ABSTRACT
Avian malaria is a mosquito-borne disease caused by protozoans of the genus Plasmodium, and it is considered one of the most important causes of morbidity and mortality in captive penguins, both in zoological gardens and rehabilitation centres. Penguins are known to be highly susceptible to this disease, and outbreaks have been associated with mortality as high as 50–80% of affected captive populations within a few weeks. The disease has also been reported in wild penguin populations, however, its impacts on the health and fitness of penguins in the wild is not clear. This review provides an overview of the aetiology, life cycle and epidemiology of avian malaria, and provides details on the strategies that can be employed for the diagnostic, treatment and prevention of this disease in captive penguins, discussing possible directions for future research.
Introduction
Avian malaria is a widespread mosquito-borne disease caused by protozoans of the genus Plasmodium (Cranfield, Citation2003; Valkiūnas, Citation2005; Atkinson, Citation2008; Marzal, Citation2012). While relatively common worldwide, these parasites are generally not associated with events of mass mortality in bird populations who co-evolve with the species of Plasmodium prevalent in their habitat (Bennet et al., Citation1993b; Valkiunas, Citation2005). However, there are a number of reports documenting the contribution of Plasmodium spp. to the mortality of wild birds originating from avian Plasmodium spp. endemic areas (Gabaldon & Ulloa, Citation1980; Dinhopl et al., Citation2015). Penguins, in particular, are highly susceptible to the development of malarial infection, resulting in severe and sometimes lethal disease (Fordyce & Jones, Citation1990; Valkiūnas, Citation2005).
The reasons why penguin species are highly susceptible to malarial parasites remain unclear. There has been speculation that because penguins inhabit cold, arid and/or windy environments, they remained relatively isolated from contact with mosquitoes, therefore encountered little exposure to mosquito-borne infections such as Plasmodium spp. (Jones & Shellam, Citation1999; Jovani et al., Citation2001). Without host–parasite co-evolution, penguins would not have had the chance to develop immunity to Plasmodium spp. infection, nor would the parasite be selected for the ability to successfully infect penguins without causing their death.
Captive penguins
Penguins are among the most popular of avian groups in zoological exhibits (Gailey-Phipps, Citation1978). Their exhibition in zoological gardens worldwide or admission to rehabilitation centres in areas where avian malaria transmission occurs may enhance the exposure to vectors and increase the avian malaria infection risk, resulting in infections, with high morbidity and mortality rates (Rodhain, Citation1939; Griner & Sheridan, Citation1967; Bak et al., Citation1984; Fix et al., Citation1988). There is also a possibility that penguins already infected in their natural environment only develop disease when subjected to the stress associated with the rehabilitation process, (Parsons & Underhill, Citation2005; Thiart, Citation2005). The detection of identical Plasmodium spp. sequences in native avifauna and captive penguins suggest that the local avifauna acts as a source of the infection (Bueno et al., Citation2010; Dinhopl et al., Citation2015). Hence, avian malaria is a major concern in captive penguin colonies, representing one of the most important causes of mortality in penguin colonies exhibited outdoors (Stoskopf & Beier, Citation1979; Cranfield, Citation2003). A recent survey on 40 zoos in the Northern Hemisphere revealed that 12.5% of the zoos, at some point, diagnosed cases of avian malaria in their penguin collections (African penguins Spheniscus demersus and Humboldt penguins Spheniscus humboldti) and 37.5% systematically test their penguin collections for Plasmodium spp. (Grilo, Citation2014). A similar dynamic takes place in rehabilitation centres in South America and South Africa, where Plasmodium spp. infections have been identified in 17–34% of African penguins and 7–13% of Magellanic penguins (Spheniscus magellanicus) undergoing in rehabilitation (Parsons & Underhill, Citation2005; Vanstreels et al., Citation2015).
Wild populations
Pathogens are considered globally one of the main causes of wildlife population extinctions (Smith et al., Citation2006). Vector and bird migration and vector introduction by anthropogenic action into non-endemic habitats represents a risk for endangered species (Warner, Citation1968; Atkinson et al., Citation2000; Levin et al., Citation2013). The potential of Plasmodium spp. to negatively affect naïve populations of wild birds has been demonstrated in Hawaii, where Plasmodium relictum is thought to have played a key role in the decline and extinction of endemic land birds following the introduction of mosquitoes to the archipelago (Van Riper et al., Citation1986; Atkinson & LaPointe, Citation2009). The high susceptibility of penguins to malarial parasites has led to concern that Plasmodium spp. could become a significant conservation threat to the conservation of penguins, particularly in tropical and subtropical regions (Brossy et al., Citation1999; Jones & Shellam, Citation1999; Sturrock & Tompkins, Citation2007; Levin et al., Citation2009; Meile et al., Citation2013; Palmer et al., Citation2013; Vanstreels et al., Citation2014).
Plasmodium spp. have been reported in wild penguins species in temperate and tropical regions: P. relictum in African penguins in South Africa (Fantham & Porter, Citation1944; Thiart, Citation2005), P. relictum in Northern rockhopper penguins (Eudyptes moseleyi) at Gough Island (Fantham & Porter, Citation1944), P. relictum in Snares (Eudyptes robustus), yellow-eyed (Megadyptes antipodes) and little penguins (Eudyptula minor) in New Zealand (Fantham & Porter, Citation1944; Laird, Citation1950; Van Rensburg, Citation2010) and, more recently, Plasmodium spp. in Galapagos penguins (Spheniscus mendiculus) at the Galapagos Islands (Levin et al., Citation2009, Citation2013).
In contrast to the rapid and severe outbreaks of avian malaria in captive penguins, the significance of this disease to the health and fitness of penguins in the wild is not clear as detailed population studies have not been conducted. None of the wild penguins in which Plasmodium spp. were detected had external signs of disease, and parasitaemia was generally low (Fantham & Porter, Citation1944; Laird, Citation1950; Brossy, Citation1992). Fantham and Porter (Citation1944) detected P. relictum in a deceased wild African penguin. As the penguin had suffered severe trauma, it is not clear whether or not avian malaria contributed to its death. However, it is worth considering that as much as 35% of the African penguins admitted to rehabilitation at the Western Cape in South Africa are infected with Plasmodium spp. (Parsons & Underhill, Citation2005), whereas infection in wild African penguins in that region seems to occur at much lower prevalence (<1%) (Fantham & Porter, Citation1944; Brossy, Citation1992; Thiart, Citation2005), indicating that infection probably contributes in debilitating these birds. On the other hand, the Plasmodium spp. infection in Galapagos penguins appears to be abortive, meaning that parasite blood replication in the host does not occur (Levin et al., Citation2009, Citation2013). However, further investigation is necessary to clarify this. Active surveillance is essential to understand the prevalence, dynamics and effects of avian malaria in wild penguin populations.
Aetiology
Haemosporidians represent a group of obligatory heteroxenous protists that use dipteran blood-sucking insects as vectors. The members of the Plasmodiidae family go through merogony in vertebrate hosts’ tissue and erythrocytes. When developing as erythrocytic meronts and gametocytes, they produce malarial pigments (haemozoin) (Valkiūnas, Citation2005).
There are more than 200 species in the genus Plasmodium, and these parasites have been described to infect reptiles, birds and mammals (Martinsen & Perkins, Citation2013). Even though they belong to the same genus, it should be clear that the species of Plasmodium associated with avian malaria belong to a different phylogenetic group than those associated with mammalian malaria (Outlaw & Ricklefs, Citation2011; Martinsen & Perkins, Citation2013), and that no infections by avian-infecting Plasmodium species have been reported in humans (Cox, Citation1998).
In penguins, seven malarial parasites have been documented: P. (Haemamoeba) relictum (Fantham & Porter, Citation1944), P. (Huffia) elongatum (Huff & Shiroishi, Citation1962), P. (Bennettinia) juxtanucleare (Grim et al., Citation2003), P. (Haemamoeba) tejerai (Silveira et al., Citation2013), P. (Haemamoeba) cathemerium, P. (Novyella) nucleophilum and P. (Novyella) unalis (Vanstreels et al., Citation2015). The majority of documented cases are caused by P. relictum and P. elongatum (Rodhain, Citation1939; Fantham & Porter, Citation1944; Laird, Citation1950; Huff & Shiroishi, Citation1962; Griner & Sheridan, Citation1967; Beier & Stoskopf, Citation1980; Bak et al., Citation1984; Grim et al., Citation2003; Chitty, Citation2011; Vanstreels et al., Citation2015). The first species is considered more prevalent (Cranfield, Citation2003). There is evidence to suggest that species belonging to the subgenus Haemamoeba, particularly P. relictum, tend to be more pathogenic to penguins and produce outbreaks with higher mortality than other malarial species (Beier & Stoskopf, Citation1980; Graczyk et al., Citation1994a).
Vectors
Mosquitoes (Diptera: Culicidae), are the vectors for malaria parasites. Only the females are haematophagus (feed on blood) and, consequently, participate in spreading the infection (Valkiūnas, Citation2005; Atkinson, Citation2008). Nine genera of mosquitoes have been reported as potential vectors of avian-infecting Plasmodium spp. (Aedeomyia, Aedes, Anopheles, Armigeres, Culex, Culiseta, Mansonia, Psorophora and Wyeomyia) (Huff, Citation1965; Valkiūnas, Citation2005; Huijben et al., Citation2007; Kimura et al., Citation2010).
Studies in zoos indicate that Culex spp. seem to be the most significant vectors in the transmission of Plasmodium spp. to captive penguins, particularly C. pipiens (Rodhain, Citation1939; Raethel, Citation1960; Beier & Trpis, Citation1981), C. quinquefasciatus (=C. fatigans) (Laird & Van Riper, Citation1981), C. tarsalis (Huff & Shiroishi, Citation1962), C. restuans (Beier & Trpis, Citation1981) or C. (Culex) sp. (Bueno et al., Citation2010). In the natural environment, C. quinquefasciatus has been implicated in the transmission of Plasmodium spp. to penguins in South Africa (Fantham & Porter, Citation1944), New Zealand (Tompkins & Gleeson, Citation2006) and Galápagos Islands (Levin et al., Citation2009).
