ABSTRACT
Reassortment among genome segments of infectious bursal disease virus (IBDV) field isolates was reported frequently worldwide, however the pathogenicity of the reassortant field IBDV is poorly understood. In this paper, a pathogenicity study on four representative IBDV field strains isolated from Southern China between 2005 and 2011 was conducted. Twenty-eight-day-old Three-Yellow chickens were divided into four groups and were inoculated intraocularly with one of the four field IBDV strains, namely NN1172, NN1005, GD10111 and JS7, respectively. The mortality and relative weight of bursa and thymus were subsequently determined in the acute phase of infection. In addition, B cells, T cells (CD4+ and CD8+) and virus were quantified in the bursa of Fabricius and thymus, respectively, by flow cytometry and real-time reverse transcription-polymerase chain reaction. The results showed that isolate NN1172, of which parts of segment A and B encoding the hypervariable (v) region of viral protein (VP2) and VP1, respectively, derived from vvIBDV strains, showed the most severe pathogenicity, and caused the most severe bursal B cell depletion as well as CD4+ and CD8+ T cell infiltration in the bursa of Fabricius. However, the virus induced the strongest decrease in CD4+ and CD8+ T cells in the thymus and exhibited the most efficient viral replication in the target organs. Isolate NN1005, whose vVP2 derived from vvIBDV and VP1 from unidentified origin, exhibited relatively lower pathogenicity compared to NN1172. The other two isolates, JS7 and GD10111, of which the vVP2 derived from vvIBDV and intermediate IBDV, and VP1 from 002–73 and attenuated IBDV, respectively, showed the lowest level of virulence. Our results suggest that various IBDV field isolates with different natural segment reassortments exhibit differential pathogenicity after infection of commercial Three-Yellow chickens.
Introduction
Infectious bursal disease (IBD) is a fatal or immunosuppressive disease of young chickens (Eterradossi & Saif, Citation2013). The disease is caused by infectious bursal disease virus (IBDV), an Avibirnavirus, a member of the family Birnaviridae, and characterized by destruction of lymphoid organs in 3–6-week-old chickens. There are two serotypes of IBDV (serotype 1 and serotype 2) and only serotype 1 virus is pathogenic for chickens. To date, there are five pathotypes of IBDV with increasing order of virulence as mild (attenuated), intermediate, classical and very virulent (vv) as well as variant virulent strains (Van Den Berg, Citation2000). vvIBDV can break through the protection of high titres of maternally derived antibodies and causes high rates of mortality in young birds (Wyeth & Chettle, Citation1990).
The genome of IBDV consists of two segments (A and B) of linear double-stranded RNA. Segment A (3261 bp) encodes a precursor protein, which is further cleaved by auto-proteolysis to produce mature VP2 (outer capsid), VP3 (inner capsid), and VP4 (a serine protease) (Azad et al., Citation1985; Birghan et al., Citation2000). VP2 is the major host-protective immunogen of IBDV and carries all the neutralizing epitopes (Fahey et al., Citation1989), of which some are responsible for antigenic variation (Schnitzler et al., Citation1993), and some for viral virulence (Brandt et al., Citation2001; Mahgoub et al., Citation2012). Segment B (2827 bp) encodes a 91-kD RNA-dependent RNA polymerase, VP1. VP1 is responsible for replication of the viral genome and the synthesis of mRNA. Increasing evidence revealed that VP1 also modulates IBDV virulence in vivo (Liu & Vakharia, Citation2004; Boot et al., Citation2005; Le Nouën et al., Citation2012).
Ig-M bearing B cells in the bursa of Fabricius (BF) are the principal targets of IBDV. Rapid progressive loss of B cells occurs following IBDV infection in the bursal cortex and medulla, peripheral blood and thymic medulla (Mahgoub et al., Citation2012). In fact, all compartments of the bird's immune system will be affected during infection with IBDV. T cells, which are not infected by IBDV, may modulate the pathogenesis by limiting viral replication in the BF during early phase of the disease by promoting bursal tissue damage and delaying tissue recovery (Aricibasi et al., Citation2010). Both CD4+ and CD8+ T cells infiltrate the IBDV-infected BF after infection (Kim et al., Citation2000). IBDV strains differing in virulence may cause differential disruption of the T-cell system (Williams & Davison, Citation2005).
Many researchers have documented that the difference in the characteristics of the IBDV genome may contribute to differences in virulence. Gene mutation(s) in the hypervariable region of VP2 (vVP2) and segment reassortment events of the IBDV genome were reported by our laboratory and other researchers (Islam et al., Citation2001; He et al., Citation2014); in addition, homologous recombination within segments has been reported previously (He et al., Citation2009; Jackwood, Citation2012). These mutation(s), heterologous reassortments or homologous recombinations were shown to influence tissue adaptation (Van Loon et al., Citation2002), virulence (Hoque et al., Citation2001; Banda & Villegas, Citation2004), pathogenicity (Hon et al., Citation2006; Le Nouën et al., Citation2006), and antigenicity (Jackwood, Citation2012) of IBDV isolates. Given the IBDV genome is composed of RNA, together with the complexity of IBDV immunization procedures, incomplete biosecurity measures in the poultry industry, and co-infection with different field strains, the molecular evolution of IBDV is still ongoing and becoming even more severe. Segment reassortant IBDV strains were shown to be prevalent in the field (Le Nouën et al., Citation2006; Wei et al., Citation2006, Citation2008; Chen et al., Citation2012; Kasanga et al., Citation2013; He et al., Citation2014; Lu et al., Citation2015). The pathogenicity of some of these reassortant field strains was investigated in specific pathogen free (SPF) layer chickens, which revealed that it correlated with IBDV genome composition (Wei et al., Citation2006, Citation2008; Lu et al., Citation2015). However, the pathogenicity of these viruses in commercial chickens has remained largely unknown. Our laboratory has reported previously that IBDV field isolates in southern China could be divided into different genotypes based on partial sequencing of the virus genome, and exchanged/reassortment isolates were the main strains circulating in the field in southern China between 2000 and 2012 (He et al., Citation2014). Furthermore, the IBDV isolates exhibited different kind of natural reassortments. We have noticed that most of those reassortant isolates came from flocks vaccinated with classical IBDV vaccines (He et al., Citation2012). To the best of our knowledge, to date there is no report describing the pathogenicity of these different kinds of reassortant IBDV field strains. In this study we investigated the differential pathogenicity of the different kinds of natural reassortant IBDV field isolates. So far, most of the basic studies on IBDV pathogenesis have been done in SPF layer chickens. Aricibasi et al. (Citation2010) documented that the genetic background of the chicken significantly influences the disease outcome. The Three-Yellow chicken is one of the most popular meat-type chickens in southern China. In order to determine the pathogenicity of natural reassortant IBDV strains in a field-associated genotype, a pathogenicity study was carried out using the commercial Three-Yellow chickens. Our investigation provides important new insights into the pathogenicity of naturally occurring reassortant IBDV field isolates.
