ABSTRACT
This review summarizes advances in understanding the pathogenesis of necrotic enteritis of chickens caused by netB-positive Clostridium perfringens. The discovery of NetB as the essential toxin trigger for the disease was followed by recognition that it forms part of a large plasmid-encoded 42 kb pathogenicity locus (NELoc-1). While the locus is critical for toxin production, it likely has additional functions related to colonization and degradation of the mucus barrier, which are essential both to multiplication and to bringing NetB close to the intestinal epithelium. Two “chitinases” (glycoside hydrolases (GHs)) present on NELoc-1 are predicted to be involved in mucin degradation, as is the large carbohydrate-binding metalloprotease, shown to be involved in mucinase activity in other clostridia. A second pathogenicity locus found in netB-positive C. perfringens, NELoc-2, also encodes a GH likely involved in mucin degradation. Upon reaching a sufficient cell density on the intestinal mucosa, the Agr-like quorum-sensing system is triggered, which in turn up-regulates the VirR/VirS regulon. This regulon includes NetB. Where NetB initiates damage is unresolved, but it may be deep in the intestinal mucosa, rather than superficially. As the disease progresses, C. perfringens line what remains of the intestinal epithelium in large numbers. This likely involves a number of different bacterial adhesins, including additional NELoc-1-encoded bacterial surface proteins, some of which may adhere to epithelial cell ligands exposed by bacterial sialidases. Further studies of the pathogenesis of necrotic enteritis should lead to development of novel ways to control the infection.
Introduction
The pathogenesis of an infectious disease describes how the disease occurs, and involves a detailed understanding of the host–pathogen interaction that leads to disease. The ideal is a holistic understanding at the molecular level of what is usually a complex, multifactorial and dynamic process. One value of understanding pathogenesis is to identify the weak points or “chinks in the armour” of the pathogen as it interacts with the host to cause disease. Control based on a detailed understanding of pathogenesis is a scientific rather than an empirical approach that, although it is expensive, usually pays off.
Understanding the pathogenesis of Clostridium perfringens in necrotic enteritis (NE) in chickens (Timbermont et al., Citation2011) is still at a relatively rudimentary stage, but considerable advances have been made in the last years and many more can be expected until such time as the disease is controlled without conventional antibiotics.
C. perfringens as a pathogen
C. perfringens is a robust Gram-positive aerotolerant anaerobic bacterium, and a ubiquitous inhabitant of the intestine and of soil, especially manured soil. It is a versatile enteric pathogen of different birds and has specialized for rapidly destroying bird tissues and acquiring nutrients (Songer, Citation1996; Shimizu et al., Citation2002). Part of the reason for its success is that it is one of the fastest-growing bacterial pathogens known (Shimizu et al., Citation2002). In the absence of readily available dead or live bird nutrient substrate, the bacterium can also survive dormant for long periods as a spore. The genetic basis for the specialist behaviour was identified in the first complete genome sequence of this “anaerobic flesh-eater” (Shimizu et al., Citation2002). Sequencing revealed that, unusually, C. perfringens lacks genes for the biosynthesis of many amino acids. In addition, it possesses both a plethora of different degradative enzymes, and numerous amino acid and sugar transporter systems, which together act to first release the nutrients it is unable to synthesize through tissue destruction, and then allow for their rapid uptake into the cell.
Although its ability to grow rapidly through the breakdown of bird tissues makes C. perfringens fearsome, any form of bacterial specialization has potentially weak points. Perhaps more notably than many other bacterial pathogens, virulence (the ability to cause disease) and metabolism are intimately linked in C. perfringens (Ohtani & Shimizu, Citation2015), because the rapid breakdown of tissues is the key to its ability to rapidly outcompete other bacteria that can decompose dead tissues. C. perfringens jumps the gun on these competitors by starting to break down tissues in live and not just dead birds, causing necrosis (tissue death) as its pathological hallmark. The intimate linking of virulence and metabolic functions is directed by the two-component regulatory system, VirR/VirS. VirR/VirS controls a regulon of about 150 genes, including toxins such as NetB, hyaluronidases, sialidases, catalytic enzymes, transporters and energy metabolism genes (Ohtani & Shimizu, Citation2015).