Life cycle
The general features of the life cycle of Plasmodiidae species presented below are based on what is known about the subgenus Haemamoeba, given that the development in these species is the most well studied (Valkiūnas, Citation2005). Because penguins have dense plumage, most vectors feed on exposed skin such as the areas surrounding the eyes, legs, feet and beak (Atkinson, Citation1999; Vanstreels & Parsons, Citation2014).
When the female mosquitoes feed on the blood of a penguin, the saliva that is injected into the host's tissues contains sporozoites that proceed to invade reticuloendothelial cells (macrophages, monocytes and endothelial cells) near the injection site and develop into the first generation of exoerythrocytic meronts (cryptozoites) () (Valkiūnas, Citation2005; Huijben et al., Citation2007). These meronts will undergo asexual multiplication to form numerous smaller mononuclear structures, the merozoites. Upon rupture of the host cell, merozoites are released and can spread through the blood stream, in order to continue to subsequent life cycle stages (Valkiūnas, Citation2005; Atkinson, Citation2008).
Figure 1. Life cycle of avian malaria parasites (using P. relictum as an example). I – primary (pre-erythrocytic) exoerythrocytic merogony; II – erythrocytic merogony; III – secondary (posterythrocytic) exoerythrocytic merogony; 1 – sporozoite invading a reticuloendothelial cell; 2 and 3 – cryptozoite; 4 – merozoite invading a reticuloendothelial cell; 5 – metacryptozoite; 6 – merozoite invading an erythrocyte; 7 and 8 – erythrocytic meront; 9 – merozoite invading an endothelial cell; 10 and 11 – phanerozoite; 12 – macrogametocyte and microgametocyte; 13 – macrogamete; 14 – exoflagellation of microgametes; 15 – zygote; 16 – ookinete; 17 and 18 – oocyst; 19 – sporozoites. © Diogo Guerra 2015.
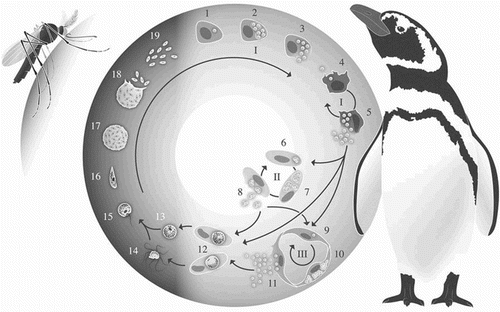
The development of the subsequent generations of merogony (metacryptozoites and phanerozoites) can occur in reticuloendothelial cells in a variety of host tissues. Exoerythrocytic meronts are most frequently detected in the lungs, liver, spleen, kidney and brain. In the species belonging to the subgenus Huffia, exoerythrocytic merogony also occurs in haematopoietic cells, particularly in the bone marrow (Valkiūnas, Citation2005; Atkinson, Citation2008).
Several cycles of merogony may follow, greatly increasing the parasite population within the avian host. Merozoites can either invade reticuloendothelial cells and undergo new cycles of exoerythrocytic merogony, or they can invade erythrocytes and proceed to erythrocytic merogony or gametogony (sexual stages) (Garnham, Citation1966; Valkiūnas, Citation2005). After invasion of erythrocytes, the merozoites develop into trophozoites, which then grow to become erythrocytic meronts; alternatively, some of the merozoites develop into gametocytes. The morphology of erythrocytic meronts and gametocytes varies among different species of Plasmodium, and is therefore used to identify and classify these parasites. When infecting erythrocytes, Plasmodium spp. (as well as Haemoproteus spp.) will form haemozoin granules (malarial pigment), a by-product from the incomplete digestion of the host cell haemoglobin. Most Plasmodium spp. will infect only mature erythrocytes, however, the meronts of the species in the Huffia subgenus can also develop in younger erythrocytic lineage cells (erythroblasts and polychromatophilic erythrocytes). It is relevant to note that the morphologically similar Haemoproteus spp. do not form erythrocytic meronts, and therefore this is a key characteristic to differentiate them from Plasmodium spp. (Valkiūnas, Citation2005; Atkinson, Citation2008).
Gametogony is the final stage of the parasite's life cycle in the avian host. When merozoites develop into gametocytes, they develop into either macrogametocytes or microgametocytes. The first has a more intensely stained cytoplasm and a more compact nucleus than the latter. These stages remain inside erythrocytes and do not continue their development until being ingested by a mosquito (Valkiūnas, Citation2005; Huijben et al., Citation2007; Atkinson, Citation2008). After the invertebrate host feeds each macrogametocyte will form one macrogamete, and each microgametocyte will undergo exflagellation and form eight microgametes. Fertilization occurs in the mosquito midgut, and an ookinete is formed within 16–48 h. The ookinete is mobile and proceeds to the epithelial layer of the midgut, where it becomes installed. Under the basal lamina, it rounds up and develops into an oocyst. During the oocyst development, sporogony takes place and the sporozoites are formed. When mature, they are released into the mosquito's haemocoele and then migrate to the salivary glands. They will be inoculated with the saliva into the avian host during the mosquito's next blood meal (Langer & Vinetz, Citation2001; Valkiūnas, Citation2005; Huijben et al., Citation2007).
Epidemiology
Avian malaria is a markedly seasonal disease, dependent upon the availability of a vector population (Graczyk et al., Citation1994c; Braga et al., Citation2011). In penguins kept in captivity in outdoor enclosures, mortality is usually reported during summer or early autumn outbreaks, with most cases concentrated from June to October in the Northern Hemisphere (Graczyk et al., Citation1994d; Huijben et al., Citation2007; Chitty et al., Citation2015) and from October to March in the Southern Hemisphere (Parsons & Underhill, Citation2005; Vanstreels et al., Citation2015).
Naïve individuals, such as chicks, juvenile birds and adults that have not had previous exposure to mosquitoes (such as recently wild-caught birds or those raised in an arthropod-free environment), show the highest levels of susceptibility (Beier & Stoskopf, 1980; Dinhopl et al., Citation2011; Wallace, Citation2014). The most severe presentation of disease occurs after primary exposure. The infection occurs very rapidly after penguins are placed in outdoor exhibits and mortality can be high (Graczyk et al., Citation1994a). Subsequent exposures to the parasite are usually not fatal (Graczyk et al., Citation1994d) and surviving birds will develop what appears to be protective immunity that controls endothelial parasite stages, while developing low-level parasitaemia with no clinical signs (Graczyk et al., Citation1994c, Citation1995a). This is called premunition, and consists of antibody and cell-mediated responses to a low-level chronic parasite infection. The bird is not capable of clearing the Plasmodium spp. infection, which results the host's immune system to be constantly stimulated. When re-infected with homologous strains of Plasmodium spp., they will only have short and low intensity parasitaemia, without mortality (Stoskopf & Beier, Citation1979; Valkiunas, Citation2005; Atkinson, Citation2008).
Specific anti-Plasmodium spp. immunoglobulins are passed as maternal antibodies through the egg yolk and are detectable for up to eight weeks post-hatch (Graczyk et al., Citation1994a; Palmer et al., Citation2013). Captive-born chicks are usually maintained indoors for intensive care during the first months of their lives and when they are first transferred to the outdoor exhibit they will have lost the maternal antibody protection, being naïve to Plasmodium spp. (Graczyk et al., Citation1994b).
Native wild bird species, particularly passerines, are known to be frequently infected with Plasmodium spp. (Bennett et al., Citation1993a; Dufva, Citation1996; Quillfeldt et al., Citation2011). These birds share habitats with captive penguins and act as reservoirs for the parasite (Beier & Trpis, Citation1981; Bueno et al., Citation2010; Dinhopl et al., Citation2011, Citation2015). As a result, the Plasmodium spp. lineages infecting penguins at a given collection will reflect those that are present in the regional avifauna (Vanstreels et al., Citation2015). In their study, Beier and Stoskopf (Citation1980) hypothesized that because infected African penguins rarely exhibited circulating parasites, these birds probably do not participate in the transmission of avian malaria; thus, Plasmodium spp. infections in penguins probably only occur when the cycle of transmission is sustained by other avian species that act as competent reservoirs.
Records show that avian malaria outbreaks in captive penguins can occur throughout the warmer months of the year; however, they often concentrate over a relatively short period of time (often two to three weeks) during which most of the mortality occurs, and the cumulative mortality can be as high as 50–80% (Rodhain, Citation1939; Griner & Sheridan, Citation1967; Fleischman et al., Citation1968; Stoskopf & Beier, Citation1979; Fix et al., Citation1988; Graczyk et al., Citation1994c, Citation1994d; Huijben et al., Citation2007; Bueno et al., Citation2010; Vanstreels et al., Citation2014). Among African penguins that survive the first infection, however, the probability of mortality decreases to 3–4% (Cranfield, Citation2003).
Clinical signs
The clinical manifestations of malaria infection may not be evident during outbreaks in captive penguins, and it is common to find dead birds without previous sign of disease (Stoskopf & Beier, Citation1979; Wallace, Citation2014). Typical signs can include loss of appetite, weight loss, respiratory distress, lethargy, weakness, pale mucous membranes, isolation from the group, vomiting, regurgitation following force-feeding and greenish faeces (Rodhain, Citation1939; Griner & Sheridan, Citation1967; Fleischman et al., Citation1968; Fix et al., Citation1988; Grim et al., Citation2003; Bueno et al., Citation2010; AZA Penguin Taxon Advisory Group, Citation2014; Campos et al., Citation2014). Severe forms of the disease have been described to induce neurological signs, including motor incoordination, convulsions and paralysis (Cranfield, Citation2003; Grim et al., Citation2003), usually in a terminal state (Valkiūnas, Citation2005).
These clinical signs are non-specific and can also be present in other common diseases reported in penguins. Diseases that can occur alone or concurrently with avian malaria include aspergillosis, West Nile Virus infection, poxviruses, helminthiasis, Chlamydophila psittaci infection and bacterial gastroenteritis involving Clostridium perfringens (Rodhain, Citation1939; Griner & Sheridan, Citation1967; Fleischman et al., Citation1968; Penrith et al., Citation1994; Graczyk & Cranfield, Citation1996; Jencek et al., Citation2012; Wallace, Citation2014; Vanstreels et al., Citation2015). As a result, clinical signs are not reliable for the diagnosis of avian malaria in penguins.