Materials and methods
Viruses
The four IBDV field strains used in the study were JS7 (isolated from Jiangsu in 2005), GD10111 (isolated from Guangdong in 2010), NN1005 (isolated from Nanning in 2010) and NN1172 (isolated from Nanning in 2011). According to the phylogenetic analysis based on partial sequences of the IBDV genome, the vVP2 of JS7 derived from vvIBDV G2 strain and VP1 from 002–73 (a classical virulent IBDV); the vVP2 of GD10111 derived from IBDV of intermediate virulence and VP1b from attenuated IBDV; the vVP2 of NN1005 derived from vvIBDV G1 strain and VP1 from unidentified origin; the vVP2 of NN1172 derived from vvIBDV G3 and VP1 from HLJ0504-like strain (a vvIBDV strain with an unique VP1 sequence). All the strains were plaque purified. The information of these strains is given in . The phylogenetic analysis of these isolates was previously described (He et al., Citation2014).
Table 1. IBDV field isolates used in this study.
Chickens
Day-old commercial Three-Yellow chickens were purchased and transferred to the isolation facility and no vaccination was administered. All the chickens were tested to be free of maternally derived IBDV antibodies by using a commercial enzyme-linked immunosorbent assay kit, FlockChek Infectious Bursal Disease Antibody Test Kits (IDEXX Laboratory, Inc., Westbrook, ME, USA) before virus inoculation. Chickens were reared in isolation units for the duration of the study and were housed in separate isolation units after infection. The bird experiments were conducted in accordance to the International Guiding Principles for Biomedical Research Involving Animals as issued by the Council for the International Organizations of Medical Sciences.
Virus preparation and titration
All purified isolates were propagated in Vero cells for 1–2 passage. Cells were then harvested, frozen (−70°c) and thawed three times, the infected cells were centrifuged at 3000 × g for 10 min. The clear supernatants containing IBDV were aliquoted and stored at −70°c. At the same time, virus titration was performed on the cell cultured-derived IBDV by incubation Vero cells with 10-fold serial dilutions (10−1−10−10). Virus dilutions were prepared in DMEM with antibiotics, and 0.1 ml was used to infect the Vero cells. Eight replicate wells of a 96-well cell culture plate were used for each dilution. The inoculated cells were incubated for four days and investigated daily by microscope for cytopathic effects. The number of wells with cytopathic effects of each dilution was recorded and the virus titres were calculated by the method of Reed & Muench. Titres are expressed as tissue culture infectious dose (TCID)50/ml (Reed & Muench, Citation1938).
Pathogenesis study
One hundred and fifty 4-week-old commercial Three-Yellow chickens were randomly divided into five groups with 30 chickens per group. Birds in Groups 1–4 were inoculated per eye-drop with NN1172, NN1005, GD10111 and JS7 at a dosage of 105 TCID50, while birds in Group 5 were inoculated with phosphate-buffered saline and served as uninfected controls. Ten inoculated birds per group were bled and sacrificed at 3 and 7 days post-infection (dpi). Bursa and thymus samples were collected from each bird, and the mean organ/body weight (BW) ratio was determined according to Ismail and Saif (Citation1991) using the following formula: (organ weight in grams × 1000)/BW in grams. From the 10 birds from each group at each time point, samples from five birds were subjected to lymphoid cell isolation and flow cytometric analysis, and the lymphoid tissue samples from the remaining five birds were subjected to RNA isolation and virus detection by reverse transcription-polymerase chain reaction.
Lymphoid cell isolation and flow cytometry analysis
Bursa and thymus samples from infected and uninfected birds were removed during necropsy, excised and washed in ice-cold DMEM. Single-cell suspensions were prepared by homogenizing, and filtering through a 70 µm nylon mesh. Cell pellets were suspended in 5 ml of complete DMEM medium and enumerated with a haemocytometer after dilution in trypan blue (Sigma, St. Louis, MO, USA). 1 × 106 cells were stained with anti-CD4-PE, CD8-RPE, and Bu-1-FITC (a specific B cell surface marker) antibodies. All staining reactions were performed according to the manufacturers’ protocols. Data were collected on a FACSAria COULTER flow cytometer using FACSEXPO32 software (BECKMAN, San Jose, CA, USA) and analysed using EXPO32 ADC analysis software (Tree Star, San Carlos, CA, USA). All antibodies were purchased from Southern Biotechnology Associates (Birmingham, AL, USA).
