mann–Whitney test, P < 0.05).
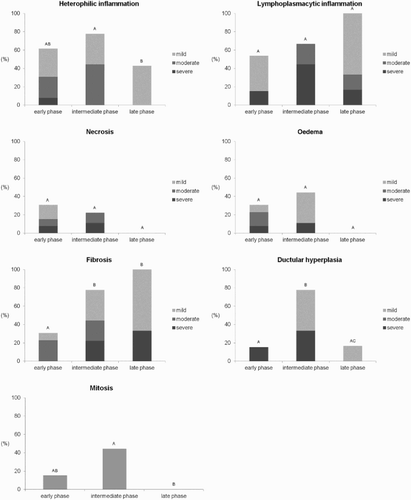
Lesions in the control animals were non-significant, being consistent with a minimal disseminated heterophilic and lymphocytic infiltration.
The application of the score for pancreatitis on HE and Masson's thrichrome-stained slides revealed () that only subjects (4/8 at 4 DPI) in the early phase had signs of pure acute pancreatitis, whereas pure chronic pancreatitis was predominant in the late phase (5/6 at 39 DPI). Mixed signs of acute and chronic inflammation were evident for the other 13 subjects. Subjects without histological pancreatic lesions (6/28) were also negative for virus detection in the pancreas and were euthanized in the early (5/6) or in the intermediate (1/6) phase post-infection.
Pancreatic islets were not microscopically discernible in 8/28 subjects, particularly in those with severe damage in the early (3/8) or the intermediate phase (5/8) ().
Immunohistochemistry
IHC analysis revealed expression of I (28/28) and G (27/28) throughout the post-infection course in nearly all turkeys (and in controls), including cases of severe and diffuse necrotizing pancreatitis (). However, distribution and quantification of I/G-producing cells showed interesting variations according to the phase of infection () and type of histological damage (). Specifically, for both hormones, even if more evident for G, positive cells were less numerous and smaller during early and intermediate phases of infection compared to the late phase ( and and ) (P < 0.05). Moreover, both hormones were expressed significantly (P < 0.05) more in the chronic rather than in the acute lesions, and G-positive areas were significantly larger than I-positive areas in the chronic phase ( and ). Furthermore, positivity to both hormones was detected within cells of the newly formed ductular structures ((b)).
Figure 3. Turkey, pancreas, glucagon, and insulin IHC expression at different infection phases with AIVs Glucagon and insulin expression, shown during the early (a), intermediate (b), late phases (c), and control (d) phases, were preserved throughout the post-infection course in nearly all turkeys, although positive areas were less numerous and smaller in the early and intermediate phases and larger in the last. Some positive cells were also evident within ductular cells (b). (glucagon: A case no. 1, B case no. 20, C case no. 26, D case no. 30; insulin: A case no. 1, B case no. 20, C case no. 27, D case no. 30. Glucagon and insulin IHC, DAB chromogen, Haematoxylin counterstain) bar = 50 µm.
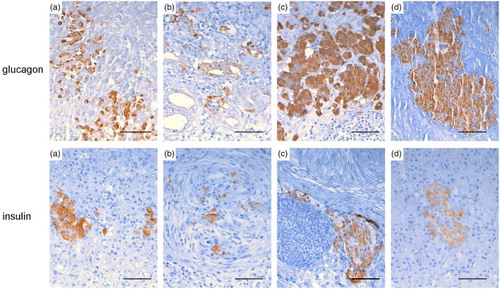
Figure 4. Ratio of the glucagon- and insulin-positive area on the total area at different infection phases. Different letters show statistical significance (Mann–Whitney test, P < 0.05).
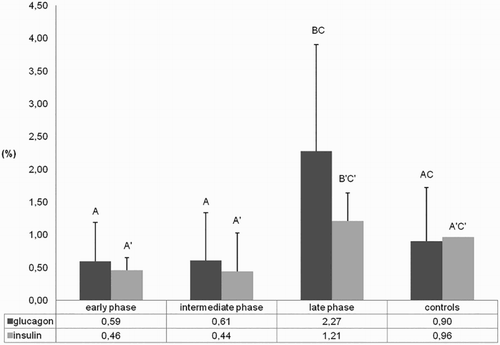
Figure 5. Ratio of the glucagon- and insulin-positive area on the total area in relation to different types of pancreatic damage. Different letters show statistical significance (Mann–Whitney test, P < 0.05).