Lesions
Lesions associated with acute infection include hepatomegaly, splenomegaly and pulmonary oedema. Liver and spleen may present a dark colour due to the accumulation of malarial pigment in macrophages (Rodhain, Citation1939; Griner & Sheridan, Citation1967; Fix et al., Citation1988; Atkinson, Citation2008). Cardiomegaly, pericardial effusion and nephromegaly have also been documented in some cases (Fleischman et al., Citation1968; Fix et al., Citation1988; Grim et al., Citation2003).
Histopathological examination often reveals tissue meronts in endothelial cells and tissue macrophages, especially in the spleen, lungs, liver and heart (Rodhain, Citation1939; Fleischman et al., Citation1968; Fix et al., Citation1988; Cranfield, Citation2003; Dinhopl et al., Citation2011; Vanstreels et al., Citation2015). It is important to note that, unlike mammal-infecting Plasmodium spp. and avian-infecting Leucocytozoon spp., there is no invasion of hepatocytes in avian-infecting Plasmodium spp. (Valkiūnas, Citation2005).
Infiltration of leukocytes is observed in various tissues. Typical findings include diffuse granulocytic pneumonia, multifocal mononuclear hepatitis, diffuse hepatic haemosiderosis, diffuse granulocytic splenitis and splenic haemorrhages and rupture. Extramedullary haematopoiesis may also occur in some cases (Rodhain, Citation1939; Fleischman et al., Citation1968; Fix et al., Citation1988; Silveira et al., Citation2013; Vanstreels et al., Citation2015).
These lesions are thought to result primarily from vascular occlusion and rupture due to the development of tissue meronts in the endothelial cells. As a result, hypoxia/anoxia, apoptosis and necrosis develop in various tissues. Additionally, erythrocytic infection by parasites may produce direct haemolysis or induce erythrocyte sequestration and extravascular haemolysis (Atkinson & Van Riper, Citation1991; Valkiūnas, Citation2005; Williams, Citation2005; Marzal, Citation2012). Some authors suggest that because penguins have low-level parasitaemia, the destruction of erythrocytes is not sufficient to cause clinical anaemia (Cranfield et al., Citation1994; Dinhopl et al., Citation2011).
In some cases death can result from brain damage associated with the exoerythrocytic merogony in endothelial cells of the capillaries irrigating the brain (Valkiūnas, Citation2005; Marzal, Citation2012). In other cases, death is thought to result from respiratory insufficiency from the marked pneumonia and lung oedema (Fix et al., Citation1988; Vanstreels et al., Citation2015) or from circulatory shock due to cardiac tamponade by pericardial effusion (Vanstreels & Parsons, Citation2014).
Diagnosis
Diagnosis is one of the most important steps to control outbreaks of avian malaria in captive penguins.
Giemsa-stained thin blood smear examination is still considered the gold standard test in avian malaria diagnosis, being extremely accurate when erythrocytic parasites are detectable in red blood cells (erythroblasts or erythrocytes) (Atkinson et al., Citation2001; Cranfield, Citation2003; Valkiūnas, Citation2005). It should be noted, however, that some of the life stages of other blood parasites known to occur in penguins such as Babesia spp. (Earlé et al., Citation1993), Haemoproteus spp. (Levin et al., Citation2009) and Leucocytozoon spp. (Fallis et al., Citation1976) may closely resemble those of Plasmodium spp. The conclusive diagnosis of avian malaria through blood smears therefore requires the observation of erythrocytic meronts, which are unique to Plasmodium spp. (Valkiūnas, Citation2005) (). Importantly, blood smears prepared from capillary blood seem to increase the sensitivity of the test when compared to those from venous blood (Njunda et al., Citation2013).
Figure 2. Schematic representation of erythrocytic life stages of P. relictum (top line) and P. elongatum (bottom line). 1 and 5 – trophozoites, 2 and 6 – erythrocytic meronts, 3 and 7 – macrogametocytes, 4 and 8 – microgametocytes. Adapted from Valkiūnas (Citation2005). Morphological descriptions and illustrations of other Plasmodium species known to infect penguins can be found in Valkiūnas (Citation2005) (P. cathemerium, P. juxtanucleare, P. nucleophilum and P. tejerai) and Mantilla et al. (Citation2013) (P. unalis).
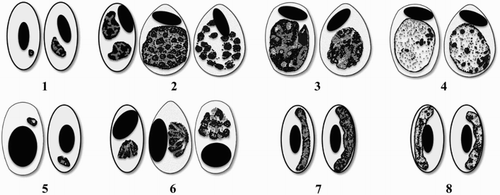
Microscopic examination of blood films is an inexpensive technique that provides an estimate of the parasitaemia intensity (Waldenström et al., Citation2004), although for penguins this may be a poor predictor of survival, as it is common to have lethal cases in penguins with low parasitaemia (Cranfield et al., Citation1990). The sensitivity of this method can be low and blood smears observation may not be useful for diagnosis (Cranfield et al., Citation1990). It is possible to see acute infections causing mortality without parasitaemia being present. In several cases, confirmation of the disease must be achieved through histopathology or molecular methods (Cranfield et al., Citation1990; Dinhopl et al., Citation2011; Vanstreels et al., Citation2014, Citation2015). Furthermore, parasites may remain undetectable in the blood stream during chronic infections and detection by microscopy is difficult. Therefore, negative blood smears cannot be considered sufficient to rule out infection (Jarvi et al., Citation2002). Lastly, a qualified and experienced professional is essential, since identification of Plasmodium spp. can be difficult and time consuming (Beier & Stoskopf, Citation1980; Zehtindjiev et al., Citation2008).
Some haematological values have been suggested to be useful markers of malaria infection. Penguins infected with malaria can present with a moderate to severe anaemia, total white blood cell counts greater than 20 × 103/µl and a relative lymphocytosis higher than 60% (Stoskopf & Beier, Citation1979; Cranfield, Citation2003; Wallace, Citation2014). These haematological differences, however, may not be sufficiently consistent or specific to be used as diagnostic criteria for avian malaria (Graczyk et al., Citation1994d; Cranfield, Citation2003). Graczyk et al. (Citation1995c) found that gamma-glutamyltranspeptidase, alanine aminotransferase and creatinine may be useful for monitoring the course of disease and overall prognosis in African penguins infected with P. relictum during pre-erythrocytic development stages.
Polymerase chain reaction (PCR)-based methods have significantly higher sensitivity than light microscopy, but may still fail to identify low-level parasitaemia or mixed infections (Richard et al., Citation2002; Valkiūnas et al., Citation2006; Krams et al., Citation2012). Different protocols for PCR and nested PCR have been developed, targeting either 18S ribosomal subunit chromosomal gene (18S rRNA) or the cytochrome b mitochondrial gene (cyt-b). There is debate on the performance and limitations of these methods (Richard et al., Citation2002; Freed & Cann, Citation2006; Valkiūnas et al., Citation2006), however, the cyt-b protocols developed by Waldenström et al. (Citation2004) and Hellgren et al. (Citation2004) have gradually become the most widely used in the past decade, in part due to the establishment of an international peer-reviewed database of sequences for phylogenetic analyses and molecular detection of avian malaria parasites (Bensch et al., Citation2009). Drawbacks include the high cost, time consumed and the fact that the existing protocols are not able to selectively amplify Plasmodium. Furthermore, PCR testing implies the subsequent need of additional gene sequencing procedures to differentiate between Haemoproteus spp., Leucocytozoon spp. and Plasmodium spp. (Cosgrove et al., Citation2006; Szöllsi et al., Citation2008) and to check variations in parasite sequences that inhibit specific alignment of primers (Richard et al., Citation2002). False negatives may occur due to insufficient concentration of parasite DNA or inadequate DNA extraction from the sample (Richard et al., Citation2002). There is also evidence that the performance of PCR-based methods may vary significantly among Plasmodium species and lineages (Valkiūnas et al., Citation2006), and some species may evade PCR detection altogether (Zehtindjiev et al., Citation2012). Because these techniques and blood smear analysis are both likely to underestimate avian malaria infection, the two methods should be considered complementary (Valkiūnas et al., Citation2006; Braga et al., Citation2011; Okanga et al., Citation2013). Furthermore, it is worth noting that PCR-positive results may be produced even when the parasite fails to develop a complete life cycle in a host; in these cases of abortive development, the positive results occur due to the amplification of DNA from sporozoites or remnants of tissue meronts (Levin et al., Citation2013; Valkiūnas et al., Citation2014).
Post-mortem diagnosis is performed by examining the coelom for hepatomegaly and splenomegaly. Other findings may include hydropericardium and lung congestion (Cranfield, Citation2003). The preparation of kidney, liver, spleen and/or lung impression smears during necropsy may also assist the diagnosis (Fix et al., Citation1988; Cranfield, Citation2003; Vanstreels & Parsons, Citation2014). In fact, some pathologists prefer the use of impression smears of cut organ surfaces over histopathology for diagnosis of avian malaria as enabling better identification of organisms in erythrocytes (St. Leger, 1 July, personal communication, 2014).
Histopathology is another classical method to diagnose avian malaria in deceased penguins, through the visualization of tissue meronts within macrophages and endothelial cells (Rodhain, Citation1939; Fleischman et al., Citation1968). Care should be taken not to mistake Plasmodium spp. tissue meronts with other protozoan cysts such as tachyzoites of Toxoplasma spp. (Ploeg et al., Citation2011), bacterial colonies or fragmented nuclei of necrotic tissues. Dinhopl et al. (Citation2011) developed a chromogenic in-situ hybridization test with a digoxigenin-labelled probe, which targets a fragment of the 18S ribosomal subunit RNA of Plasmodium spp. using paraffin wax-embedded tissues. Using tissue samples from Humboldt, Southern rockhopper (Eudyptes chrysocome) and King penguins (Aptenodytes patagonicus), Plasmodium spp. meronts were identified by a purple to black signal within the capillary endothelium. With this method, mistaking fragmented nuclei within necrotic tissue with meronts does not occur, as it may in histopathology.