Extraction of viral RNA and quantitative RT-PCR
Bursa and thymus samples from infected and uninfected birds were subjected to RNA extraction by using TRIzol (Invitrogen Corp., Carlsbad, CA, USA). Briefly, bursa/thymus samples were homogenized in sterile physiological saline solution (1:5, w/v), the mixture was then frozen and thawed three times and finally centrifuged at 3000 × g for 10 min. Two hundred micro litres of the supernatants were suspended in 1 ml of TRIzol reagent and total RNA was prepared according to the manufacturer's instructions. Finally, total RNA extracted from each sample was suspended in 30 μl of water and used for virus detection by qRT-PCR as described in a previous study (He et al., Citation2013).
Statistical analysis
All values are reported as means ± standard errors (SE). Statistical significance was calculated using one-way analysis of variance whenever multiple groups were compared. For individual comparisons of multiple groups, Student–Newman–Keuls post-hoc test was used to calculate P values. Means with P values of <0.005 were considered significantly different. All statistical calculations were performed using Primer of Biostatistics (Glantz, Citation2012).
Results
IBDV field isolates differentially caused clinical disease
First, we sought to determine the overall pathogenicity of the IBDV field isolates using Three-Yellow chicken, a popular meat-type of chicken in southern China. To do this, we infected 4-week-old Three-Yellow chickens with four IBDV field isolates as described in Materials and Methods. Chickens exhibited distinct clinical signs (e.g. ruffled feathers, hunched posture, lethargy) and gross lesions after infection (data not shown). All four isolates resulted in mortalities during the 1–7 dpi observation period as given in : NN1172 infection led to the highest mortality rate (30%), followed by NN1005 (mortality rate 20%), GD10111 and JS7 (equivalent mortality rate, 10%). Chickens in the NN1172-infected group died as early as 3 dpi, however, dead chickens were observed mostly at the time between 4 and 6 dpi. Dead birds had typical IBD lesions (haemorrhagic and enlarged or atrophied lymphoid organs, muscular haemorrhages and swollen kidneys). As expected, no clinical signs or deaths were observed in the uninfected control chickens.
Table 2. Pathogenicity of IBDV field strains in Three-Yellow chickens.
The relative bursa and thymus weights of birds of each group at 3 and 7 dpi are presented in . The bursa/body weight (B/BW) and thymus/body weight (T/BW) ratios of the infected chickens increased (P < 0.05) at 3 dpi, while both decreased (P < 0.05) at 7 dpi. The B/BW and T/BW ratios at 3 dpi were not significantly different among the four IBDV-infected groups, however, chickens in the NN1172-infected group showed the smallest bursa and thymus (P < 0.05) at 7 dpi, compared to birds of the other three-infected groups and mock-infected ones. The results suggest that IBDV field isolates with various genome segment reassortments caused different bursa and thymus atrophy pattern.
IBDV field isolates differentially induce depletion of B lymphocytes in the bursa and thymus
In order to determine if the differences in clinical disease caused by the reassortant IBDV field isolates differentially affected the target B cells, Bu-1+ cells in bursa and thymus were analysed at 3 and 7 dpi by flow cytometric analysis. As shown in , Bu-1+ cells in the bursa increased transiently at 3 dpi, and rapidly depleted at 7 dpi. Among the four isolates of different genotype, NN1172 induced the most severe depletion of Bu-1+ cells, followed by NN1005 and GD10111, while the JS7-infected group showed the least depletion of Bu-1+ cells in the BF. All the IBDV isolates induced depletion of Bu-1+ cells in the thymus of infected birds at both investigated time points (3 and 7 dpi) compared to uninfected chickens. Interestingly, the pattern among the four isolates was different in the thymus. NN1172 and NN1005 induced constant depletion at 3 and 7 dpi, while the Bu-1+ cell numbers in DG10111- and JS7-infected groups were beginning to increase again at 7 dpi compared to 3 dpi, but numbers were still lower compared to those of the uninfected control. Flow cytometry data suggested that IBDV isolates with different genome segment composition may differentially modulate Bu-1+ cells in bursa and thymus after infection of commercial Three-Yellow chickens. The isolate NN1172, whose VP1 is similar to HLJ-0504 (vvIBDV with unique VP1 sequence) and VP2 belonging to vvIBDV of sub-group G3 (He et al., Citation2014), induced the most severe depletion of Bu-1+ cells at 7 dpi in the bursa and constant depletion in the thymus.
Figure 1. Percentage of B cells per total number of lymphoid cells in the bursa and thymus. (a) Bursal sample; (b) thymus sample. Flow cytometry detection revealed differential modulation of Bu-1+ B cell numbers by different IBDV field strains. Presented is the fold change in the infected group relative to the corresponding control group of this experiment. Error bars indicate the means ± SE per group. *P < 0.05 significantly different between the NN1172-infected group and the other three IBDV-infected groups. #P < 0.05 significant difference between the NN1005- and GD10111-infected group, ^P < 0.05 significant difference between NN1005-infected and JS7-infected group. $P < 0.05 significant difference between GD10111- and JS7-infected group. n = 5/group.