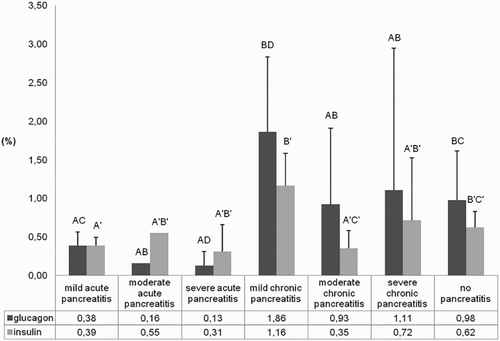
In six out of 28 subjects (21.4%) – 4/6 from the early phase and 2/6 from the intermediate phase – the presence of the viral nucleoprotein was detected within the nuclei and the cytoplasm of both necrotic and intact exocrine pancreatic cells, as well as in some ductal cells () and occasionally in endothelial cells. The viral positivity was detected in cases with severe changes (score 2 or 3), thus indicating a potential correlation. No other cells (inflammatory and fibroblasts) were clearly positive for the virus antigen. Birds sacrificed from 10 DPI onwards and controls were negative for influenza nucleoprotein () in both the exocrine and endocrine portions ((b)).
Figure 6. Turkey, pancreas, avian influenza A virus expression. Presence of the viral nucleoprotein was detected within the nuclei and the cytoplasm of both necrotic and intact exocrine pancreatic cells and also of some ductal cells (a). Islet cells, when detectable, appeared negative (b). (A case no. 11, B case no. 8. AIV IHC, DAB chromogen, Haematoxylin counterstain) bar = 50 µm.
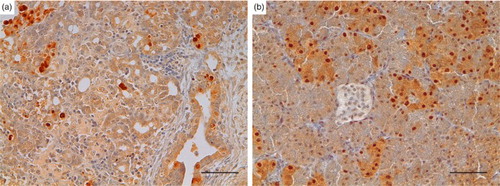
Blood biochemistry and serological assay
Birds were found to be seropositive for AI type A and for H7 subtype since 7 and 14 DPI, respectively. HI titres increased proportionally with the duration of infection (data not shown). In order to identify metabolism alteration in our cohort of turkeys (), the ranges of lipasemia and glycaemia in healthy turkeys published by Capua and co-authors in 2013 were used as standard, in particular 4.1 ± 1.5 U/L for lipasemia and 15.1 ± 1.7 mmol/L for glycaemia (Capua et al., Citation2013). Increase in lipase was considered significant when it was at least 10 times higher than the average of controls, whereas a significant increase in glycaemia was fixed if >17mmol/L. Increased lipasemia was detected in 7/18 subjects at DPI 7, whereas glycaemia slightly increased after the challenge and up to 7 DPI, reaching a peak in 5/8 subjects at DPI 14. The high increase in lipasemia showed evidence of resolution at DPI 14. Normalization of glycaemia was evidenced at DPI 21.
Molecular analysis
Tracheal and cloacal swabs were positive at 2 DPI in almost all animals (25/28). At 3 and 4 DPI all swabs showed positive results, confirming viral replication and active infection.
Viral RNA was amplified in 10 out of 17 (58.8%) pancreas from the early (6/10) and the intermediate (4/10) post-infectious phases. Only 2/7 negative subjects (nos 6 and 7) showed pancreatic lesions (mild acute pancreatitis). Cases with lower Ct values (higher AIV RNA positivity) were associated with positive IHC expression. No viral RNA was detected from the uninfected controls.
Discussion
The aim of this study was to describe in detail the morphological features of pancreatic damage in turkeys infected by two LPAIVs, investigating also the localization of the viral antigen within pancreatic cell subtypes and evaluating I and G expression in the pancreas to monitor the potential metabolic consequences of the infection.
As is known, AIVs cause systemic or non-systemic infections according to the viral strain involved. The systemic disease in HPAI infections is characterized by rapid viral replication with multiorganic failure and by sudden death of the animals some few days after the infection. As a consequence of the systemic replication, necrotizing pancreatitis is a common finding of HPAIV infections (Abolnik et al., Citation2009; Tanimura et al., Citation2006; Teifke et al., Citation2007; Bertran et al., Citation2011). Conversely, respiratory and enteric signs mainly characterize the non-systemic LPAI infections. The pathogenetic mechanism behind the pancreatic colonization in chickens and turkeys infected with LPAIVs is poorly understood (Condobery & Slemons, Citation1992; Shinya et al., Citation1995; Mutinelli et al., Citation2003; Zanella, Citation2003; Okamatsu et al., Citation2006; Morales et al., Citation2009).