NetB and the “necrotic enteritis signature”
The discovery of the pore-forming toxin NetB and demonstration of its critical and essential role in the ability of chicken C. perfringens isolates to cause NE was a paradigm shift in understanding the pathogenesis of NE (Keyburn et al., Citation2008). NetB is a member of the beta-sheet pore-forming toxin family that includes a number of clostridial and other well-characterized bacterial toxins (Yan et al., Citation2013). It makes holes in cell membranes that cause leakage of the contents and destroy the cell.
Although NetB can be thought of as the essential factor that initiates disease, its delivery to target cells clearly involves a complex delivery system. The nature of some of these additional components has become clear through genomic sequencing.
Genomic sequencing showed that strains of C. perfringens that carried netB were characterized by a genetic “signature” () which consisted of: (i) a large 42 kb pathogenicity locus (NELoc1) carrying netB and found on a large tcp-conjugative plasmid of the type commonly found in C. perfringens causing enteric disease in birds; (ii) a smaller 11.2 kb region found in the chromosome (NELoc-2) and (iii) a short 5.6 kb region (NELoc-3) present on a second, different, large tcp-conjugative plasmid encoding a toxin called CPB2 (Lepp et al., Citation2010; Parreira et al., Citation2012). Further genetic work showed that isolates carrying netB possessed a number of other chromosomal genes that were thought to increase “fitness” to cause disease, for example sugar transporters and iron-acquisition genes (Lepp et al., Citation2013). These and other studies (Hibberd et al., Citation2011) demonstrated that NE isolates belonged to at least two clonal lineages, subsequently expanded to a third (Lacey et al., Citation2015). It is apparent though not yet proven that possession of an additional toxin, TpeL, a member of a large clostridial glucosylating cytotoxin family found on another tcp-conjugative plasmid, enhances the virulence of netB-containing strains (Chalmers et al., Citation2008; Coursodon et al., Citation2012).
Figure 1. Genetic organization of NE-specific loci. The genetic organization of (A) NELoc-1, (B) NELoc-2 and (C) NELoc-3. Each arrow represents a predicted gene and the total size is given below each locus. Predicted functional annotations and locus tags are shown above and below each gene, respectively. Genes are colour-coded by their putative role based upon sequence analyses.
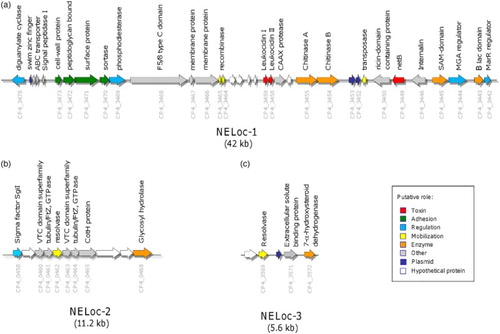
Factors predisposing to NE
Many of the factors that predispose chickens to NE are well understood and the basis of this predisposition is generally clear and is discussed elsewhere in this volume (Moore, Citation2016; Prescott et al., Citation2016). For example, the well-known predisposition to NE by coccidia must partly relate to provision of nutrients for C. perfringens through the increased mucogenesis often associated with coccidial infection (Collier et al., Citation2008; Forder et al., Citation2012) as well as the release of essential amino acids through coccidia-evoked tissue damage.
The pathogenesis of NE
The pathogenesis of a bacterial infection is commonly broken down into different stages illustrated in . These involve colonization of the site of disease, multiplication, acquisition of nutrients to allow further multiplication, evasion of host defences, damage to the host and then transmission. Although it can be broken down into different elements, the process is likely essentially simultaneous. Much of what follows is acknowledged to be speculative but are reasonable speculations that may provide a conceptual framework around which future research can be based.
Figure 2. The pathogenesis of a bacterial infection is commonly broken down into the different stages illustrated. Although it can be broken down into different components (colonization, multiplication, acquisition of nutrients to allow further multiplication, evasion of host defences, damage, and transmission), the process is dynamic and likely almost simultaneous particularly in the case of a fast-growing bacterial pathogen such as C. perfringens.