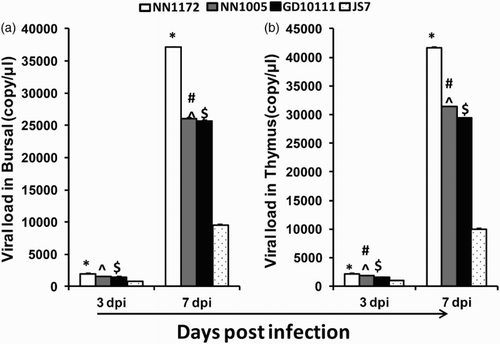
IBDV field isolates differentially modulated CD4+ and CD8+ cells in the bursa and thymus
CD4+ and CD8+ T cells were analysed to elucidate the lymphoid cells involved in bursa and thymus pathology, viral clearance, and/or immunosuppression. CD4+ and CD8+ T cells were detected by flow cytometric analysis at 3 and 7 dpi, respectively. The results are given in . At 3 dpi, the proportion of the CD4+ and CD8+ T cells was transiently decreased in the bursa of infected groups. However, both CD4+ and CD8+ T cell numbers rapidly increased again in infected groups at 7 dpi. Among the four different IBDV-genotypes, NN1172, NN1005 and GD10111 induced a significant increase in CD4+ and CD8+ T cell numbers in the bursa compared to uninfected birds, of which NN1172-infected birds exhibited the strongest increase of both CD4+ and CD8+ T cells. At 7 dpi, isolate JS7 induced a still further decrease in CD4+ T cells but an increase in the number of CD8+ T cells compared to uninfected birds, as well as compared to CD8+ T cell numbers of this group measured at 3 dpi. These data suggest that the strongest infiltration of both CD4+ and CD8+ T cells was induced by NN1172 in the BF and would suggest the strongest viral clearance response and slower bursa recovery than those induced by other isolates of lesser virulence.
Table 3. Percentage of CD4+ and CD8+ T cells per total number of lymphoid cells.
The trend of CD4+ and CD8+ T cell number in the thymus was similar to that of Bu-1+ in the bursa. The four isolates induced transiently increasing numbers of CD4+ and CD8+ T cells at 3 dpi, and then induced significant decreasing numbers at 7 dpi (). The decrease of the CD8+ cell was greater than that of CD4+ T cells. NN1172 and NN1005 induced the strongest decrease in CD8+ cells while JS7 induced the least decrease of CD8+ cells in the thymus. These data were consistent with those of Mahgoub's group (Mahgoub et al., Citation2012), who noted that depletion of cortical thymocytes was evident during the acute phase of the infection. The results suggested that the T cells in the thymus were affected by the IBDV field isolates and the depletion of the T cells in the thymus was differentially affected by isolates of different genotypes, with NN1172 as the most virulent isolate, inducing the most severe T cell depletion.
Table 4. Percentage of CD4+ and CD8+ T cells per total number of lymphoid cells in the thymus.
IBDV field isolates replicated differently in target organs
The replication rate of the IBDV isolates in bursa and thymus was detected by qRT-PCR assay at 3 and 7 dpi. As shown in , all the four isolates replicated effectively in bursa and thymus at both investigated time points, however, the quantity of viral RNA was low at 3 dpi, but significantly increased at 7 dpi. NN1172 showed the most effective replication as demonstrated by the highest amount of viral RNA in the bursa and thymus. The replication ability of JS7 was the least effective. These data suggested that IBDV field isolates differing in partial genome segments (vVP2 and VP1) exhibited differential ability of replication in target organs. The virus with the highest virulence showed the strongest capability of replication, coinciding with depletion of B cells and T cells in target organs.
Figure 2. Viral load by quantitative RT-PCR. (a) Bursa samples; (b) thymus samples. Data are expressed mean copy number ± SE (five chickens and above per group at each time point). *P < 0.05 significant difference between NN1172-infected and other groups. #P < 0.05 significant difference between NN1005- and GD10111-infected group, ^P < 0.05 significant difference between NN1005- and JS7-infected group. $P < 0.05 significant difference between GD10111- and JS7-infected group.
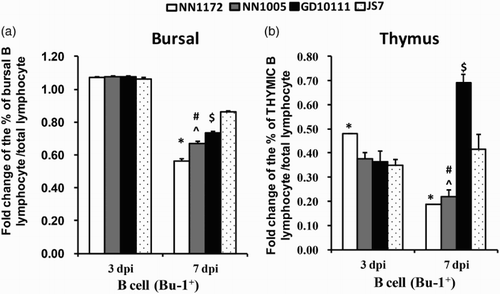
Discussion
The present study demonstrated different pathogenicity of IBDV field isolates with different natural genome segment reassortments after challenge of commercial Three-Yellow chickens. A number of reports showed that mutations in both genome segments, or reassortment between two segments of IBDV, affected the pathogenicity of the virus (Lim et al., Citation1999; Mundt, Citation1999; Van Den Berg, Citation2000; Qi et al., Citation2009). Further, Boot and co-workers reported that laboratory-engineered reassortant viruses deriving from vvIBDV exhibited delayed replication in the bursa (Boot et al., Citation2005) or failed to induce morbidity and mortality (Boot et al., Citation2000) unless they also had a typical vvIBDV-related segment B. Recently, Jackwood et al. (Citation2011) and Le Nouën et al. (Citation2006) reported that naturally occurring segment B-reassorted vvIBDV isolates led to reduced pathogenicity in chickens. Both A and B genome segments were proved by reverse genetics to be essential for the pathogenesis of IBDV (Escaffre et al., Citation2013). The vVP2 in segment A and the 5′ terminal of VP1 in segment B (VP1-b) have been demonstrated to be sufficient to assess the genetic relatedness of IBDV isolates. Based on these partial sequence analyses, the diversity of segment A and segment B was documented and segment reassorted IBDVs were identified (Le Nouën et al., Citation2005, Citation2006; Jackwood, Citation2012). In our previous study (He et al., Citation2014), IBDV field isolates NN1172, NN1005, GD10111 and JS7, all identified to be natural segment reassortant strains based on these two regions, exhibited different derivatives of partial sequences in vVP2 and VP1-b segments. The genome of NN1172 consists of vvIBDV-vVP2 and HLJ0504-VP1-b. The VP1 of HLJ0504 is derived from a distinct ancestor which is different from both vvIBDV and attenuated IBDV VP1 sequences, but HLJ0504 is very virulent in SPF chickens, in this regard, the VP1-b of NN1172 can be considered to be derived from vvIBDV. In this study, the mortality caused by NN1172 in the Three-Yellow chickens is about 30%, lower than its mortality in SPF chickens (100%, 4/4, data not shown), which was consistent with the result of HLJ0504 (vvIBDV) (Qi et al., Citation2011). It was documented that the mortality caused by vvIBDV field isolates could reach up to 100% in SPF chickens, 50–60% in layers, and 25–30% in broilers under experimental conditions (Ingrao et al., Citation2013). In this regard, NN1172 is a highly virulent strain, consistent with its genome characteristics. The vVP2 of NN1005 came from vvIBDV, but the origin of VP1-b could not be identified (either different from vvIBDV or from attenuated IBDV, or related to HLJ0504). We found that the mortality caused by NN1005 is about 20% in the commercial chickens, which is lower than that of NN1172 (). Isolate GD10111, whose vVP2 came from an intermediate IBDV and VP1-b from attenuated IBDV, induced 10% mortality in the chickens. The isolate JS7, whose vVP2 came from vvIBDV and VP1-b from classical strain 002–73, also showed 10% mortality. These results further suggest that the pathogenicity of IBDV field strains for commercial chickens is correlated with the origins of both genome segments. Isolates whose genome segments derived from vvIBDV strains are highly related to severe pathogenicity.