In our experiment we recorded pancreatic involvement as a common finding, with no significant differences between the two LPAIVs. The histopathological lesions progressed from acute, to mixed, to chronic with a significant compensatory regenerative ductular capability, particularly evident in the intermediary post-infection phase.
In the early and intermediate phases of infection, turkeys in which high AIV RNA positivity (low RRT-PCR CT values) as well as the presence of viral antigen detectable by IHC were identified, severe histological lesions were observed (scores 2 and 3), thus suggesting a possible association between a high viral amount and severe tissue damage. Given the non-systemic nature of this infection, it is unclear how the viruses have reached the pancreas. This could have presumably occurred either through ductal reflux from the gut or through the blood, following extensive replication in the respiratory and enteric tracts. The former has already been suggested (Oughton et al., Citation2011; Tse et al., Citation2011), and is substantiated by viral positivity in the endothelium and the ducts.
By day 39 (late phase), the pancreatic lesions appeared less severe, with a significant component of both endocrine and exocrine functional tissue, suggesting a transient damage with subsequent regeneration (Hoffmann & Solter, Citation2008).
Viral antigen was detected in six cases from day 4 to day 9, both in necrotic and viable acinar cells and in some pre-existing and newly formed ductal cells. The low number of pancreas positive by IHC may be due to the low sensitivity of the method used. By comparing the data of IHC with those of RRT-PCR (see ), we noticed that it was possible to identify the viral antigen only in the presence of high amount of virus in the pancreas tissue (low RRT-PCR CT values). Similarly also RRT-PCR on the pancreas of infected animals might have resulted in some falsely negative cases because of the inhibitory factors in this tissue. As a matter of fact, intact RNA extraction from the pancreas is very difficult due to the high level of its ribonucleases (RNases). Despite the improvement in several approaches, including rapid removal of pancreatic tissue from the abdominal cavity and homogenization at cold temperatures to inhibit RNases, the isolation of intact, high-quality RNA from this tissue remains challenging because of the complexity and indefinite reproducibility of the above-mentioned techniques (Gill et al., Citation1996; Dastgheib et al., Citation2014).
In the pancreas which presented the viral antigen at standard histology, islets were either absent (5/6) or just slightly evident (1/6). In this condition, it was impossible to determine whether pancreatic islet cell death was a consequence of virus infection or secondary to extensive exocrine inflammatory process and fibrosis. Human influenza viruses have been shown to infect in vitro human pancreatic beta cells (Capua et al., Citation2013); therefore, a similar capability could be hypothesized for AIVs. As demonstrated by I/G IHC results, well-differentiated islets manifested a progressive post-infection reduction and a subsequent regrowth. The I/G positivity decreased particularly in the intermediate phase, and even in cases of moderate-to-severe damage, it rarely disappeared. In this phase, positive cells were disseminated in the pancreatic tissue and associated with scattered positivity in the proliferative ductules. The G-secreting portion was often larger than the I-secreting area as expected in poults. In birds, G seems to play a relevant role for glucose metabolism, proving to be eight times higher than in mammals and, according to some authors, also higher than I (Hazelwood, Citation1984; Pilny, Citation2008). I instead could respond to G variations but not be just governed by the pancreas, and plays a less important role (Pilny, Citation2008; Ritchie & Pilny, Citation2008).
To monitor the tissue damage in relation to the endocrine metabolic derangement, blood biochemistry was performed in a subset of cases at one-week intervals. A significant increase in lipasemia was evident at 7 DPI in subjects that manifested a chronic pancreatic damage (scores 2 and 3). Blood lipase levels are considered as an indicator of pancreatic damage (Hoffmann & Solter, Citation2008). In our samples, lipase concentrations normalized by 14 DPI in agreement with the reparative and regenerative process of the exocrine pancreas. Mild hyperglycaemia was detected in many subjects before the infection, possibly due to the stress consequent to handling and blood sampling. Interestingly, an increase in glycaemia was detected at 14 DPI. This finding was in keeping with the results reported by Capua et al. (Citation2013). Curiously, two cases showed high levels of glycaemia on DPI 14, without prior increase in lipase levels. Such a contradictory result could be interpreted, speculating that the collection of blood at 1-week interval could have missed a temporary increase in lipase levels between 7 and 14 DPI.