Graczyk et al. (Citation1994c) developed an enzyme-linked immunosorbent assay (ELISA) to detect anti-P. relictum and anti-P. elongatum antibodies from infected African penguins using P. falciparum antigens. This technique is simple (based on collection of blood or egg-yolk samples on filter paper), sensitive, rapid and relatively inexpensive (Graczyk et al., Citation1995b, d; Graczyk & Cranfield, Citation1996; Atkinson & Paxton, Citation2013). Also, there is the possibility of testing against several antigens at the same time (Graczyk et al., Citation1994c), detecting exposure to the parasite and measuring the response to vaccination (Cranfield, Graczyk & McCutchan, Citation2000). On the other hand, some authors have suggested that this method may produce false-positive results and therefore could have limited specificity (Sturrock & Tompkins, Citation2007; McDonald, Citation2012). Additionally, it is important to note that antibody levels measured by ELISA do not correlate with parasitaemia, as birds may have antibodies from previous exposure to the pathogen, and will maintain the antibodies even if the infection is cleared. Additionally, this technique is not useful to determine when treatment is needed or when it can be discontinued (Cranfield et al., Citation2000).
Rapid diagnostic tests identifying Plasmodium lactate dehydrogenase have been used in African penguin with variable results (Killick et al., Citation2008; Parsons, 10 December, personal communication, 2012). At present, the validity of this method for these species is questionable.
Treatment
The effectiveness of medical treatment usually decreases greatly by the time the first clinical signs appear (Cranfield, Citation2003). Positions on whether constant or seasonal treatment should be used vary with different institutions, as some prefer to avoid mortality associated with malaria and others prefer not to expose their colonies to negative side effects of antimalarial drugs. Unnecessary or early treatment may interfere with the acquisition of natural immunity, leaving penguins more susceptible in the following seasons (Cranfield, Citation2003). When administered at the right time, antimalarial drugs () can assist in suppressing the parasitaemia, while maintaining a stimulus for the development of immunity (Graczyk et al., Citation1994d). Cranfield et al. (Citation2000) stated that a routine of weekly examination of blood smears and treatment with primaquine and chloroquine when penguins were parasitaemic reduced the experienced mortality from 50% to 10–15%.
Table 1. Examples of antimalarials used in penguin colonies, their parasite stage's target and mechanism of action.
Treatment protocols described for malaria infections on captive penguins vary in posology, but overall, associations of primaquine and chloroquine have been the most popular choices. More recently, zoos have experimented with other drugs such as mefloquine or the combination of atovaquone and proguanil; these have been shown to be effective for malaria treatment in other birds, even if exoerythrocytic stages are unaffected (Remple, Citation2004; Palinauskas et al., Citation2009). A summary of protocols described in the literature is presented in ; it should be noted, however, that the absence of studies on the pharmacokinetics and pharmacodynamics of these drugs in penguins implies that all dosing is based on empirical evidence.
Table 2. Examples of treatment and prophylactic protocols described in the literature specifically designed for penguins.
Perhaps the most important side effect from administrating prophylactic drugs is the stress due to the frequent handling of penguins, possibly interfering with breeding success and appetite (Chitty, Citation2011). In addition, moulting and brooding birds will often enter a period of anorexia that will make prophylactic dosing difficult without handling or stressing birds (AZA Penguin Taxon Advisory Group, Citation2014). Furthermore, side effects from treatment with antimalarial drugs must be considered. Pyrimethamine is a folic acid inhibitor and a known teratogen, hence caution must be taken when used in laying females during the reproductive season; Tollini et al. (Citation2000) recommend the discontinuation of pyrimethamine treatment 10 days before breeding/laying season. Oral supplementation with folic acid may be given when birds are on prophylactic regimen (AZA Penguin Taxon Advisory Group, Citation2014). Sulfadiazine has been shown to cause diarrhoea in penguins (Tollini et al., Citation2000). Regurgitation has been noticed in African penguins when treated for several consecutive days on a mefloquine prophylactic treatment (Gyimesi, 9 August, personal communication, 2015). Wünschmann et al. (Citation2006) described a neuronal storage disease in Humboldt penguins treated prophylactically with chloroquine during the mosquito season (June until end of October or death). Chloroquine has been shown to cause the same effects in rats and miniature pigs in experimental conditions (Klinghardt et al., Citation1981; Dietzmann et al., Citation1985), hence caution is advised when employing high and long-term treatment with this drug.
Palliative care can also be considered to manage clinical signs and secondary effects of the infection. For severely anaemic penguins, blood transfusion may be an option when haematocrit rapidly decreases to less than 20% and/or is not stable. In these cases, blood transfusion appears to shorten the convalescent period until antimalarial drugs start to take effect. When haematocrit is stable, blood transfusion is not necessary as penguins usually have a good erythropoiesis regenerative response. In this case, supportive care alone is the best option (fluids, iron and complex B vitamins supplementation, and oxygen therapy) (AZA Penguin Taxon Advisory Group, Citation2014).
Bueno et al. (Citation2010) described the use of injectable aminophylline and hydrocortisone to diminish respiratory symptoms in Magellanic penguins infected with P. relictum. Tollini et al. (Citation2000) suggest the administration of fluconazole (100 mg SID) to prevent opportunistic aspergillosis.
Even though isolation of infected birds may facilitate the treatment process, penguins are sociable birds, and thus housing with companion birds or the mate is recommended. If this is not possible, mates should be within visual or vocal range of the isolated penguins (AZA Penguin Taxon Advisory Group, Citation2014).
Prophylaxis
Prophylaxis is the key to managing malarial infection in captive penguins (Cranfield, Citation2003; Valkiūnas, Citation2005). Prevention of this disease can be achieved through three approaches: (1) reducing or eliminating mosquitoes, as well as employing physical barriers to protect penguins from being exposed to them, (2) using drug prophylaxis to prevent the development and/or reduce the clinical severity of the infection, and (3) allowing the penguin's immune system to respond to the infection (Cranfield et al., Citation2000; Cranfield, Citation2003; Vanstreels & Parsons, Citation2014).
Zoological gardens and rehabilitation centres use different approaches when dealing with malaria in their penguin colonies. The most straightforward strategy to prevent malarial infection is to maintain penguins in mosquito-free indoor facilities year-round (Graczyk et al., Citation1994d). Using pesticide strips and covering zoo exhibits and rehabilitation centre's facilities with fine-mesh bolting silk or other mosquito netting is effective in controlling the presence of mosquitoes (Beier & Stoskopf, Citation1980; Valkiūnas, Citation2005). Penguins may also be kept in indoor facilities during mosquito season or during the hours of high vector activity (Valkiūnas, Citation2005; AZA Penguin Taxon Advisory Group, Citation2014). Beier and Stoskopf (Citation1980) determined that feeding periodicity for the vectors peaked between midnight and 2 am, as diurnal contact is not likely to happen. Additionally, observations showed that juvenile penguins spend a great amount of time outdoors at night compared with adults, enhancing vector exposure.
When this is not possible, other measures may help reduce exposure to vectors. The usage of fans to circulate air and create wind currents in the outdoor exhibits may help to control vector infestation (AZA Penguin Taxon Advisory Group, Citation2014). Setting up mosquito traps may also be a good option (Valkiūnas, Citation2005). Since some plants have mosquito repellent properties due to their essential oils (Choi et al., Citation2002), use of spray repellent products in the nest boxes and having mosquito repellent plants (e.g. lavender, lemongrass) may deter vectors from coming near the penguin exhibit.
Monitoring mosquito larvae density in ponds in and around the zoo may help to evaluate the probability of infection (Bureau of Medicine and Surgery, Citation2000). Draining and cleaning these water bodies or using pumps to keep water moving helps to reduce mosquito larvae habitat and it may reduce the incidence of avian malaria in nearby captive penguins (Cereghetti et al., Citation2012; Grilo, Citation2014). The bacterium Bacillus thuringiensis israelensis is environmental-friendly and considered a highly effective method for controlling larvae of many mosquito species (Stockklausner et al., Citation2013). However, some authors refer a low to moderate level of mosquito resistance in areas where these bacteria have been used intensively (e.g. crop protection) (Paris et al., Citation2011; Tetreau et al., Citation2013). Other disadvantages are presented, such as the possibility of the bacteria sinking to the bottom of the pond, adsorption onto organic matter, inactivation by sunlight and ingestion by organisms to which it is not toxic (Ben-Dov, Citation2014). Another possibility is the use of larvae-eating fish in the ponds, such as the Fathead Minnow (Pimephales promelas) or the Mosquito fish (Gambusia sp.) (Irwin & Paskewitz, Citation2009; Thurber et al., Citation2014).
Preventative drug treatment protocols have been widely used by zoos, and usually rely on the daily to weekly administration of primaquine (see ) (Grilo, Citation2014). Such treatments do not prevent the emergence of avian malaria, however they are known to reduce the severity of outbreaks should they occur. When drug prophylaxis is routinely employed, it usually is done every year, since it may prevent some individuals from developing natural immunity against Plasmodium spp. (Cranfield, Citation2003).
If penguins are allowed to acquire natural immunity, close monitoring to rapidly initiate treatment is required (Graczyk et al., Citation1994d). Even when immunity is established, testing for malaria is still essential to control for possible relapses (Cranfield, Citation2003; AZA Penguin Taxon Advisory Group, Citation2014).
Cranfield et al. (Citation2000) developed an experimental vaccine using DNA sequences of the circumsporozoite gene of P. relictum and P. elongatum for use in penguins. Naïve African penguins housed indoors were vaccinated intradermally above the eyes and intramuscularly in the gastrocnemius muscle. Booster injections were given three to four weeks later and then penguins were allowed to go the outside exhibit. Antibody levels increased after vaccination and there was a 75% reduction in mortality. In another study, Grim et al. (Citation2004) reported that parasitaemia rates (P. relictum) in vaccinated African penguins decreased from 50% to 17% despite intense mosquito infection rate, with no mortalities or side effects recorded in the vaccination year. Even though birds are still stimulated by natural infection after immunization, the long-term immunity was low and vaccination had to be repeated every summer. In a study using the vaccine on canaries, McCutchan et al. (Citation2004) found that vaccination produced a statistically significant decrease in mortality due to avian malaria in canaries. However, two seasons after the vaccination, this difference no longer existed. Also, the mortality recorded the in second year occurred in vaccinated birds, while the ones with natural immunity survived. The hypothesis that the vaccine eliminates or significantly reduces the parasite load and therefore inhibits acquisition of natural immunity seems to be the explanation. As such, boosters must be given every season (Cranfield et al., Citation2000; Cranfield, Citation2003).
