ABSTRACT
Constant monitoring is performed to elucidate the role of natural hosts in the ecology of Newcastle disease virus (NDV). In this study, an NDV strain isolated from an asymptomatic pigeon was sequenced and analysed. Results showed that the full-length genomes of this isolate were 15,198 nucleotides with the gene order of 3′-NP-P-M-F-HN-L-5′. This NDV isolate was lentogenic, with an intracerebral pathogenicity index of 0.00 and a mean time of death more than 148 h. The isolate possessed a motif of -112E-R-Q-E-R-L117- at the F protein cleavage site. In addition, 7 and 13 amino acid substitutions were identified in the functional domains of fusion protein (F) and haemagglutinin-neuraminidase protein (HN) proteins, respectively. Analysis of the amino acids of neutralizing epitopes of F and HN proteins showed 3 and 10 amino acid substitutions, respectively, in the isolate. Phylogenetic analysis classified the isolate into genotype Ib in Class I. This isolate shared high homologies with the NDV strains isolated from wild birds and waterfowl in southern and eastern parts of China from 2005 to 2013. To our knowledge, this study is the first to report a NDV strain isolated from pigeon that belongs to genotype Ib in Class I, rather than to the traditional genotype VI or other sub-genotypes in Class II. This study provides information to elucidate the distribution and evolution of Class I viruses for further NDV prevention.
Introduction
Newcastle disease (ND) is one of the most highly pathogenic viral diseases of avian species because it causes high mortality and morbidity rates (Alexander, Citation2001). ND is caused by Newcastle disease virus (NDV), which is also known as avian paramyxovirus type 1 (APMV-1); this virus belongs to the genus Avulavirus and subfamily Paramyxovirinae (family Paramyxoviridae, order Mononegavirales) (Mayo, Citation2002). The NDV genome is non-segmented and contains single-stranded negative-sense RNA (Czegledi et al., Citation2006), with six transcriptional units encoding nucleocapsid protein (NP), phosphoprotein (P), matrix protein (M), fusion protein (F), haemagglutinin-neuraminidase protein (HN) and polymerase protein (L). Two additional proteins, namely, V and W proteins, are derived from the P gene via RNA editing (Steward et al., Citation1993; Jacques et al., Citation1994). NDV is generally categorized based on pathogenicity in chickens into three pathotypes, namely, velogenic, mesogenic or lentogenic. NDV strains can be separated into Classes I and II based on genomic size and sequence analysis. Class I viruses, with a genome size of 15,198 nucleotides (nt), are mostly avirulent and frequently isolated from wild waterfowl and live bird markets. Class I has been recently grouped into nine genotypes (Kim et al., Citation2007). Class II viruses comprise most virulent NDVs, and at least 18 genotypes have been identified in this group (Snoeck et al., Citation2013). Genotypes I–IV viruses are early sub-lineages before 1960, with a genome size of 15,186 nt, whereas NDV isolates after 1960 present a genome size of 15,192 nt (Czegledi et al., Citation2006; Ujvari et al., Citation2006; Maminiaina et al., Citation2010; Diel et al., Citation2012).
NDV has a wide host range and can infect at least 250 species in most avian orders. Wild birds and waterfowl, which are generally considered as natural NDV reservoirs, harbour lentogenic strains and occasionally carry velogenic strains (Collins et al., Citation1996; Kim et al., Citation2007; Lindh et al., Citation2012). Pigeon paramyxovirus type 1 (PPMV-1), which is responsible for autonomous ND-like infectious disease in pigeons, is an antigenic and host variant of NDV from chickens (Kaleta et al., Citation1985; Collins et al., Citation1994). PPMV-1 seriously affects pigeon or dove populations. By contrast, domestic birds may show few or no clinical signs in response to PPMV-1 strains, which are markedly virulent for pigeons (Meulemans et al., Citation2002; Guo et al., Citation2014; Smietanka et al., Citation2014). Under natural conditions, the risk posed by virus isolated from pigeons to the poultry industry appears after serial passages in chickens or embryonated eggs; hence, the virulence of the virus increases by passaging in different hosts (Liu et al., Citation2006; Irvine et al., Citation2009; Dortmans et al., Citation2011). It is widely accepted that cleavage of the F protein precursor (F0) is the primary determinant of NDV virulence. Virulent (velogenic or mesogenic) NDV strains contain a multi-basic amino acid-rich region (112R/K-R-X-R/K-R-F117) in the cleavage site whereas avirulent (lentogenic) strains possess 112G/E-K/R-X-G/E-R-L117 motif cleaved by secreted trypsin-like protease into active F protein, which consists of disulphide-linked F1 and F2 polypeptides (Nagai et al., Citation1976; Glickman et al., Citation1988; De Leeuw et al., Citation2003). However, a recent study using the reverse genetics system showed that the virulence of NDV is not always correlated with the cleavability of the F protein, and viral pathogenicity could be determined through the activity of viral replication proteins, such as the ribonucleoprotein complex (Collins et al., Citation1994, Citation1996; Dortmans et al., Citation2009, Citation2010, Citation2011).