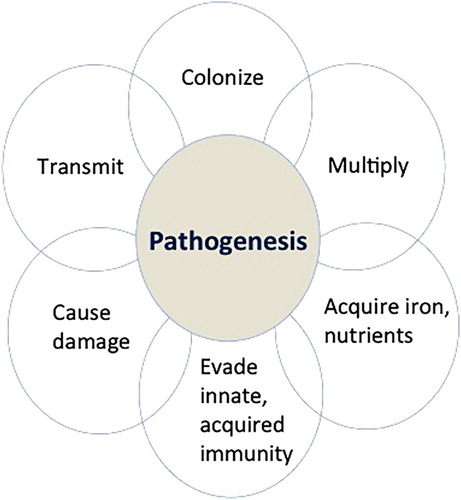
Colonization and degradation of the intestinal mucus layer
There may be an initial phase of displacement of other C. perfringens from the intestine or mucosal surfaces by bacteriocins (Timbermont et al., Citation2011, Citation2014). It seems very likely that colonization and degradation of the mucus layer of the small intestine are an essential prerequisite to the development of NE. Mucus secreted by intestinal epithelial cells onto their surface represents a major barrier to their colonization by bacteria (McGuckin et al., Citation2011; Ficko-Blean et al., Citation2012). Mucus is rich in O-glycoylated mucin glycoproteins and contains a diverse array of antimicrobial molecules designed to add a barrier to bacterial colonization of the intestinal epithelial cells. The wide range of oligosaccharide sugars on the central glycosylated regions of mucin glycoproteins presents numerous potential binding sites for microbial adhesins as well as an energy source for bacteria that can metabolize them (McGuckin et al., Citation2011; Ficko-Blean et al., Citation2012). Not only does C. perfringens generally possess GHs capable of breaking down specific O-glycans in mucin (Fujita et al., Citation2011; Ficko-Blean et al., Citation2012) but also NE isolates possess additional enzymes capable of degrading mucins. Notably, there are two “chitinase” genes present on the large, plasmid-encoded, NELoc-1 (). These chitinases belong to the GH families 18 and 19, of which family 18 is widespread among bacterial pathogens (Frederiksen et al., Citation2013). Although annotated as “chitinases” (Lepp et al., Citation2010), these are likely to be involved as bacterial endoglycosidases hydrolyzing the chitobiose core of N-linked glycoproteins, and thus involved in mucus colonization and degradation. The importance of mucus colonization and degradation as a likely important part of the pathogenesis of NE is underscored by the presence of an additional glycosyl hydrolase on NELoc 2 (). Glycoproteins are not only found in mucus but are also epithelial cell-surface membranes, so that these GHs may have broader degradative functions. Another prominent NELoc-1 gene is the large zinc metalloprotease, 1645 amino acids in size, annotated as a F5/8 type C domain-containing protein (Lepp et al., Citation2010). This zinc metalloprotease was shown to be a protective antigen against NE (Kulkarni et al., Citation2008) and also has the unusual feature of possessing a carbohydrate-binding domain of the M60-like superfamily, which has been shown to be involved in mucinase activity (Nakjang et al., Citation2012).
Colony formation and quorum sensing
Colonization and degradation of mucus in the small intestine are likely a critical source of nutrients for C. perfringens (McGuckin et al., Citation2011; Ficko-Blean et al., Citation2012) that allows the bacterium to form localized microcolonies on the mucosal surface. Many bacteria regulate gene expression through the system of “quorum sensing”, whereby a population, once it reaches a sufficient density, sends out a “signal” of small auto-inducing molecules that up-regulate the expression of the VirR-VirS regulon and of virulence and related metabolism genes. In C. perfringens, the agr-like (accessory gene regulator) QS system switches on the VirR/VirS two-component regulatory system, and initiates the disease process (Ohtani et al., Citation2009; Ohtani & Shimizu, Citation2015). netB is under the control of the VirR/VirS two-component regulatory system (Cheung et al., Citation2010) as are numerous other virulence-associated and metabolic genes (Ohtani & Shimizu, Citation2015), and there is evidence to suggest the internalin-like gene on NELoc-1, likely involved in adhesion, is also regulated by VirR/VirS (Lepp et al., Citation2010). The small 5-mer auto-inducing peptide of the agr system in C. perfringens has been identified (Ma et al., Citation2015). In addition, an artificial 6-mer peptide that can inhibit toxin production has also been identified, a potential example of the value of understanding pathogenesis to science-based control of disease (Ma et al., Citation2015). Yu et al. (Citation2015) have demonstrated that Agr-like but not the LuxS/AI-2 QS system regulates NetB production. Infection with Eimeria species may assist in the process of bacterial colonization and QS for reasons mentioned earlier.