IBDV is known to cause lytic infection of the resident B cells within the acute phase of the infection, which may last up to 7–10 days, bursal follicles are depleted of B cells and the BF becomes atrophic (Sharma et al., Citation2000). In a study by Williams and Davison (Citation2005), the depletion of Bu-1+ cells in BF and thymus of chickens infected with vvIBDV strain UK661 was evident. UK661 caused earlier and more severe immunopathology than classical virulent strains. In our study, all the four field strains induced depletion of Bu-1+ cells with NN1172 inducing the most severe depletion of B cells at 7 dpi both in the BF and thymus of infected birds. The differentially induced depletions of B cells were consistent with the clinical mortalities observed in infected birds suggesting that NN1172 exhibited the highest pathogenicity for the chicken bursa among the four field isolates. However, we found that Bu-1+ cells increased in the BF at 3 dpi. This result seems inconsistent with the fact that the B cell is lysed by IBDV. Anatomically, blood capillaries are present in the cortex of the bursal follicle, blood capillaries and small venules are also present in inter follicular tissue (Frazier, Citation1974; Tanimura & Sharma, Citation1997). At 3 dpi, the thymus and bursa exhibited severe haemorrhages, and most of the lymphocytes were recruited into the IBDV target organs, with most of the T cells into thymus, and most of the B cells into the bursa, resulting in the transient increasing numbers of B cells in the bursal, and T cells in the thymus. Tanimura and Sharma (Citation1997) found that severe bursal haemorrhage at 3 dpi would affect the distribution of T cells. We speculate that severe bursal haemorrhages would also affect the Bu-1+ cell at 3 dpi, since all the BF of infected birds were severely haemorrhaged at 3 dpi.
There is evidence that T cells also play a key role in the pathogenesis of IBDV. Chemical induction of the T cell depletion caused an increase in the bursal viral load (Kim et al., Citation2000). In our study, the numbers of both the CD4+ and CD8+ T cells increased in the bursal at seven days after infection with all four strains, accompanied by the increasing viral load, which is consistent with the results of Tanimura and Sharma (Citation1997). They suggested that the T cell response was associated with IBDV antigen load in the bursa. Kim et al. (Citation2000) also revealed that the infiltration of CD4+ and CD8+ T cells started at 4 dpi and increased until T cells represented 65% of the bursal cell population at 7 dpi. Williams's group (Williams & Davison, Citation2005) also suggested that CD4+ and CD8+ T cells increased throughout the bursa until at least 14 dpi. The number of T cells (both CD4+ and CD8+) increased most significantly after NN1172 infection in comparison to the other isolates in the bursa, consistent with its increasing viral load, then followed by isolates NN1005, GD10111 and the least response was by JS7.
However, the number of T cells (CD4+ and CD8+) decreased at 3 dpi in the bursa. We noticed that this result is not consistent with most of the reports. However, we also noticed that the time when CD4+ and CD8+ T cells influx is detected in the bursa is not so clearly defined according to the reported data. Kim and co-workers (Kim et al., Citation2000) demonstrated infiltration of CD4+ and CD8+ T cells into the IBDV-infected BF starting at 4 dpi. Poonia and Charan (Citation2004) reported substantial infiltration of CD4+ and CD8+ T-cells into the bursal follicles of virus-infected groups from 4 dpi onwards. In a more recent report by Withers et al. (Citation2005), the numbers of CD4+ and CD8+ cells increased in the BF as early as 1 dpi. We propose that the decreasing number of CD4+ and CD8+ T cells at 3 dpi in the bursa in our study is related to the transient increasing number of Bu-1+ cells in the BF and T cells in the thymus because of severe haemorrhage. Since most of the B cells influx into the bursa and most T cell influx into the thymus, a transient decrease of T cell numbers in the bursa at 3 dpi may be observed. However, this situation needs further investigation.