There is evidence of genetic resistance to infection, particularly in birds that do not succumb to initial infection and become chronically infected. (USGS, Citation2006). Graczyk et al. (Citation1994b) proposed that females that produce high titres of anti-Plasmodium spp. antibodies should be used preferentially for reproduction and that the serological profiles of each individual should be part of breeding programmes of outdoor colonies.
Conclusion and future directions
Avian malaria has been shown to profoundly impact many of the captive penguin colonies worldwide. Fortunately, certain measures can be taken to reduce or eliminate the effects of this disease in highly susceptible species, including penguins.
In the future, better knowledge of the correlation of parasitological variables with environmental and housing conditions, demographics of penguin populations, health status of colonies regarding other common diseases and treatment and supportive care protocols associated with the development of the disease would be valuable insights with practical implications for the prevention and treatment of avian malaria in captive penguins. Pharmacokinetic studies will be fundamental to provide objective criteria regarding posology for antimalarial treatment protocols in penguins. Also, avian malaria prevalence studies in nearby captive and free-ranging birds may assist in identifying which species can serve as reservoirs of the disease. Similarly, prevalence studies of mosquito vectors and any harboured Plasmodium species are required. Development and testing of novel inexpensive and rapid diagnostic methods will increase the possibility of an accurate diagnosis at early stages, improving the effectiveness of medical treatment.
Almost 90 years after Scott (Citation1927) first reported avian malaria in a king penguin, this disease remains an important cause of mortality in captive penguins. Climate changes and human activity may be affecting the impact of this disease over certain wild and endangered penguin populations (Levin et al., Citation2009; Palmer et al., Citation2013). Climate change is predicted to increase malarial infection risk in wild birds (Garamszegi, Citation2011).
It is the ethical responsibility and in the direct interest of zoological gardens and rehabilitation centres to implement measures to prevent and mitigate the impacts of avian malaria in their penguin collections, but also to be committed in conducting and supporting scientific research towards a better understanding of the disease, and especially to help in designing intervention and control strategies to mitigate the potential impact of avian malaria in wild populations at risk.
Acknowledgements
The authors wish to thank Dr Diogo Guerra for the malaria life cycle illustration. M.L.G. and L.M.d.C. are thankful to Interdisciplinary Centre of Research in Animal Health CIISA-FMV-ULisboa. JLCD and RETV are thankful to São Paulo Research Foundation (FAPESP 2009/53956-9, 2010/51801-5), Brazilian Federal Agency for the Support and Evaluation of Graduate Education (CAPES) and National Counsel of Technological and Scientific Development (CNPq). This work was performed under the frame of EurNegVec COST Action TD1303.
Disclosure statement
No potential conflict of interest was reported by the authors.
Additional information
Funding
References
- Atkinson, C.T. (1999). Hemosporidiosis. In M. Friend & J.C. Franson (Eds.), Field Manual of Wildlife Diseases (pp. 193–194). Madison, WI: U.S. Geological Survey.
- Atkinson, C.T. (2008). Avian malaria. In C.T. Atkinson, N.J. Thomas & D.B. Hunter (Eds.), Parasitic Diseases of Wild Birds (pp. 35–53). Ames, IA: Wiley-Blackwell.
- Atkinson, C.T., Dusek, R.J. & Lease, J.K. (2001). Serological response and immunity to superinfection with avian malaria in experimentally-infected Hawaii amakihi. Journal of Wildlife Diseases, 37, 20–27. doi: 10.7589/0090-3558-37.1.20
- Atkinson, C.T., Dusek, R.J., Woods, K.L. & Iko, W.M. (2000). Pathogenicity of avian malaria in experimentally-infected Hawaii amakihi. Journal of Wildlife Diseases, 36, 197–201. doi: 10.7589/0090-3558-36.2.197
- Atkinson, C.T. & LaPointe, D.A. (2009). Introduced avian diseases, climate change, and the future of Hawaiian honeycreepers. Journal of Avian Medicine and Surgery, 23, 53–63. doi: 10.1647/2008-059.1
- Atkinson, C.T. & Paxton, E.H. (2013). Immunological Markers for Tolerance to Avian Malaria in Hawaìi Amakihi: New Tools for Restoring Native Hawaiian Forest Birds? (Technical Report HCSU-042).
- Atkinson, C.T. & Van Riper, C. (1991). Pathogenicity and epizootiology of avian haematozoa: Plasmodium, Leucocytozoon and Haemoproteus. In J.L. Loye & M. Zuk (Eds.), Bird-Parasite Interactions. Ecology, Evolution and Behavior (pp. 19–48). New York, NY: Oxford University Press.
- AZA Penguin Taxon Advisory Group. (2014). Penguin (Spheniscidae) Care Manual. Silver Spring, MD: Association of Zoos and Aquariums.
- Bak, U., Park, J. & Lim, Y. (1984). An outbreak of malaria in penguins at the farmland Zoo. Korean Journal of Parasitology, 22, 267–272. doi: 10.3347/kjp.1984.22.2.267
- Basilico, N., Spaccapelo, R. & D'Alessandro, S. (2015). Malaria diagnosis, therapy, vaccines, and vector control. In M. Prato (Ed.), Human and Mosquito Lysozymes: Old Molecules for New Approaches Against Malaria (pp. 19–43). Cham, Switzerland: Springer International.
- Beier, J.C. & Stoskopf, M.K. (1980). The epidemiology of avian malaria in black-footed penguins (Spheniscus demersus). The Journal of Zoo Animal Medicine, 11, 99–105. doi: 10.2307/20094486
- Beier, J.C. & Trpis, M. (1981). Incrimination of natural culicine vectors which transmit Plasmodium elongatum to penguins at the Baltimore Zoo. Canadian Journal of Zoology, 59, 470–475. doi: 10.1139/z81-068
- Ben-Dov, E. (2014). Bacillus thuringiensis subsp. israelensis and its dipteran-specific toxins. Toxins, 6, 1222–1243. doi: 10.3390/toxins6041222
- Bennett, G.F., Bishop, M.A. & Peirce, M.A. (1993a). Checklist of the avian species of Plasmodium Marchiafava & Celli, 1885 (Apicomplexa) and their distribution by avian family and Wallacean life zones. Systemic Parasitology, 26, 171–179. doi: 10.1007/BF00009724
- Bennett, G.F., Peirce, M.A. & Ashford, R.W. (1993b). Avian haematozoa: mortality and pathogenicity. Journal of Natural History, 27, 993–1001. doi: 10.1080/00222939300770621
- Bensch, S., Hellgren, O. & Pérez-Tris, J. (2009). MalAvi: a public database of malaria parasites and related haemosporidians in avian hosts based on mitochondrial cytochrome b lineages. Molecular Ecology Resources, 9, 1353–1358. doi: 10.1111/j.1755-0998.2009.02692.x
- Braga, E.M., Silveira, P., Belo, N.O. & Valkiūnas, G. (2011). Recent advances in the study of avian malaria: an overview with an emphasis on the distribution of Plasmodium spp. in Brazil. Memórias do Instituto Oswaldo Cruz, 106, 3–11.
- Brossy, J.J. (1992). Malaria in wild and captive Jackass Penguins Spheniscus demersus along the southern African coast. Ostrich, 63, 10–12. doi: 10.1080/00306525.1992.9634174
- Brossy, J.J., Plös, A.L., Blackbeard, J.M. & Kline, A. (1999). Diseases acquired by captive penguins: what happens when they are released into the wild? Marine Ornithology, 27, 185–186.
- Bueno, M.G., Lopez, R.P.G., Menezes, R.M.T., Costa-Nascimento, M.J., Lima, G.F.M.C., Araújo, R.A.S., Guida, F.J.V. & Kirchgatter, K. (2010). Identification of Plasmodium relictum causing mortality in penguins (Spheniscus magellanicus) from São Paulo Zoo, Brazil. Veterinary Parasitology, 173, 123–127. doi: 10.1016/j.vetpar.2010.06.026
- Bureau of Medicine and Surgery. (2000). Unit Protective Measures. Navy Medical Department Pocket Guide to Malaria Prevention and Control.
- Campos, S.D.E., Pires, J.R., Nascimento, C.L., Dutra, G., Torres-Filho, R.A., Toma, H.K., Brener, B. & Almosny, N.R.P. (2014). Analysis of hematologic and serum chemistry values of Spheniscus magellanicus with molecular detection of avian malarial parasites (Plasmodium spp.). Pesquisa Veterinária Brasileira, 34, 1236–1242. doi: 10.1590/S0100-736X2014001200016
- Cereghetti, N., Wenker, C., Hoby, S., Müller, P., Marti, H. & Lengeler, C. (2012). Avian malaria and 1st prevention strategies in the Zoo Basel. In F. Chappuis, C. Lengeler & N. Müller (Eds.), Posters SSTMP Joint Annual Meeting (p. 2). St. Gallen, Switzerland.
- Chitty, J. (2011). Use of doxycycline in treatment of malaria in penguins. In E. Bergman (Ed.), Proceedings of the Association of Avian Veterinarians 32nd Annual Conference & Expo with the Association of Exotic Mammal Veterinarians (pp. 63–65). Seattle, WA.
- Chitty, J., Barrows, M., Feltrer, Y., Flach, E., Grilo, M., Justice, W., Sayers, G., Thornton, S. & Whitehead, M. (2015). A review of avian malaria in penguins in UK Zoos. In N. Chai, M. Huynh, C. Pignon & L. Schilliger (Eds.), Proceedings of the 2nd International Conference on Avian Herpetological and Exotic Mammal Medicine (ICARE) (p. 263). Paris.