Where does NetB start the damage?
It seems clear that NetB has a critical role in starting the damage that initiates NE (Keyburn et al., Citation2008), and that this follows colonization and degradation of intestinal mucus so that the pore-forming NetB can access cells. Careful studies by Olkowski et al. (Citation2006, Citation2008) of the histopathology of NE, however, suggest that damage to the villi occurs initially at the basement membrane and lateral part of the enterocytes, spreading through the lamina propria. Surface epithelial damage occurs only later in the disease process, so that death of enterocytes is actually a consequence of destruction of the lamina propria, extracellular matrix and intercellular junctions. This suggested pathogenesis is similar to the pathogenesis of the beta-toxin (CPB) in enteritis of neonatal pigs where this NetB-related pore-forming toxin targets endothelial cells rather than intestinal epithelium per se (Roos et al., Citation2015). The receptor for NetB has not been identified and it may be in endothelial rather than intestinal epithelial cells. Olkowski et al. (Citation2008) suggested that initiation of NE involves proteolytic factors through as-yet-unidentified proteolytic and collagenolytic enzymes. If this hypothesis is correct, the prominent NELoc-1 zinc metalloprotease might be important in this regard, although NetB is clearly the initiating factor.
Deeper mucosal intestinal epithelium colonization
A hallmark of the histopathology of the small intestine of chickens with NE is the large number of C. perfringens organisms that line the submucosa after the more superficial mucosa has become necrotic and has sloughed off (). These bacteria may represent a tightly adherent biofilm, suggesting that biofilm formation might be an essential component of the pathogenesis of the infection, especially in its later stages. Biofilms are naturally formed adherent communities of bacteria within an extracellular polymeric matrix (van Hoek, Citation2013). Their formation is complex and in other Gram-positive bacteria involves pili, extracellular carbohydrates, chitinases, and cyclic-di-GMP small molecules, among others (van Hoek, Citation2013). Biofilms commonly represent a mechanism for bacterial persistence and survival rather than for virulence. Whether the prominent C. perfringens observed layered on the surface of the necrotic but unsloughed mucosa in NE represents a true biofilm rather than bacteria that are tightly adherent to different ligands exposed through the disease process remains to be determined (Charlebois et al., Citation2015). The Agr-like quorum-sensing system is involved in regulating production of the alpha toxin (CPA) and perfringolysin (PFO) in building biofilms (Vidal et al., Citation2015).
Figure 3. Gram-stained histological section of the jejunum of a chicken with NE, photographed at about 40×. A hallmark of the histopathology of the small intestine of chickens with NE is the large number of C. perfringens (dark-blue bacteria, indicated by the yellow arrows) that are present deep in the submucosa after the more superficial mucosa has become necrotic and has sloughed off. The numerous extracellular matrix proteins exposed by the NetB-induced damage are likely the target receptors for different bacterial adhesins that must allow C. perfringens to adhere in such a characteristic manner.
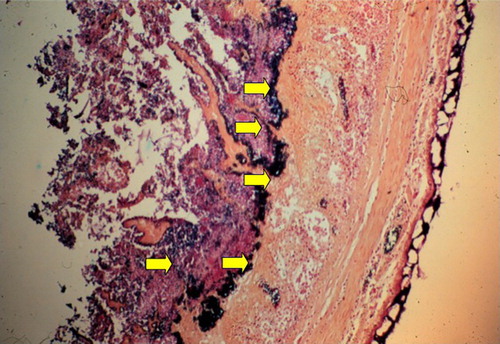
The numerous extracellular matrix proteins exposed by the NetB-induced damage are likely the target of adhesins that must allow C. perfringens to adhere in such a characteristic manner (). Nearly half of the genes encoded on NELoc-1 appear to be involved in expression of surface proteins predicted to encode adhesins (Lepp et al., Citation2010). Wade et al. (Citation2015a) have identified the ability of virulent, NetB-positive C. perfringens strains to adhere to collagen types IV and V in vitro and associated this with a putative fimbrial adhesin VR-10B operon. Mutation of a gene in the operon, which is apparently unique to chicken source C. perfringens and always present in netB-positive isolates (Lepp et al., Citation2013), showed not only that this operon was responsible for collagen adhesion but also rendered the mutated strain apparently avirulent in an NE model (Wade et al., Citation2015b).