It is intriguing to have noticed recent human cases of pancreatic damage associated with human influenza A virus infection, including both acute pancreatitis and the onset of type 1 diabetes (Blum et al., Citation2010; Cano et al., Citation2010; Calore et al., Citation2011; Nenna et al., Citation2011; Watanabe, Citation2011). Type 1 diabetes is a complex disease in which different factors might reduce the overall functional mass of I-secreting cells (Pipi et al., Citation2014); we therefore hypothesize that also in our avian samples the destruction of I-producing islets could be responsible of the hyperglycaemic state. However, control of glycaemia in birds recognizes some differences from that in humans and suffers more complex and partially still unrevealed mechanisms. G seems to play a major role in avian species, in particular during fasting, in which the metabolism switches from I to G secretion (Laurent & Mialhe, Citation1978; Hoffmann & Solter, Citation2008). As previously described in turkeys infected with LPAIV, our subjects affected by avian influenza showed systemic malaise, depression, and reluctance to feed; we can therefore suppose that the reduced food intake and the malabsorption caused by viral enteritis and pancreatitis (Capua & Mutinelli, Citation2001b) have led to further reduction in glucose intake. In this condition, blood glucose levels dropped and glucagon was secreted stimulating hepatic glycogenolysis and the consequent release of newly synthetized glucose into the bloodstream. There is also a second or concomitant hypothesis that could explain the manifested hyperglycaemia. We can speculate that the progressive pancreatic regeneration evidenced from 7 DPI produces at first a predominance of new G-secreting cells with an overproduction of G. G production in this case would be responsible for the observed hyperglycaemia followed by a feedback control via I-secretion from the newly available cells and, possibly, from outside the pancreas (Pilny, Citation2008; Ritchie & Pilny, Citation2008).
Hyperglycaemia was transient and manifested a decreasing trend after one week (21 DPI), and a re-establishment of functional endocrine tissue was indicated by both histology and I/G IHC.
The ability to completely re-establish endocrine and exocrine pancreatic functions from a residual portion as small as 1% has been documented in birds (Laurent & Mialhe, Citation1978; Hoffmann & Solter, Citation2008). This amazing compensatory potential of the pancreas in poults was here substantiated by the extent of ductular proliferation also in severely damaged samples and in association with viral positivity in newly formed cells. These data could indicate that the viral infection was not able to impede cellular proliferation. In addition, newly formed cells manifested some I/G positivity, thus suggesting a common progenitor for both exocrine and endocrine pancreas in the turkey.
Several hypotheses for islet cells regeneration in humans and mice have been postulated and found to be potentially coexisting, such as neogenesis from precursor/acinar cells and proliferation of pre-existing endocrine cells (Holditch et al., Citation2014; Migliorini et al., Citation2014). Ductal progenitor cells in humans were reported to be able to differentiate into all islet lineages, contributing to compensatory growth after tissue destruction (Inada et al., Citation2008; Bhartiya et al., Citation2014; Migliorini et al., Citation2014). Considerable challenges still remain before in vitro production of functional islet (beta) cells can be achieved as a fascinating perspective to replace lost and non-functional cells in both types of diabetes in humans. Both intra-islet (alpha/beta) and extra-islet (acinar/ductal) progenitors might be involved and deserve further analysis.
In conclusion, our data confirm that in turkeys LPAIVs have a tropism for the pancreatic tissue, causing organic and functional damage that can potentially compromise health and zootechnical performances. The transient state of the metabolic change in avians most probably relies on the highly significant compensatory capacity of the pancreas, which would explain the recovery of naturally affected birds in the field. The pathogenic mechanism behind this recovery would be worthy of further investigation as an interesting substrate for comparative medicine.
Additional information
Funding
References
- Abolnik, C., Londt, B.Z., Manvell, R.J., Shell, W., Banks, J., Gerdes, G.H., Akol, G. & Brown, I.H. (2009). Characterisation of a highly pathogenic influenza A virus of subtype H5N2 isolated from ostriches in South Africa in 2004. Influenza and Other Respiratory Viruses, 3, 63–68. doi: 10.1111/j.1750-2659.2009.00074.x
- Alexander, D.J. & Brown, I.H. (2009). History of highly pathogenic avian influenza. Revue Scientifique et Technique, 28, 19–38.