The T cell populations changed predominantly in the infected thymus. In Williams and Davison's (Citation2005) study, the numbers of CD4+ and CD8+ cells in the infected thymus showed marked variations from day to day, both populations decreased over time. Fewer CD4+ cells were detected at 7 dpi in infected thymus. In our study, CD4+ and CD8+ cells transiently increased at 3 dpi in thymus of all infected birds because of severe haemorrhage, and then decreased significantly at 7 dpi, consistent with the results of Williams and Davison (Citation2005). NN1172 induced a marked decrease of CD4+ and CD8+ cells. Tanimura and Sharma (Citation1997) revealed that the severity of pathology after UK661 infection greatly exceeded the ones of the less virulent strains, and the thymus pathology progressed much further. In this regard, it should be suggested that NN1172 should be more virulent than other strains. The remaining strains are ranked in order of decreasing virulence as NN1005, GD10111 and JS7.
Variation in the chickens’ breed susceptibility has been documented for many poultry diseases (Bumstead et al., Citation1991). In the case of IBDV, brown and white Leghorn breeds are more susceptible than broiler breeds (Bumstead et al., Citation1993). In a study of Hassan et al. (Citation2002) most of the mortalities occurred between 4 and 6 dpi when inoculating native breeds, and the mortalities caused by IBDV were lower than those in brown and white Leghorn breeds. In our study, the earliest mortalities caused by NN1172 occurred at 4 dpi, similar to Hassan et al. (Citation2002) results, and the mortalities were not as high as those in white Leghorn SPF chickens. However, the morbidities were as high as 100% for all of the four field isolates. The results of our pathogenicity experiment with IBDV in commercial chickens may be more indicative of the disease in field cases than experiments in SPF chickens.
In summary, IBDV field isolates with different natural segment reassortments exhibited differential pathogenicity in commercial Three-Yellow chickens. In case of the four isolates in our study, NN1172 showed the most severe pathogenicity as exhibited by highest mortality, most severe bursal B cell depletion, most severe T cell infiltration in the BF and T cell depletion in the thymus, and highest viral load in the target organs. NN1005 ranked the second in severity of pathogenicity, while GD10111 and JS7 would be the least virulent among the four field isolates. The results also suggested that a molecular epidemiology study based on partial IBDV genome sequencing, as our group reported (He et al., Citation2014), is still important to IBDV control, as some changes of the field isolates (not only the changes of the genome, but also the pathogenicity trend) could be inferred from those studies. However, the characteristics of the whole genome, which site or sites in the genome contribute to the differential pathogenicity and what mechanisms are involved in the differential pathogenicity still need to be investigated to further elucidate the molecular pathogenicity of the virus.
Disclosure statement
No potential conflict of interest was reported by the authors.
Additional information
Funding
References
- Aricibasi, M., Jung, A., Heller, E.D. & Rautenschlein, S. (2010). Differences in genetic background influence the induction of innate and acquired immune responses in chickens depending on the virulence of the infecting infectious bursal disease virus (IBDV) strain. Veterinary Immunology and Immunopathology, 135, 79–92. doi: 10.1016/j.vetimm.2009.11.005
- Azad, A.A., Barrett, S.A. & Fahey, K.J. (1985). The characterization and molecular cloning of the double-stranded RNA genome of an Australian strain of infectious bursal disease virus. Virology, 143, 35–44. doi: 10.1016/0042-6822(85)90094-7
- Banda, A. & Villegas, P. (2004). Genetic characterization of very virulent infectious bursal disease viruses from Latin America. Avian Diseases, 48, 540–549. doi: 10.1637/7157-12304R
- Birghan, C., Mundt, E. & Gorbalenya, A.E. (2000). A non-canonical ion proteinase lacking the ATPase domain employs the ser-Lys catalytic dyad to exercise broad control over the life cycle of a double-stranded RNA virus. The EMBO Journal, 19, 114–123. doi: 10.1093/emboj/19.1.114
- Boot, H.J., Hoekman, A.J. & Gielkens, A.L. (2005). The enhanced virulence of very virulent infectious bursal disease virus is partly determined by its B-segment. Archives of Virology, 150, 137–144. doi: 10.1007/s00705-004-0405-9
- Boot, H.J., ter Huurne, A.A., Hoekman, A.J., Peeters, B.P. & Gielkens, A.L. (2000). Rescue of very virulent and mosaic infectious bursal disease virus from cloned cDNA: VP2 is not the sole determinant of the very virulent phenotype. Journal of Virology, 74, 6701–6711. doi: 10.1128/JVI.74.15.6701-6711.2000
- Brandt, M., Yao, K., Liu, M., Heckert, R.A. & Vakharia, V.N. (2001). Molecular determinants of virulence, cell tropism, and pathogenic phenotype of infectious bursal disease virus. Journal of Virology, 75, 11974–11982. doi: 10.1128/JVI.75.24.11974-11982.2001
- Bumstead, N., Millard, B.M., Barrow, P.A. & Cook, J.K.A. (1991). Genetic basis of disease resistance in chickens. In J.B. Owan & R.F.E. Axford. Breeding for Disease Resistance in Farm Animals (pp. 10–23). Wallingford: Commonwealth Agricultural Bureau.
- Bumstead, N., Reece, R.L. & Cook, J.K. (1993). Genetic differences in susceptibility of chicken lines to infection with infectious bursal disease virus. Poultry Science, 72, 403–410. doi: 10.3382/ps.0720403
- Chen, F., Liu, J., Yan, Z., Liu, D., Ji, J., Qin, J., Li, H., Ma, J., Bi, Y. & Xie, Q. (2012). Complete genome sequence analysis of a natural reassortant infectious bursal disease virus in China. Journal of Virology, 86, 11942–11943. doi: 10.1128/JVI.02043-12
- Escaffre, O., Le Nouën, C., Amelot, M., Ambroggio, X., Ogden, K.M., Guionie, O., Toquin, D., Müller, H., Islam M.R., Eterradossi N. (2013). Both genome segments contribute to the pathogenicity of very virulent infectious bursal disease virus. Journal of Virology, 87, 2767–2780. doi: 10.1128/JVI.02360-12
- Eterradossi N. & Saif Y.M. (2013). Infectious bursal disease. In D.E. Swayne, J.R. Glisson, L.R. Mcdougald, L.K. Nolan, D.L. Suarez, & V. Nair. Diseases of Poultry 13th edn (pp. 219–246). Ames: Wiley-Blackwell.