The sialidases of C. perfringens, such as NanI, which are regulated by the VirR/VirS system among others, appear to be involved in promoting adhesion of C. perfringens through modification of epithelial cell surfaces to allow binding to sialidase-exposed receptors on enterocyte cell surfaces (Li & McClane, Citation2014a). In Type C strains, they also up-regulate epsilon toxin production and enhance toxin action on host cells (Li et al., Citation2015), although the role of sialidases in the pathogenesis of NE has not been explored.
The genes for putative C. perfringens cell-surface anchored proteins are found between the two cyclic-di-GMP regulatory genes on NELoc-1 () (Lepp et al., Citation2010) and are likely to be involved in binding to the damaged intestinal mucosa. Homologous predicted cell-surface anchor protein genes have been found on other C. perfringens plasmids, for example in the pathogenicity locus associated with a tcp-plasmid-encoded pore-forming toxin gene, netF (Mehdizadeh Gohari et al., Citation2016). Cyclic di-GMP signalling may have numerous effects in the pathogenesis of NE since it is a highly flexible regulatory system that controls virulence, motility and biofilm formation in some bacteria (Römling, Citation2012; Ryan, Citation2013) and, in Clostridium difficile, sortase-anchored adhesins (Peltier et al., Citation2015).
Conclusions
Numerous questions about the detailed pathogenesis of NE need to be resolved, although the elements are becoming clearer. Because of the nature of the bacterium, the disease process is very acute, so that breaking the disease down to its “steps” may be very difficult, particularly since these are dynamic and may occur almost simultaneously. Current models may be inadequate to reveal the subtleties of the pathogenesis of NE. What is the role in pathogenesis of each of the genes or operons on the NELoc-1, NELoc-2 and NELoc-3, and how do these interact with each other in the disease process? We need to understand the detailed regulation of virulence, in part since regulatory processes may be one of the bacterium's “weak points” for targeted intervention. Why does NE occur in the small intestine? Is it as simple as proteolytic activity of the large bowel flora breaking down NetB? What is the role of bacteriocins and the microbiota in predisposing to NE? What are the adhesins for the organism that promote both the initial and the deeper, ongoing, colonization? Are there multiple adhesins or are one or two most critical? How is mucus colonized? Does biofilm formation occur, is it important and can it be inhibited? Can we block quorum sensing and prevent NE with artificial peptides? (Ma et al., Citation2015; Singh et al., Citation2015) Can we target cyclic-di-GMP signalling (Fatima et al., Citation2013) to attenuate virulence? Clearly answering these and many other questions are important as we design unconventional, non-antibiotic, approaches to the control of NE.
Additional information
Funding
References
- Chalmers, G., Bruce, H.L., Hunter, D.B., Parreira, V.R., Kulkarni, R.R., Jiang, Y.F., Prescott, J.F. & Boerlin, P. (2008). Multilocus sequence typing analysis of Clostridium perfringens isolates from necrotic enteritis outbreaks in broiler chicken populations. Journal of Clinical Microbiology, 46, 3957–3964. doi: 10.1128/JCM.01548-08
- Charlebois, A., Jacques, M. & Archambault M. (2015). Comparative transcriptomic analysis of Clostridium perfringens biofilms and planktonic cells. In 1st International Conference on Necrotic Enteritis of Poultry, Copenhagen, Denmark. Abstract.