- Bertran, K., Perez-Ramirez, E., Busquets, N., Dolz, R., Ramis, A., Darji, A., Abad, F.X., Valle, R., Chaves, A., Vergara-Alert, J., Barral, M., Höfle, U. & Majó, N. (2011). Pathogenesis and transmissibility of highly (H7N1) and low (H7N9) pathogenic avian influenza virus infection in red-legged partridge (Alectoris rufa). Veterinary Research, 42, 24. doi: 10.1186/1297-9716-42-24
- Bhartiya, D., Mundekar, A., Mahale, V. & Patel, H. (2014). Very small embryonic-like stem cells are involved in regeneration of mouse pancreas post-pancreatectomy. Stem Cell Research & Therapy, 5, 106. doi: 10.1186/scrt494
- Blum, A., Podvitzky, O., Shalabi, R. & Simsolo, C. (2010). Acute pancreatitis may be caused by H1N1 influenza A virus infection. Israel Medical Association Journal, 12, 640–641.
- Calore, E.E., Uip, D.E. & Perez, N.M. (2011). Pathology of the swine-origin influenza A (H1N1) flu. Pathology – Research and Practice, 207, 86–90. doi: 10.1016/j.prp.2010.11.003
- Cano, M., Iglesias, P., Perez, G. & Diez, J.J. (2010). Infección por virus influenza A (H1N1) como causa de cetoacidosis diabética grave en la diabetes tipo 1. Endocrinología y Nutrición, 57, 37–38. doi: 10.1016/S1575-0922(10)70008-5
- Capua, I., Mercalli, A., Pizzuto, M.S., Romero-Tejeda, A., Kasloff, S., De Battisti, C., Bonfante, F., Patrono, L.V., Vicenzi, E., Zappulli, V., Lampasona, V., Stefani, A., Doglioni, C., Terregino, C., Cattoli, G. & Piemonti, L. (2013). Influenza A viruses grow in human pancreatic cells and cause pancreatitis and diabetes in an animal model. Journal of Virology, 87, 597–610. doi: 10.1128/JVI.00714-12
- Capua, I. & Mutinelli, F. (2001a). Mortality in Muscovy ducks (Cairina moschata) and domestic geese (Anser anser var. domestica) associated with natural infection with a highly pathogenic avian influenza virus of H7N1 subtype. Avian Pathology, 30, 179–183. doi: 10.1080/03079450120044597
- Capua, I. & Mutinelli, F. (2001b). A Color Atlas and Text on Avian Influenza. Casalecchio di Reno: Papi Editore.
- Condobery, P.K. & Slemons, R.D. (1992). Biological properties of waterfowl-origin type A influenza viruses in chickens. Avian Diseases, 36, 17–23. doi: 10.2307/1591708
- Dastgheib, S., Irajie, C. Assaei, R., Koohpeima, F. & Mokarram, P. (2014). Optimization of RNA extraction from rat pancreatic tissue. Iranian Journal of Medical Sciences, 39, 282–288.
- De Cock, H.E., Forman, M.A., Farver, T.B. & Marks, S.L. (2007). Prevalence and histopathologic characteristics of pancreatitis in cats. Veterinary Pathology, 44, 39–49. doi: 10.1354/vp.44-1-39
- Gill, S.S., Aubin, R.A., Bura, C.A., Curran, I.H. & Matula, T.I. (1996). Ensuring recovery of intact RNA from rat pancreas. Molecular Biotechnology, 6, 359–362. doi: 10.1007/BF02761714
- Hazelwood, R.L. (1984). Pancreatic hormones, insulin/glucagon molar ratios, and somatostatin as determinants of avian carbohydrate metabolism. Journal of Experimental Zoology, 232, 647–652. doi: 10.1002/jez.1402320333
- Hazelwood, R.L. (2000). Pancreas. In C.G. Whittow. Sturkie's Avian Physiology 5th edn (pp. 539–555). San Diego: Elsevier.
- Hoffmann, W.E. & Solter, P.F. (2008). Diagnostic enzymology of domestic animals. In J. Kaneko, J.W. Harvey & M.L. Bruss. Clinical Biochemistry of Domestic Animals (pp. 365–366). New York: Elsevier Inc.