- Fahey, K.J., Erny, K. & Crooks, J. (1989). A conformational immunogen on VP2 of infectious bursal disease virus that induces virus-neutralizing antibodies that passively protect chickens. Journal of General Virology, 70, 1473–1481. doi: 10.1099/0022-1317-70-6-1473
- Frazier, J.A. (1974). The ultrastructure of the lymphoid follicles of the chick bursa of Fabricius. Acta Anatomica, 88, 385–397. doi: 10.1159/000144247
- Glantz, S.A. (2012). Primer of Biostatistics. New York: McGraw-Hill.
- Hassan, M.K., Afify, M. & Aly, M.M. (2002). Susceptibility of vaccinated and unvaccinated Egyptian chickens to very virulent infectious bursal disease virus. Avian Pathology, 31, 149–156. doi: 10.1080/03079450120118630
- He, C.Q., Ma, L.Y., Wang, D., Li, G.R. & Ding, N.Z. (2009). Homologous recombination is apparent in infectious bursal disease virus. Virology, 384, 51–58. doi: 10.1016/j.virol.2008.11.009
- He, X.M., Wei, P., Yang, X., Guan, D., Wang, G. & Qin, A. (2012). Molecular epidemiology of infectious bursal disease viruses isolated from Southern China during the years 2000–2010. Virus Genes, 45, 246–255. doi: 10.1007/s11262-012-0764-3
- He, X.M., Gan, S.S., Xiong, Z.X., Xuan, J.C., Liao, Y.J. & Wei, P. (2013). Development and Application of Real Time Quantitative RT-PCR Assay for IBDV Detection. Southwest China Journal of Agricultural Sciences, 26, 798–802.
- He, X.M., Xiong, Z., Yang, L., Guan, D., Yang, X. & Wei, P. (2014). Molecular epidemiology studies on partial sequences of both genome segments reveal that reassortant infectious bursal disease viruses were dominantly prevalent in southern China during 2000–2012. Archives of Virology, 159, 3279–3292. doi: 10.1007/s00705-014-2195-z
- Hon, C.C., Lam, T.Y., Drummond, A., Rambaut, A., Lee, Y.F., Yip, C.W., Zeng, F, Lam, P.Y., Ng, P.T. & Leung, F.C. (2006). Phylogenetic analysis reveals a correlation between the expansion of very virulent infectious bursal disease virus and reassortment of its genome segment B. Journal of Virology, 80, 8503–8509. doi: 10.1128/JVI.00585-06
- Hoque, M.M., Omar, A.R., Chong, L.K., Hair-Bejo, M. & Aini, I. (2001). Pathogenicity of SspI-positive infectious bursal disease virus and molecular characterization of the VP2 hypervariable region. Avian Pathology, 30, 369–380. doi: 10.1080/03079450120066377
- Ingrao, F., Rauw, F., Lambrecht, B. & Van Den Berg, T. (2013). Infectious bursal disease: a complex host-pathogen interaction. Developmental and Comparative Immunology, 41, 429–438. doi: 10.1016/j.dci.2013.03.017
- Islam, M.R., Zierenberg, K. & Muller, H. (2001). The genome segment B encoding the RNA-dependent RNA polymerase protein VP1 of very virulent infectious bursal disease virus (IBDV) is phylogenetically distinct from that of all other IBDV strains. Archives of Virology, 146, 2481–2492. doi: 10.1007/s007050170018
- Ismail, N.M. & Saif, Y.M. (1991). Immunogenicity of infectious bursal disease viruses in chickens. Avian Diseases, 35, 460–469. doi: 10.2307/1591208
- Jackwood, D.J. (2012). Molecular epidemiologic evidence of homologous recombination in infectious bursal disease viruses. Avian Diseases, 56, 574–577. doi: 10.1637/10053-010912-ResNote.1
- Jackwood, D.J., Sommer-Wagner, S.E., Crossley, B.M., Stoute, S.T., Woolcock, P.R. & Charlton, B.R. (2011). Identification and pathogenicity of a natural reassortant between a very virulent serotype 1 infectious bursal disease virus (IBDV) and a serotype 2 IBDV. Virology, 420, 98–105. doi: 10.1016/j.virol.2011.08.023
- Kasanga, C.J., Yamaguchi, T., Munang'andu, H.M., Ohya, K. & Fukushi, H. (2013). Genomic sequence of an infectious bursal disease virus isolate from Zambia: classical attenuated segment B reassortment in nature with existing very virulent segment A. Archives of Virology, 158, 685–689. doi: 10.1007/s00705-012-1531-4
- Kim, I.J., You, S.K., Kim, H., Yeh, H.Y. & Sharma, J.M. (2000). Characteristics of bursal T lymphocytes induced by infectious bursal disease virus. Journal of Virology, 74, 8884–8892. doi: 10.1128/JVI.74.19.8884-8892.2000
- Le Nouën, C., Rivallan, G., Toquin, D. & Eterradossi, N. (2005). Significance of the genetic relationships deduced from partial nucleotide sequencing of infectious bursal disease virus genome segments A or B. Archives of Virology, 150, 313–325. doi: 10.1007/s00705-004-0409-5
- Le Nouën, C., Rivallan, G., Toquin, D., Darlu, P., Morin, Y., Beven, W., de Boisseson, C., Cazaban, C., Comte, S., Gardin, Y. & Eterradossi, N. (2006). Very virulent infectious bursal disease virus: reduced pathogenicity in a rare natural segment-B-reassorted isolate. Journal of General Virology, 87, 209–216. doi: 10.1099/vir.0.81184-0
- Le Nouën, C., Toquin, D., Muller, H., Raue, R., Kean, K.M., Langlois, P., Cherbonnel, M. & Eterradossi N. (2012). Different domains of the RNA polymerase of infectious bursal disease virus contribute to virulence. PLoS One, 7, 1–16. doi: 10.1371/journal.pone.0028064
- Lim, B.L., Cao, Y.C., Yu, T. & Mo, C.W. (1999). Adaptation of very virulent infectious bursal disease virus to chicken embryonic fibroblasts by site-directed mutagenesis of residues 279 and 284 of viral coat protein VP2. Journal of Virology, 73, 2854–2862.