- Cheung, J.K., Keyburn, A.L., Carter, G.P., Lanckriet, A.L., Van Immerseel, F., Moore, R.J. & Rood, J.I. (2010). The VirSR two-component signal transduction system regulates NetB toxin production in Clostridium perfringens. Infection and Immunity, 78, 3064–3072. doi: 10.1128/IAI.00123-10
- Collier, C.T., Hofacre, C.L., Payne, A.M., Anderson, D.B., Kaiser, P., Mackie, R.I. & Gaskins, H.R. (2008). Coccidia-induced mucogenesis promotes the onset of necrotic enteritis by supporting Clostridium perfringens growth. Veterinary Immunology & Immunopathology, 122, 104–115. doi: 10.1016/j.vetimm.2007.10.014
- Coursodon, C.F., Glock, R.D., Moore, K.L., Cooper, K.K. & Songer, J.G. (2012). TpeL-producing strains of Clostridium perfringens type A are highly virulent for broiler chicks. Anaerobe, 18, 117–121. doi: 10.1016/j.anaerobe.2011.10.001
- Fatima, M., Rempel, H., Kuang, X.T., Allen, K.J., Cheng, K.M., Malouin, F. & Diarra, M.S. (2013). Effect of 3’,5'-cyclic diguanylic acid in a broiler Clostridium perfringens infection model. Poultry Science, 92, 2644–2650. doi: 10.3382/ps.2013-03143
- Ficko-Blean, E., Stuart, C.P., Suits, M.D., Cid, M., Tessier, M., Woods, R.J. & Boraston, A.B. (2012). Carbohydrate recognition by an architecturally complex α-N-acetylglucosaminidase from Clostridium perfringens. PLoS One, 7, e33524. doi: 10.1371/journal.pone.0033524
- Forder, R.E., Nattrass, G.S., Geier, M.S., Hughes, R.J. & Hynd, P.I. (2012). Quantitative analyses of genes associated with mucin synthesis of broiler chickens with induced necrotic enteritis. Poultry Science, 91, 1335–1341. doi: 10.3382/ps.2011-02062
- Frederiksen, R.F., Paspaliari, D.K., Larsen, T., Storgaard, B.G., Larsen, M.H., Ingmer, H., Palcic, M.M. & Leisner, J.J. (2013). Bacterial chitinases and chitin-binding proteins as virulence factors. Microbiology, 159, 833–847. doi: 10.1099/mic.0.051839-0
- Fujita, M., Tsuchida, A., Hirata, A., Kobayashi, N., Goto, K., Osumi, K., Hirose, Y., Nakayama, J., Yamanoi, T., Ashida, H. & Mizuno, M. (2011). Glycoside hydrolase family 89 α-N-acetylglucosaminidase from Clostridium perfringens specifically acts on GlcNacα1,4Galβ1R at the non-reducing terminus of O-glycans in gastric mucin. Journal of Biological Chemistry, 286, 6479–6489. doi: 10.1074/jbc.M110.206722
- Hibberd, M.C., Neumann, A.P., Rehberger, T.G. & Siragusa, G.R. (2011). Multilocus sequence typing subtypes of poultry Clostridium perfringens isolates demonstrate disease niche partitioning. Journal of Clinical Microbiology, 49, 1556–1567. doi: 10.1128/JCM.01884-10
- Hiscox, T.J., Chakravorty, A., Choo, J.M., Ohtani, K., Shimizu, T., Cheung, J.K. & Rood, J.I. (2011). Regulation of virulence by the RevR response regulator in Clostridium perfringens. Infection & Immunity, 79, 2145–2153. doi: 10.1128/IAI.00060-11
- van Hoek, M.L. (2013). Biofilms: an advancement in our understanding of Francisella species. Virulence, 4, 833–846. doi: 10.4161/viru.27023
- Keyburn, A.L., Boyce, J.D., Vaz, P., Bannam, T.L., Ford, M.E., Parker, D., Di Rubbo, A., Rood, J.I. & Moore, R.J. (2008). NetB, a new toxin that is associated with avian necrotic enteritis caused by Clostridium perfringens. PLoS Pathogens, 4, e26. doi: 10.1371/journal.ppat.0040026
- Kulkarni, R., Parreira, V., Sharif, S. & Prescott, J.F. (2008). Oral immunization of broiler chickens against necrotic enteritis with an attenuated Salmonella vaccine vector expressing Clostridium perfringens antigens. Vaccine, 26, 4194–4203. doi: 10.1016/j.vaccine.2008.05.079
- Lacey, J., Allnutt, T., Wade, B., Keyburn, A., Chen, H., Seemann, T., Stent, T., Han, X., Rood, J., Johanesen, P., Lyras, D. & Moore, R. (2015). Comparative genomics of chicken isolates of Clostridium perfringens. In 1st International Conference on Necrotic Enteritis of Poultry, Copenhagen, Denmark. Abstract.