- Choi, W., Park, B., Ku, S. & Lee, S. (2002). Repellent activities of essential oils and monoterpenes against Culex pipiens pallens. Journal of the American Mosquito Control Association, 18, 348–351.
- Cosgrove, C.L., Knowles, S.C., Day, K.P. & Sheldon, B.C. (2006). No evidence for avian malaria infection during the nestling phase in a passerine bird. Journal of Parasitology, 92, 1302–1304. doi: 10.1645/GE-878R.1
- Cox, F.E.G. (1998). Babesiosis and malaria. In S.R. Palmer, E.J.L. Soulsby & D.I.H. Simpson (Eds.), Zoonoses (pp. 599–607). New York, NY: Oxford University Press.
- Cranfield, M.R. (2003). Sphenisciformes (penguins). In M.E. Fowler & R.E. Miller (Eds.), Fowler's Zoo and Wildlife Medicine (5th ed., pp. 107–108). Saint Louis, MO: Saunders.
- Cranfield, M.R., Graczyk, T.K., Beall, F.B., Ialeggio, D.M., Shaw, M.L. & Skjoldager, M.L. (1994). Subclinical avian malaria infections in African black-footed penguins (Spheniscus demersus) and induction of parasite recrudescence. Journal of Wildlife Diseases, 30, 372–376. doi: 10.7589/0090-3558-30.3.372
- Cranfield, M.R., Graczyk, T.K. & McCutchan, T.F. (2000). ELISA antibody test, PCR and a DNA vaccine for use with avian malaria in African penguins. In C.K. Baer & R.A. Pattersons (Eds.), Proceedings of the American Association of Zoo Veterinarians/International Association for Aquatic Animal Medicine Joint Conference (pp. 39–41). New Orleans, LA.
- Cranfield, M.R., Shaw, M.L., Beall, F.B., Skjoldager, M.L. & Ialeggio, D.M. (1990). A review and update of avian malaria in the African penguin (Spheniscus demersus). In J. Olsen (Ed.), Proceedings of the Annual Conference of the American Association of Zoo Veterinarians (pp. 243–248). South Padre Island, TX.
- Dietzmann, K., Kemnitz, P. & Schneidewind, J. (1985). Morphologic and histochemical studies of the development of chloroquine-induced storage disease in the semilunar ganglion of the rat. Acta Histochemica, 76, 37–47. doi: 10.1016/S0065-1281(85)80032-5
- Dinhopl, N., Mostegl, M.M., Richter, B., Nedorost, N., Maderner, A., Fragner, K. & Weissenböck, H. (2011). Application of in-situ hybridization for the detection and identification of avian malaria parasites in paraffin wax-embedded tissues from captive penguins. Avian Pathology, 40, 315–320. doi: 10.1080/03079457.2011.569533
- Dinhopl, N., Nedorost, N., Mostegl, M.M., Weissenbacher-Lang, C. & Weissenböck, H. (2015). In situ hybridization and sequence analysis reveal an association of Plasmodium spp. with mortalities in wild passerine birds in Austria. Parasitology Research, 114, 1455–1462. doi: 10.1007/s00436-015-4328-z
- Dufva, R. (1996). Blood parasites, health, reproductive success, and egg volume in female great tits Parus major. Journal of Avian Biology, 27, 83–87. doi: 10.2307/3676964
- Earle, R.A., Huchzermeyer, F.W., Bennett, G.F. & Brassy, J.J. (1993). Babesia peircei sp. nov. from the jackass penguin. South African Journal of Zoology, 28, 88–90. doi: 10.1080/02541858.1993.11448298
- Fallis, A.M., Bisset, S.A. & Allison, F.R. (1976). Leucocytozoon tawaki n.sp. (Eucoccida: Leucocytozoidae) from the penguin Eudyptes pachyrhynchus, and preliminary observations on its development in Austrosimulium spp. (Diptera: Simuliidae). New Zealand Journal of Zoology, 3, 11–16. doi: 10.1080/03014223.1976.9517893
- Fantham, H.B. & Porter, A. (1944). On a Plasmodium (Plasmodium relictum var. spheniscidæ, n. var.), observed in four species of penguins. Proceedings of the Zoological Society of London, 114, 279–292. doi: 10.1111/j.1096-3642.1944.tb00222.x
- Fix, S.A., Waterhouse, C., Greiner, E.C. & Stoskopf, M.K. (1988). Plasmodium relictum as a cause of avian malaria in wild-caught Magellanic penguins (Spheniscus magellanicus). Journal of Wildlife Diseases, 24, 610–619. doi: 10.7589/0090-3558-24.4.610
- Fleischman, R.W., Sladen, W.J.L. & Melby, E.C. (1968). Malaria (Plasmodium elongatum) in captive African penguins (Spheniscus demersus). Journal of the American Veterinary Medical Association, 153, 928–935.
- Fordyce, R.E. & Jones, C.M. (1990). Penguin history and new fossil material from New Zealand. In L.S. Davis & J.T. Darby (Eds.), Penguin Biology (pp. 417–446). London: Academic Press.
- Freed, L.A. & Cann, R.L. (2006). DNA quality and accuracy of avian malaria PCR diagnostics: a review. The Condor, 108, 459–473. doi: 10.1650/0010-5422(2006)108[459:DQAAOA]2.0.CO;2
- Gabaldon, A. & Ulloa, G. (1980). Holoendemicity of malaria: an avian model. Transactions of the Royal Society of Tropical Medicine and Hygiene, 74, 501–507. doi: 10.1016/0035-9203(80)90067-X
- Gailey-Phipps, J. (1978). A world survey of penguins in captivity. International Zoo Yearbook, 18, 7–13. doi: 10.1111/j.1748-1090.1978.tb00209.x
- Garamszegi, L.Z. (2011). Climate change increases the risk of malaria in birds. Global Change Biology, 17, 1751–1759. doi: 10.1111/j.1365-2486.2010.02346.x
- Garnham, P.C.C. (1966). Malaria Parasites and Other Haemosporidia. Oxford: Blackwell Scientific Public.
- Graczyk, T.K. & Cranfield, M.R. (1996). A model for the prediction of relative titres of avian malaria and Aspergillus spp. IgG in Jackass penguin (Spheniscus demersus) females based on maternal IgG in egg-yolk. International Journal for Parasitology, 26, 749–754. doi: 10.1016/0020-7519(96)00061-6
- Graczyk, T.K., Brossy, J.J., Plost, A. & Stoskopft, M.K. (1995a). Avian malaria seroprevalence in Jackass penguins (Spheniscus demersus) in South Africa. Journal of Parasitology, 81, 703–707. doi: 10.2307/3283958
- Graczyk, T.K., Cockrem, J.F., Cranfield, M.R., Darby, J.T. & Moore, P. (1995b). Avian malaria seroprevalence in wild New Zealand penguins. Parasite, 2, 401–405.
- Graczyk, T.K., Cranfield, M.R. & Bicknese, E.J. (1995c). Evaluation of serum chemistry values associated with avian malaria infections in African black-footed penguins (Spheniscus demersus). Parasitology Research, 81, 316–319. doi: 10.1007/BF00931537
- Graczyk, T.K., Cranfield, M.R., Brossy, J.B., Cockrem, J.F., Jouventin, P. & Seddon, P.J. (1995d). Detection of avian malaria infections in wild and captive penguins. Journal of the Helminthological Society of Washington, 62, 135–141.
- Graczyk, T.K., Cranfield, M.R., McCutchan, T.F. & Bicknese, E.J. (1994a). Characteristics of naturally acquired avian malaria infections in naive juvenile African black-footed penguins (Spheniscus demersus). Parasitology Research, 80, 634–637. doi: 10.1007/BF00932944
- Graczyk, T.K., Cranfield, M.R., Shaw, M.L. & Craig, L.E. (1994b). Maternal antibodies against Plasmodium spp. in African Black-footed Penguin (Spheniscus demersus) chicks. Journal of Wildlife Diseases, 30, 365–371. doi: 10.7589/0090-3558-30.3.365
- Graczyk, T.K., Cranfield, M.R., Skjoldager, M.L. & Shaw, M.L. (1994c). An ELISA for detecting anti-Plasmodium spp. antibodies in African black-footed penguins (Spheniscus demersus). Journal of Parasitology, 80, 60–66. doi: 10.2307/3283346
- Graczyk, T.K., Shaw, M.L., Cranfield, M.R. & Beali, F.B. (1994d). Hematologic characteristics of avian malaria cases in African Black-footed penguins (Spheniscus demersus) during the first outdoor exposure season. Journal of Parasitology, 80, 302–308. doi: 10.2307/3283762
- Grilo, M.L.A. (2014). Characterization of infection by malaria parasites in penguins housed in zoological collections (82 pp.). MSc thesis, University of Lisbon, Lisbon.
- Grim, K.C., McCutchan, T., Li, J., Sullivan, M., Graczyk, T.K., McConkey, G. & Cranfield, M. (2004). Preliminary results of an anticircumsporozoite DNA vaccine trial for protection against avian malaria in captive African black-footed penguins (Spheniscus demersus). Journal of Zoo and Wildlife Medicine, 35, 154–161. doi: 10.1638/03-060
- Grim, K.C., Van der Merwe, E., Sullivan, M., Parsons, N., McCutchan, T.F. & Cranfield, M. (2003). Plasmodium juxtanucleare associated with mortality in black-footed penguins (Spheniscus demersus) admitted to a rehabilitation center. Journal of Zoo and Wildlife Medicine, 34, 250–255. doi: 10.1638/02-070
- Griner, L.A. & Sheridan, B.W. (1967). Malaria (Plasmodium relictum) in penguins at the San Diego Zoo. Veterinary Clinical Pathology, 1, 7–17.