- Liu, M.H. & Vakharia, V.N. (2004). VP1 protein of infectious bursal disease virus modulates the virulence in vivo. Virology, 330, 62–73. doi: 10.1016/j.virol.2004.09.009
- Lu, Z., Zhang, L., Wang, N., Chen, Y., Gao, L., Wang, Y., Gao, H., Gao, Y., Li, K., Qi, X. & Wang, X. (2015). Naturally occurring reassortant infectious bursal disease virus in northern China. Virus Research, 203, 92–95. doi: 10.1016/j.virusres.2015.04.003
- Mahgoub, H.A., Bailey, M. & Kaiser, P. (2012). An overview of infectious bursal disease. Archives of Virology, 157, 2047–2057. doi: 10.1007/s00705-012-1377-9
- Mundt, E. (1999). Tissue culture infectivity of different strains of infectious bursal disease virus is determined by distinct amino acids in VP2. Journal of General Virology, 80, 2067–2076. doi: 10.1099/0022-1317-80-8-2067
- Poonia, B. & Charan, S. (2004). Infiltration by CD4+ and CD8+ lymphocytes in bursa of chickens infected with infectious bursal disease virus (IBDV): strain-specific differences. Indian journal of Experimental Biology, 42, 823–829.
- Qi, X., Gao, H., Gao, Y., Qin, L., Wang, Y., Gao, L. & Wang, X. (2009). Naturally occurring mutations at residues 253 and 284 in VP2 contribute to the cell tropism and virulence of very virulent infectious bursal disease virus. Antiviral Research, 84, 225–233. doi: 10.1016/j.antiviral.2009.09.006
- Qi, X., Gao, L., Qin, L., Deng, X., Wu, G., Zhang, L., Yu, F., Ren, X., Gao, Y., Gao, H., Wang, Y. & Wang, X. (2011). Genomic sequencing and molecular characteristics of a very virulent strain of infectious bursal disease virus isolated in China. Agricultural Science & Technology – Hunan, 12, 1946–1949.
- Reed, L.J. & Muench, H. (1938). A simple method of determining fifty percent end points. American Journal of Hygiene, 27, 493–497.
- Schnitzler, D., Bernstein, F., Muller, H. & Becht, H. (1993). The genetic basis for the antigenicity of the VP2 protein of the infectious bursal disease virus. Journal of General Virology, 74, 1563–1571. doi: 10.1099/0022-1317-74-8-1563
- Sharma, J.M., Kim, I.J., Rautenschlein, S. & Yeh, H.Y. (2000). Infectious bursal disease virus of chickens: pathogenesis and immunosuppression. Developmental and Comparative Immunology, 24, 223–235. doi: 10.1016/S0145-305X(99)00074-9
- Tanimura, N. & Sharma, J.M. (1997). Appearance of T cells in the bursa of fabricius and cecal tonsils during the acute phase of infectious bursal disease virus infection in chickens. Avian Diseases, 41, 638–645. doi: 10.2307/1592155
- Van Den Berg, T.P. (2000). Acute infectious bursal disease in poultry: a review. Avian Pathology, 29, 175–194. doi: 10.1080/03079450050045431
- Van Loon, A.A.W.M., de Haas, N., Zeyda, I. & Mundt, E. (2002). Alteration of amino acids in VP2 of very virulent infectious bursal disease virus results in tissue culture adaptation and attenuation in chickens. Journal of General Virology, 83, 121–129. doi: 10.1099/0022-1317-83-1-121
- Wei, Y.W., Li, J.R., Zheng, J.T., Xu, H., Li, L. & Yu, L. (2006). Genetic reassortment of infectious bursal disease virus in nature. Biochemical and Biophysical Research Communications, 350, 277–287. doi: 10.1016/j.bbrc.2006.09.040
- Wei, Y., Yu, X., Zheng, J., Chu, W., Xu, H. & Yu, L. (2008). Reassortant infectious bursal disease virus isolated in China. Virus Research, 131, 279–282. doi: 10.1016/j.virusres.2007.08.013
- Williams, A.E. & Davison, T.F. (2005). Enhanced immunopathology induced by very virulent infectious bursal disease virus. Avian Pathology, 34, 4–14. doi: 10.1080/03079450400025364
- Withers, D.R., Young, J.R. & Davison, T.F. (2005). Infectious bursal disease virus-induced immunosuppression in the chick is associated with the presence of undifferentiated follicles in the recovering bursa. Viral Immunology, 18, 127–137. doi: 10.1089/vim.2005.18.127
- Wyeth, P.J. & Chettle, N.J. (1990). Use of infectious bursal disease vaccines in chicks with maternally derived antibodies. Veterinary Record, 126, 577–578.