- Holditch, S.J., Terzic, A. & Ikeda, Y. (2014). Concise review: pluripotent stem cell-based regenerative applications for failing-cell function. Stem Cells Translational Medicine, 3, 653–661. doi: 10.5966/sctm.2013-0184
- Inada, A., Nienaber, C., Katsuta, H., Fujitani, Y., Levine, J., Morita, R., Sharma, A. & Bonner-Weir, S. (2008). Carbonic anhydrase II-positive pancreatic cells are progenitors for both endocrine and exocrine pancreas after birth. Proceedings of the National Academy of Sciences of the United States of America, 105, 19915–19919.
- Johnston, K.M. & Key, D.W. (1992). Paramyxovirus-1 in feral pigeons (Columba livia) in Ontario. The Canadian Veterinary Journal, 33, 796–800.
- Laurent, F. & Mialhe, P. (1978). Effect of free fatty acids and amino acids on glucagon and insulin secretions in normal and diabetic ducks. Diabetologia, 15, 313–321. doi: 10.1007/BF02573825
- Migliorini, A., Bader, E. & Lickert, H. (2014). Islet cell plasticity and regeneration. Molecular Metabolism, 3, 268–274. doi: 10.1016/j.molmet.2014.01.010
- Molesti, E., Cattoli, G., Ferrara, F., Böttcher-Friebertshäuser, E., Terregino, C. & Temperton, N. (2012). The production and development of H7 Influenza virus pseudotypes for the study of humoral responses against avian viruses. Journal of Molecular and Genetic Medicine, 7, 315–320.
- Morales, A.C. Jr, Hilt, D.A., Williams, S.M., Pantin-Jackwood, M.J., Suarez, D.L., Spackman, E., Stallknecht, D.E. & Jackwood, M.W. (2009). Biologic characterization of H4, H6, and H9 type low pathogenicity avian influenza viruses from wild birds in chickens and turkeys. Avian Diseases, 53, 552–562. doi: 10.1637/8877-041509-Reg.1
- Mutinelli, F., Capua, I., Terregino, C. & Cattoli, G. (2003). Clinical, gross, and microscopic findings in different avian species naturally infected during the H7N1 low- and high-pathogenicity avian influenza epidemics in Italy during 1999 and 2000. Avian Diseases, 47, 844–848. doi: 10.1637/0005-2086-47.s3.844
- Nakamura, K., Ohtsu, N., Nakamura, T., Yamamoto, Y., Yamada, M., Mase, M. & Imai, K. (2008). Pathologic and immunohistochemical studies of Newcastle disease (ND) in broiler chickens vaccinated with ND: severe nonpurulent encephalitis and necrotizing pancreatitis. Veterinary Pathology, 45, 928–933. doi: 10.1354/vp.45-6-928
- Nenna, R., Papoff, P., Moretti, C., Pierangeli, A., Sabatino, G., Costantino, F., Soscia, F., Cangiano, G., Ferro, V., Mennini, M., Salvadei, S., Scagnolari, C., Antonelli, G. & Midulla, F. (2011). Detection of respiratory viruses in the 2009 winter season in Rome: 2009 influenza A (H1N1) complications in children and concomitant type 1 diabetes onset. International Journal of Immunopathology and Pharmacology, 24, 651–659.
- OIE. (2014). Avian influenza. In OIE Manual of Diagnostic Tests and Vaccines for Terrestrial Animals. Paris: Office International des Epizooties.