- Hellgren, O., Waldenström, J. & Bensch, S. (2004). A new PCR assay for simultaneous studies of Leucocytozoon, Plasmodium, and Haemoproteus from avian blood. Journal of Parasitology, 90, 797–802. doi: 10.1645/GE-184R1
- Huff, C.G. (1965). Susceptibility of mosquitoes to avian malaria. Experimental Parasitology, 16, 107–132. doi: 10.1016/0014-4894(65)90036-6
- Huff, C.G. & Shiroishi, T. (1962). Natural infection of Humboldt's penguin with Plasmodium elongatum. Journal of Parasitology, 48, 495. doi: 10.2307/3275227
- Huijben, S., Schaftenaar, W., Wijsman, A., Paaijmans, K. & Takken, W. (2007). Avian malaria in Europe: an emerging infectious disease? In W. Takken & B.G.J. Knols (Eds.), Emerging Pests and Vector-Borne Diseases in Europe (pp. 59–74). Wageningen: Wageningen Academic.
- Irwin, P. & Paskewitz, S. (2009). Investigation of fathead minnows (Pimephales promelas) as a biological control agent of Culex mosquitoes under laboratory and field conditions. Journal of the American Mosquito Control Association, 25, 301–309. doi: 10.2987/09-0013.1
- Jarvi, S.I., Schultz, J.J. & Atkinson, C.T. (2002). PCR diagnostics underestimate the prevalence of avian malaria (Plasmodium relictum) in experimentally-infected passerines. Journal of Parasitology, 88, 153–158. doi: 10.1645/0022-3395(2002)088[0153:PDUTPO]2.0.CO;2
- Jencek, J.E., Beaufrère, H., Tully, T.N.Jr., Garner, M.M., Dunker, F.H. & Baszler, T.V. (2012). An outbreak of Chlamydophila psittaci in an outdoor colony of magellanic penguins (Spheniscus magellanicus). Journal of Avian Medicine and Surgery, 26, 225–231. doi: 10.1647/2010-046R1.1
- Jones, H.I. & Shellam, G.R. (1999). Blood parasites in penguins, and their potential impact on conservation. Marine Ornithology, 27, 181–184.
- Jovani, R., Tella, J.L., Forrero, M.G., Bertellotti, M., Blanco, G., Ceballos, O. & Donázar, J.A. (2001). Apparent absence of blood parasites in the Patagonian seabird community: is it related to the marine environment? Waterbirds, 24, 430–433. doi: 10.2307/1522076
- Killick, R., Redrobe, S., Wenger, S. & Wyatt, K. (2008). Validation of a parasite lactate dehydrogenase (pLDH) test for malaria in two avian species. In L. Blackwood & D.J. Brockman (Eds.), Proceedings of the 51st Congress of the British Small Animal Veterinary Association (p. 473). Birmingham, UK.
- Kimura, M., Darbro, J.M. & Harrington, L.C. (2010). Avian malaria parasites share congeneric mosquito vectors. Journal of Parasitology, 96, 144–151. doi: 10.1645/GE-2060.1
- Klinghardt, G.W., Fredman, P. & Svennerholm, L. (1981). Chloroquine intoxication induces ganglioside storage in nervous tissues: a chemical and histopathological study of brain, spinal cord, spinal ganglia andretina in the miniature pig. Journal of Neurochemistry, 37, 897–908. doi: 10.1111/j.1471-4159.1981.tb04477.x
- Krams, I., Suraka, V., Cirule, D., Hukkanen, M., Tummeleht, L., Mierauskas, P., Rytkönen, S., Rantala, M.J., Vrublevska, J., Orell, M. & Krama, T. (2012). A comparison of microscopy and PCR diagnostics for low intensity infections of haemosporidian parasites in the Siberian tit Poecile cinctus. Annales Zoologici Fennici, 49, 331–340. doi: 10.5735/086.049.0506
- Krettli, A.U., Andrade-Neto, V.F., Brandão, M.G.L. & Ferrari, W.M.S. (2001). The search for new antimalarial drugs from plants used to treat fever and malaria or plants randomly selected: a review. Memórias do Instituto Oswaldo Cruz, 96, 1033–1042. doi: 10.1590/S0074-02762001000800002
- Laird, M. (1950). Some parasites of New Zealand birds. Victoria University College Zoological Publishings, 5, 1–20.
- Laird, M. & Van Riper, C. (1981). Questionable reports of Plasmodium from birds in Hawaii, with the recognition of P. relictum ssp. capistranoae (Russell, 1932) as the avian malaria parasite there. In E.U. Canning (Ed.), Parasitological Topics – A Presentation Volume to P.C.C. Garnham, F.R.S. on the Occasion of His 80th Birthday 1981 (pp. 159–165). Lawrence, KS: Allen Press.
- Langer, R.C. & Vinetz, J.M. (2001). Plasmodium ookinete-secreted chitinase and parasite penetration of the mosquito peritrophic matrix. Trends in Parasitology, 17, 269–272. doi: 10.1016/S1471-4922(01)01918-3
- Levin, I.I., Outlaw, D.C., Hernán-Vargas, F.G. & Parker, P.G. (2009). Plasmodium blood parasite found in endangered Galapagos penguins (Spheniscus mendiculus). Biological Conservation, 142, 3191–3195. doi: 10.1016/j.biocon.2009.06.017
- Levin, I.I., Zwiers, P., Deem, S.L., Geest, E.A., Higashiguchi, J.M., Iezhova, T.A., Jiménez-Uzcátegui, G., Kim, D.H., Morton, J.P., Perlut, N.G., Renfrew, R.B., Sari, E.H.R., Valkiūnas, G. & Parker, P.G. (2013). Multiple lineages of avian malaria parasites (Plasmodium) in the Galapagos Islands and evidence for arrival via migratory birds. Conservation Biology, 27, 1366–1377. doi: 10.1111/cobi.12127
- Mantilla, J.S., González, A.D., Valkiūnas, G., Moncada, L.I. & Matta, N.E. (2013). Description and molecular characterization of Plasmodium (Novyella) unalis sp. nov. from the Great Thrush (Turdus fuscater) in highland of Colombia. Parasitology Research, 112, 4193–4204. doi: 10.1007/s00436-013-3611-0
- Martinsen, E.S. & Perkins, S.L. (2013). The diversity of Plasmodium and other Haemosporidians: the interesection of taxonomy, phylogenetics and genomics. In J.M. Carlton, S.L. Perkins & K.W. Deitsch (Eds.), Malaria Parasites: Comparative Genomics, Evolution and Molecular Biology (pp. 1–15). Norfolk: Caister Academic Press.
- Marzal, A. (2012). Recent advances in studies on avian malaria parasites. In O. Okwa (Ed.), Malaria Parasites (pp. 135–158). Rijeka: InTech.
- McCutchan, T.F., Grim, K.C., Li, J., Weiss, W., Rathore, D., Sullivan, M., Graczyk, T.K., Kumar, S. & Cranfield, M.R. (2004). Measuring the effects of an ever-changing environment on malaria control. Infection and Immunity, 72, 2248–2253. doi: 10.1128/IAI.72.4.2248-2253.2004
- McDonald, S.P. (2012). Parasitology of the yellow-eyed penguin (Megadyptes antipodes) (214 pp.). MSc thesis, University of Otago, Otago.
- Meile, R.J., Lacy, R.C., Vargas, H. & Parker, P.G. (2013). Modeling Plasmodium parasite arrival in the Galapagos Penguin (Spheniscus mendiculus). The Auk, 130, 440–448. doi: 10.1525/auk.2013.12147
- Njunda, A.L., Assob, N.J.C., Nsagha, S.D., Kamga, F.H.L., Mokenyu, M.D. & Kwenti, T.E. (2013). Comparison of capillary and venous blood using blood film microscopy in the detection of malaria parasites: a hospital based study. Scientific Journal of Microbiology, 2, 89–94.
- Okanga, S., Cumming, G.S., Hockey, P.A.R., Grome, M. & Peters, J.L. (2013). A comparison of techniques employed in detection of avian malaria infection, South Africa. African Zoology, 48, 309–317. doi: 10.3377/004.048.0215
- Olliaro, P. (2001). Mode of action and mechanisms of resistance for antimalarial drugs. Pharmacology & Therapeutics, 89, 207–219. doi: 10.1016/S0163-7258(00)00115-7
- Outlaw, D.C. & Ricklefs, R.E. (2011). Rerooting the evolutionary tree of malaria parasites. Proceedings of the National Academy of Sciences of the United States of America, 108, 13183–13187. doi: 10.1073/pnas.1109153108
- Palinauskas, V., Valkiūnas, G., Križanauskienė, A., Bensch, S. & Bolshakov, C.V. (2009). Plasmodium relictum (lineage P-SGS1): further observation of effects on experimentally infected passeriform birds, with remarks on treatment with Malarone™. Experimental Parasitology, 123, 134–139. doi: 10.1016/j.exppara.2009.06.012
- Palmer, J.L., McCutchan, T.F., Hernan-Vargas, F., Deem, S.L., Cruz, M., Hartman, D.A. & Parker, P.G. (2013). Seroprevalence of malarial antibodies in Galapagos penguins (Spheniscus mendiculus). Journal of Parasitology, 99, 770–776. doi: 10.1645/12-57.1
- Paris, M., Tetreau, G., Laurent, F., Lelu, M., Despres, L. & David, J. (2011). Persistence of Bacillus thuringiensis israelensis (Bti) in the environment induces resistance to multiple Bti toxins in mosquitoes. Pest Management Science, 67, 122–128. doi: 10.1002/ps.2046
- Parsons, N.J. & Underhill, L.G. (2005). Oiled and injured African penguins Spheniscus demersus and other seabirds admitted for rehabilitation in the Western Cape, South Africa, 2001 and 2002. African Journal of Marine Science, 27, 289–296. doi: 10.2989/18142320509504087
- Penrith, M., Huchzermeyer, F.W., De Wet, S.C. & Penrith, M.J. (1994). Concurrent infection with Clostridium and Plasmodium in a captive king penguin Aptenodytes patagonicus. Avian Pathology, 23, 373–380. doi: 10.1080/03079459408419007
- Ploeg, M., Ultee, T. & Kik, M. (2011). Disseminated toxoplasmosis in black-footed penguins (Spheniscus demersus). Summarized from Avian Diseases, 55, 701–703. doi: 10.1637/9700-030411-Case.1
- Quillfeldt, P., Arriero, E., Martínez, J., Masello, J.F. & Merino, S. (2011). Prevalence of blood parasites in seabirds – a review. Frontiers in Zoology, 8, 26. doi: 10.1186/1742-9994-8-26
- Raethel, H.S. (1960). Plasmodieninfektionen bei Pinguinen des Berliner Zoologischen Gartens und ihre Bedeutung für die Pinguinhaltung. KleintierPraxis, 5, 64–70.