- Lepp, D., Gong, J., Songer, J.G., Boerlin, P., Parreira, V.R. & Prescott, J.F. (2013). Identification of accessory genome regions in poultry Clostridium perfringens isolates carrying the netB plasmid. Journal of Bacteriology, 195, 1152–1166. doi: 10.1128/JB.01032-12
- Lepp, D., Roxas, B., Parreira, V.R., Marri, P.R., Rosey, E.L., Gong, J., Songer, J.G., Vedantam, G. & Prescott, J.F. (2010). Identification of novel pathogenicity loci in Clostridium perfringens strains that cause avian necrotic enteritis. PLoS One, 5, e10795. doi: 10.1371/journal.pone.0010795
- Li, J., Freedman, J.C. & McClane, B.A. (2015). NanI sialidase, CcpA, and CodY work together to regulate epsilon toxin production by Clostridium perfringens type D Strain CN3718. Journal of Bacteriology, 197, 3339–3353. doi: 10.1128/JB.00349-15
- Li, J. & McClane, B.A. (2014). Contributions of NanI sialidase to Caco-2 cell adherence by Clostridium perfringens type A and C strains causing human intestinal disease. Infection & Immunity, 82, 4620–4630. doi: 10.1128/IAI.02322-14
- Ma, M., Li, J. & McClane, B.A. (2015). Structure-function analysis of peptide signaling in the Clostridium perfringens Agr-like quorum sensing system. Journal of Bacteriology, 197, 1807–1818. doi: 10.1128/JB.02614-14
- McGuckin, M.A., Lindén, S.K., Sutton, P. & Florin, T.H. (2011). Mucin dynamics and enteric pathogens. Nature Reviews Microbiology, 9, 265–278. doi: 10.1038/nrmicro2538
- Mehdizadeh Gohari, I.M., Kropinski, A.M., Weese, J.S., Parreira, V.R., Whitehead, A.E., Boerlin, P. & Prescott, J.F. (2016). Comparative genomic analysis of two netF + Clostridium perfringens isolates associated with foal and canine necrotizing enteritis. PLoS One, 11, e0148344.
- Moore, R. (2016). Necrotic enteritis predisposing factors in broiler chickens Avian Pathology, 45, 275–281.
- Nakjang, S., Ndeh, D.A., Wipat, A., Bolam, D.N. & Hirt, R.P. (2012). A novel extracellular metallopeptidase domain shared by animal host-associated mutualistic and pathogenic microbes. PLoS One, 7, e30287. doi: 10.1371/journal.pone.0030287
- Ohtani, K. & Shimizu, T. (2015). Regulation of toxin gene expression in Clostridium perfringens. Research in Microbiology, 166, 280–289. doi: 10.1016/j.resmic.2014.09.010
- Ohtani, K., Yuan, Y., Hassan, S., Wang, R., Wang, Y. & Shimizu, T. (2009). Virulence gene regulation by the agr system in Clostridium perfringens. Journal of Bacteriology, 191, 3919–3927. doi: 10.1128/JB.01455-08
- Olkowski, A.A., Wojnarowicz, C., Chirino-Trejo, M. & Drew, M.D. (2006). Responses of broiler chickens orally challenged with Clostridium perfringens isolated from field cases of necrotic enteritis. Research in Veterinary Science, 81, 99–108. doi: 10.1016/j.rvsc.2005.10.006
- Olkowski, A.A., Wojnarowicz, C., Chirino-Trejo, M., Laarveld, B. & Sawicki, G. (2008). Sub-clinical necrotic enteritis in broiler chickens: novel etiological consideration based on ultra-structural and molecular changes in the intestinal tissue. Research in Veterinary Science, 85, 543–553. doi: 10.1016/j.rvsc.2008.02.007
- Parreira, V.R., Costa, M., Eikmeyer, F., Blom, J. & Prescott, J.F. (2012). Sequence of two plasmids from Clostridium perfringens chicken necrotic enteritis isolates and comparison with C. perfringens conjugative plasmids. PLoS One, 7, e49753. doi: 10.1371/journal.pone.0049753
- Peltier, J., Shaw, H.A., Couchman, E.C., Dawson, L.F., Yu, L., Choudhary, J.S., Kaever, V., Wren, B.W. & Fairweather, N.F. (2015). Cyclic-di-GMP regulates production of sortase substrates of Clostridium difficile and their surface exposure through ZmpI protease-mediated cleavage. Journal of Biological Chemistry, 290, 24453–24469. doi: 10.1074/jbc.M115.665091