- Okamatsu, M., Saito, T., Yamamoto, Y., Mase, M., Tsuduku, S., Nakamura, K., Tsukamoto, K. & Yamaguchi, S. (2006). Low pathogenicity H5N2 avian influenza outbreak in Japan during the 2005–2006. Veterinary Microbiology, 124, 35–46. doi: 10.1016/j.vetmic.2007.04.025
- Oughton, M., Dascal, A., Laporta, D., Charest, H., Afilalo, M. & Miller, M. (2011). Evidence of viremia in 2 cases of severe pandemic influenza A H1N1/09. Diagnostic Microbiology and Infectious Disease, 70, 213–217. doi: 10.1016/j.diagmicrobio.2010.12.013
- Palmieri, C., Franca, M., Uzal, F., Anderson, M., Barr, B., Woods, L., Moore, J., Woolcock, P. & Shivaprasad, H.L. (2011). Pathology and immunohistochemical findings of West Nile virus infection in psittaciformes. Veterinary Pathology, 48, 975–984. doi: 10.1177/0300985810391112
- Phalen, D.N., Falcon, M. & Tomaszewski, E.K. (2007). Endocrine pancreatic insufficiency secondary to chronic herpesvirus pancreatitis in a cockatiel (Nymphicus hollandicus). Journal of Avian Medicine and Surgery, 21, 140–145. doi: 10.1647/1082-6742(2007)21[140:EPISTC]2.0.CO;2
- Pilny, A.A. (2008). The avian pancreas in health and disease. Veterinary Clinics of North America: Exotic Animal Practice, 11, 25–34.
- Pipi, E., Marketou, M. & Tsirogianni, A. (2014). Distinct clinical and laboratory characteristics of latent autoimmune diabetes in adults in relation to type 1 and type 2 diabetes mellitus. World Journal of Diabetes, 5, 505–510. doi: 10.4239/wjd.v5.i4.505
- Poovorawan, Y., Pyungporn, S., Prachayangprecha, S. & Makkoch, J. (2013). Global alert to avian influenza virus infection: from H5N1 to H7N9. Pathogens and Global Health, 107, 217–223. doi: 10.1179/2047773213Y.0000000103
- Qian, D.H., Zhu, G.J., Wu, L.Z. & Hua, G.X. (2006). Isolation and characterization of a coronavirus from pigeons with pancreatitis. American Journal of Veterinary Research, 67, 1575–1579. doi: 10.2460/ajvr.67.9.1575
- Ritchie, M. & Pilny, A.A. (2008). The anatomy and physiology of the avian endocrine system. Veterinary Clinics of North America: Exotic Animal Practice, 11, 1–14.
- Shinya, K., Awakura, T., Shimada, A., Silvano, F.D., Umemura, T. & Otsuki, K. (1995). Pathogenesis of pancreatic atrophy by avian influenza A virus infection. Avian Pathology, 24, 623–632. doi: 10.1080/03079459508419102
- Spackman, E., Senne, D.A., Myers, T.J., Bulaga, L.L., Garber, L.P., Perdue, M.L., Lohman, K., Daum, L.T. & Suarez, D.L. (2002). Development of a real-time reverse transcriptase PCR assay for type A influenza virus and the avian H5 and H7 hemagglutinin subtypes. Journal of Clinical Microbiology, 40, 3256–3260. doi: 10.1128/JCM.40.9.3256-3260.2002
- Tanimura, N., Tsukamoto, K., Okamatsu, M., Mase, M., Imada, T., Nakamura, K., Kubo, M., Yamaguchi, S., Irishio, W., Hayashi, M., Nakai, T., Yamauchi, A., Nishimura, M. & Imai, K. (2006). Pathology of fatal highly pathogenic H5N1 avian influenza virus infection in large-billed crows (Corvus macrorhynchos) during the 2004 outbreak in Japan. Veterinary Pathology, 43, 500–509. doi: 10.1354/vp.43-4-500
- Teifke, J.P., Klopfleisch, R., Globig, A., Starick, E., Hoffmann, B., Wolf, P.U., Beer, M., Mettenleiter, T.C. & Harder, T.C. (2007). Pathology of natural infections by H5N1 highly pathogenic avian influenza virus in mute (Cygnus olor) and whooper (Cygnus cygnus) swans. Veterinary Pathology, 44, 137–143. doi: 10.1354/vp.44-2-137
- Tse, H., To, K.K., Wen, X., Chen, H., Chan, K.H., Tsoi, H.W., Li, I.W. & Yuen, K.Y. (2011). Clinical and virological factors associated with viremia in pandemic influenza A/H1N1/2009 virus infection. PLoS One, 6, e22534. doi: 10.1371/journal.pone.0022534
- Watanabe, N. (2011). Conversion to type 1 diabetes after H1N1 influenza infection: a case report. Journal of Diabetes, 3, 103. doi: 10.1111/j.1753-0407.2010.00110.x
- Zanella, A. (2003). Avian influenza attributable to serovar H7N1 in light layers in Italy. Avian Diseases, 47, 1177–1180. doi: 10.1637/0005-2086-47.s3.1177