- Remple, J.D. (2004). Intracellular hematozoa of raptors: a review and update. Journal of Avian Medicine and Surgery, 18, 75–88. doi: 10.1647/2003-008
- Richard, F.A., Sehgal, R.N.M., Jones, H.I. & Smith, T.B. (2002). A comparative analysis of PCR-based detection methods for avian malaria. Journal of Parasitology, 88, 819–822. doi: 10.1645/0022-3395(2002)088[0819:ACAOPB]2.0.CO;2
- Rodhain, J. (1939). L'infection a Plasmodium relictum chez les pingouins. Annales de Parasitologia, 17, 139–157.
- Scott, H.H. (1927). Report on the deaths occurring in the society's gardens during the year 1926. Proceedings of the Zoological Society of London, 97, 173–198. doi: 10.1111/j.1096-3642.1927.tb02254.x
- Silveira, P., Belo, N.O., Lacorte, G.A., Kolesnikovas, C.K.M., Vanstreels, R.E.T., Steindel, M., Catão-Dias, J.L., Valkiūnas, G. & Braga, E.M. (2013). Parasitological and new molecular-phylogenetic characterization of the malaria parasite Plasmodium tejerai in South American penguins. Parasitology International, 62, 165–171. doi: 10.1016/j.parint.2012.12.004
- Smith, K.F., Sax, D.F. & Lafferty, K.D. (2006). Evidence for the role of infectious disease in species extinction and endangerment. Conservation Biology, 20, 1349–1357. doi: 10.1111/j.1523-1739.2006.00524.x
- Stockklausner, J., Czajka, C., Jöst, H., Borner, J., Saenger, K., Schmidt, V., Becker, N. & Tannich, E. (2013). Avian malaria in Inca terns at the zoo Heidelberg and the mosquito control program. In J. Chitty, R. Marschang, J. Mayer, B. Speer, M. Lierz, U. Heffels-Redmann & L. Ziegler (Eds.), Proceedings of the 1st International Conference on Avian, Herpetological & Exotic Mammal Medicine (ICARE) (p. 409). Wiesbaden, Germany.
- Stoskopf, M.K. & Beall, F.B. (1980). The husbandry and medicine of captive penguins. In W. Armand (Ed.), Proceedings of the Annual Conference of the American Association of Zoo Veterinarians (pp. 81–96). Washington, DC.
- Stoskopf, M.K. & Beier, J. (1979). Avian malaria in African black-footed penguins. Journal of the American Veterinary Medical Association, 175, 944–947.
- Sturrock, H.J.W. & Tompkins, D.M. (2007). Avian malaria (Plasmodium spp) in yellow-eyed penguins: investigating the cause of high seroprevalence but low observed infection. New Zealand Veterinary Journal, 55, 158–160. doi: 10.1080/00480169.2007.36761
- Szöllsi, E., Hellgren, O. & Hasselquist, D. (2008). A cautionary note on the use of nested PCR for parasite screening – an example from avian blood parasites. Journal of Parasitology, 94, 562–564. doi: 10.1645/GE-1286.1
- Tetreau, G., Stalinski, R., David, J. & Després, L. (2013). Monitoring resistance to Bacillus thuringiensis subsp. israelensis in the field by performing bioassays with each Cry toxin separately. Memórias do Instituto Oswaldo Cruz, 108, 894–900. doi: 10.1590/0074-0276130155
- Thiart, H. (2005). Immunological and epidemiological investigations into avian malaria in the African penguin during rehabilitation and in breeding colonies (134 pp.). MSc thesis, University of Stellenbosch, Stellenbosch.
- Thurber, M.I., Gamble, K.C., Krebs, B. & Goldberg, T.L. (2014). Molecular detection of Plasmodium in free-ranging birds and captive flamingos (Phoenicopterus chilensis) in Chicago. Journal of Zoo and Wildlife Medicine, 45, 749–754. doi: 10.1638/2013-0284.1
- Tollini, J., Brecksen, A. & Sureda, A. (2000). Prevention and treatment of avian malaria in a captive penguin colony. Penguin Conservation, 13, 26–27.
- Tompkins, D.M. & Gleeson, D.M. (2006). Relationship between avian malaria distribution and an exotic invasive mosquito in New Zealand. Journal of the Royal Society of New Zealand, 36, 51–62. doi: 10.1080/03014223.2006.9517799
- United States Geological Survey. (2006). Ecology and Diagnosis of Introduced Avian Malaria in Hawaiian Forest Birds (USGS FS 2005–3151).
- Valkiūnas, G. (2005). Avian Malaria Parasites and Other Haemosporidia. Boca Raton, FL: CRC Press.
- Valkiūnas, G., Bensch, S., Iezhova, T.A., Križanauskienė, A., Hellgren, O. & Bolshakov, C.V. (2006). Nested cytochrome b polymerase chain reaction diagnostics underestimate mixed infections of avian blood haemosporidian parasites: microscopy is still essential. Journal of Parasitology, 92, 418–422. doi: 10.1645/GE-3547RN.1
- Valkiūnas, G., Palinauskas, V., Ilgūnas, M., Bukauskaitė, D., Dimitrov, D., Bernotienė, R., Zehtindjiev, P., Ilieva, M. & Iezhova, T.A. (2014). Molecular characterization of five widespread avian haemosporidian parasites (Haemosporida), with perspectives on the PCR-based detection of haemosporidians in wildlife. Parasitological Research, 113, 2251–2263. doi: 10.1007/s00436-014-3880-2
- Van Rensburg, M.J. (2010). Parasitism, disease and breeding ecology of little blue penguins (Eudyptula minor) on Tiritiri Matangi Island, New Zealand (240 pp.). MSc thesis, Massey University, Auckland.
- Van Riper, C., Van Riper, S.G., Goff, M.L. & Laird, M. (1986). The epizootiology and ecological significance of malaria in Hawaiian land birds. Ecological Monographs, 56, 327–344. doi: 10.2307/1942550
- Vanstreels, R.E.T., Kolesnikovas, C.K.M., Sandri, S., Silveira, P., Belo, N.O., Junior, F.C.F., Epiphanio, S., Steindel, M., Braga, E.M. & Catão-Dias, J.L. (2014). Outbreak of avian malaria associated to multiple species of Plasmodium in Magellanic penguins undergoing rehabilitation in Southern Brazil. PLoS ONE, 9, e94994. doi: 10.1371/journal.pone.0094994
- Vanstreels, R.E.T. & Parsons, N.J. (2014). Malária Aviária e outros Hemosporídeos Aviários. In Z.S. Cubas, J.C.R. Silva & J.L. Catão-Dias (Eds.), Tratado de Animais Selvagens (2nd ed., Vol. 2, pp. 1427–1443). São Paulo: Roca.
- Vanstreels, R.E.T., Silva-Filho, R.P., Kolesnikovas, C.K.M., Bhering, R.C.C., Ruoppolo, V., Epiphanio, S., Amaku, M., Junior, F.C.F., Braga, E.M. & Catão-Dias, J.L. (2015). Epidemiology and pathology of avian malaria in penguins undergoing rehabilitation in Brazil. Veterinary Research, 46, 30. doi: 10.1186/s13567-015-0160-9
- Vinetz, J.H., Clain, J., Bounkeua, V., Eastman, R.T. & Fidock, D. (2011). Chemotherapy of malaria. In L.L. Brunton (Ed.), Goodman and Gilman's the Pharmacological Basis of Therapeutics (12th ed., pp. 1383–1418). New York, NY: McGraw-Hill Professional.
- Waldenström, J., Bensch, S., Hasselquist, D. & Östman, Ö. (2004). A new nested polymerase chain reaction method very efficient in detecting Plasmodium and Haemoproteus infections from avian blood. Journal of Parasitology, 90, 191–194. doi: 10.1645/GE-3221RN
- Wallace, R.S. (2014). Sphenisciformes (penguins). In M.E. Fowler & R.E. Miller (Eds.), Fowler's Zoo and Wildlife Medicine (8th ed., pp. 82–88). Saint Louis, MO: Saunders.
- Warner, R.E. (1968). The role of introduced diseases in the extinction of the endemic Hawaiian avifauna. The Condor, 70, 101–120. doi: 10.2307/1365954
- Willette, M., Ponder, J., Cruz-Martinez, L., Arent, L., Padilla, I.B., Nicolas de Francisco, O. & Redig, P. (2009). Management of select bacterial and parasitic conditions of raptors. Veterinary Clinics of North America: Exotic Animal Practice, 12, 491–517.
- Williams, R.B. (2005). Avian malaria: clinical and chemical pathology of Plasmodium gallinaceumin the domesticated fowl Gallus gallus. Avian Pathology, 34, 29–47. doi: 10.1080/03079450400025430
- Wünschmann, A., Armien, A., Wallace, R., Wictor, M. & Oglesbee, M. (2006). Neuronal storage disease in a group of captive Humboldt penguins (Spheniscus humboldti). Veterinary Pathology, 43, 1029–1033. doi: 10.1354/vp.43-6-1029
- Zehtindjiev, P., Ilieva, M., Westerdahl, H., Hansson, B., Valkiūnas, G. & Bensch, S. (2008). Dynamics of parasitemia of malaria parasites in a naturally and experimentally infected migratory songbird, the great reed warbler Acrocephalus arundinaceus. Experimental Parasitology, 119, 99–110. doi: 10.1016/j.exppara.2007.12.018
- Zehtindjiev, P., Križanauskienė, A., Bensch, S., Palinauskas, V., Asghar, M., Dimitrov, D., Scebba, S. & Valkiūnas, G. (2012). A new morphologically distinct avian malaria parasite that fails detection by established polymerase chain reaction-based protocols for amplification of the cytochrome B gene. Journal of Parasitology, 98, 657–665. doi: 10.1645/GE-3006.1