- Römling, U. (2012). Cyclic di-GMP, an established secondary messenger still speeding up. Environmental Microbiology, 14, 1817–1829. doi: 10.1111/j.1462-2920.2011.02617.x
- Roos, S., Wyder, M., Candi, A., Regenscheit, N., Nathues, C., Van Immerseel, F. & Posthaus H. (2015). Binding studies on isolated porcine small intestinal mucosa and in vitro toxicity studies reveal lack of effect of C. perfringens beta-toxin on the porcine intestinal epithelium. Toxins, 7, 1235–1252. doi: 10.3390/toxins7041235
- Ryan, R.P. (2013). Cyclic di-GMP signalling and the regulation of bacterial virulence. Microbiology, 159, 1286–1297. doi: 10.1099/mic.0.068189-0
- Shimizu, T., Ohtani, K., Hirakawa, H., Ohshima, K., Yamashita, A., Shiba, T., Ogasawara, N., Hattori, M., Kuhara, S. & Hayashi, H. (2002). Complete genome sequence of Clostridium perfringens, an anaerobic flesh-eater. Proceedings of the National Academy of Sciences of the USA, 99, 996–1001. doi: 10.1073/pnas.022493799
- Singh, R.P., Okubo, K., Ohtani, K., Adachi, K., Sonomoto, K. & Nakayama, J. (2015). Rationale design of quorum-quenching peptides that target the VirSR system of Clostridium perfringens. FEMS Microbiology Letters, 362, 1–7. doi: 10.1093/femsle/fnv188
- Songer, J.G. (1996). Clostridial enteric diseases of domestic animals. Clinical Microbiology Reviews, 9, 216–234.
- Timbermont, L., De Smet, L., Van Nieuwerbergh, F., Parreira, V.R., Van Driessche, G., Haesebrouck, F., Ducatelle, R., Prescott, J., Deforce, D., Devreese, B. & Van Immerseel, F. (2014). Perfrin, a novel bacteriocin associated with netB-positive Clostridium perfringens strains from broiler chickens with necrotic enteritis. Veterinary Research, 45, 40. doi: 10.1186/1297-9716-45-40
- Timbermont, L., Haesebrouck, F., Ducatelle, R. & Van Immerseel, F. (2011). Necrotic enteritis in broilers: an updated review on the pathogenesis. Avian Pathology, 40, 341–347. doi: 10.1080/03079457.2011.590967
- Vidal, J.E., Shak, J.R. & Canizalez-Roman, A. (2015). The CpAL quorum sensing system regulates production of hemolysins CPA and PFO to build Clostridium perfringens biofilms. Infection & Immunity, 83, 2430–2442. doi: 10.1128/IAI.00240-15
- Wade, B., Keyburn, A.L., Seemann, T., Rood, J.I. & Moore, R.J. (2015a). Binding of Clostridium perfringens to collagen correlates with the ability to cause necrotic enteritis in chickens. Veterinary Microbiology, 180, 299–303. In 1st International Conference on Necrotic Enteritis of Poultry, Copenhagen, Denmark. Abstract.
- Wade, B., Keyburn, A.L., Haring, V., Ford, M., Rood, J.I. & Moore, R.J. (2015b). Fimbriae genes play a role in collagen adherence, colonization, and virulence of Clostridium perfringens in avian necrotic enteritis. In 1st International Conference on Necrotic Enteritis of Poultry, Copenhagen, Denmark. Abstract.
- Yan, X.-X., Porter, C.J., Hardy, S.P., Steer, D., Smith, A.I., Quinsey, N.S., Hughes, V., Cheung, J.K., Keyburn, A.L., Kaldhusdal, M., Moore, R.J., Bannam, T.L., Whisstock, J.C. & Rood, J.I. (2013). Structural and functional analysis of the pore-forming toxin NetB from Clostridium perfringens. mBio, 4, e00019–13. doi: 10.1128/mBio.00019-13
- Yu, Q., Lepp, D., Gohari, I.M., Yin, X., Yu, H., Prescott, J.F., Nie, S., Xie, M. & Gong, J. (2015). Agr-like, but not LuxS, quorum sensing regulates NetB toxin production of Clostridium perfringens type A strain CP1 through the VirS/VirR system. In 1st International Conference on Necrotic Enteritis of Poultry, Copenhagen, Denmark.