Most NDV isolates from pigeons are classified based on phylogenetic tree analysis into a distinct sub-lineage (VIb) or other sub-lineages in Class II (Meulemans et al., Citation2002). Since 1985, PPMV-1, which is also classified into genotype VI or sub-genotype in Class II, has been circulated and documented in several provinces in China (Liu et al., Citation2006). Class I isolates are usually recovered from natural hosts, such as wild birds or waterfowl, and most of these isolates present low virulence (Kim et al., Citation2007). Limited information is available about the distribution, genetic diversity or ability of these viruses to cause ND outbreaks in poultry and natural hosts because of the current lack of epidemic surveillance data on wild birds. Therefore, constant monitoring is required to elucidate the role of natural reservoir in the ecology of NDV. Our previous study described the pathogenic and phylogenetic characteristics of several wild bird NDVs, such as Shaanxi06 (velogenic, genotype VIId), Shaanxi10 (mesogenic, genotype VIi), Spotted-dove08 (letogenic, genotype IX) and Blackbird08 (velogenic, genotype IX) (Chen et al., Citation2013; Liu et al., Citation2013). In the present study, an NDV strain was isolated from a pigeon in China. The complete genome sequence of this isolate was determined and analysed. However, phylogenetic analysis classified this NDV strain into genotype Ib in Class I, rather than into the traditional genotype VI in Class II.
Materials and methods
Sample collection
In November 2014, 34 tracheal and cloacal swabs were collected from clinically healthy pigeons in Huangyuan County, Qinghai Province, northwest of China. Pigeons with shiny soot-black feathers and iridescent green back, chest and crown were identified as wood pigeons, which belong to the genus Columba and the family Columbidae. These pigeons are mainly used for ornamental and competitive purposes. They forage for food ad libitum in nature during the day and take shelter in peasant households at night.
Virus isolation and identification. Swab samples were soaked in phosphate-buffered saline (PBS) with penicillin (1000 U/ml) and streptomycin (100 mg/ml) overnight at 4°C. After centrifugation, supernatants were inoculated into the allantoic cavity of 10-day-old specific pathogen-free (SPF) chicken embryos (Merial-vital Laboratory Animal Technology, Beijing, China). Allantoic fluid was harvested after 3 days of incubation at 37°C. After three series of blind passages, the virus in the harvested allantoic fluid was tested using haemagglutination (HA) and haemagglutination inhibition (HI) with standard NDV- and avian influenza virus (AIV)-specific sera according to the OIE operation standard (OIE, Citation2008). HA-positive allantoic fluids were stored at −70°C, and the NDV isolates were further purified through plaque purification on chicken embryo fibroblast cells.
Pathogenicity assessment
Pathogenicity tests were performed according to the OIE standard procedures to determine mean time of death (MDT) in 10-day-old embryonated chicken eggs and intracerebral pathogenicity index (ICPI) in 1-day-old SPF chickens (OIE, Citation2008). Two groups with 10 one-day-old SPF layer chickens were housed in separate isolators. The first group was inoculated intracerebrally with 10-fold dilution of infectious viral dose (HA >4) of 0.1 ml of this NDV isolate. The second group was inoculated intracerebrally phosphate-buffered saline at a similar dose. The MDT of this isolate was tested. Meanwhile, six groups with 10 ten-day-old SPF chicken embryos were used, and each embryo was inoculated with a serial dilution of infectious dose (10−6–10−10) per 0.1 ml. MDT and ICPI levels were recorded and calculated.
All the experimental procedures were approved by an independent Animal Care and Use Committee in Shaanxi Province, China. The experiments were performed in accordance with the guidelines formulated by the World Organisation for Animal Health and Welfare Standards (OIE, Citation2008).
Viral RNA preparation, RT-PCR and sequencing analysis
Total RNA was extracted from allantoic fluids by using RNAiso Plus Reagent (TakaRa Biotechnology Dalian, Dalian, China). cDNA was synthesized using PrimeScrip First-strand cDNA Synthesis Kit (TakaRa Biotechnology Dalian) with random hexamers according to the manufacturer’s instruction. Ten pairs of primers were designed based on the sequences published on Genbank (accession number: KM885161). The primer sequences are listed in . PCR amplification was performed in a 50 μl reaction volume containing 10 pmol of each primer, 200 ng of cDNA as template and 2.5 U Pfu polymerase (TransStartTM Fast Pfu DNA Polymerase, TransGenBiotech, Beijing, China) following the manufacturer’s instructions. The purified PCR products were cloned into the pMD19-T vector (TaKaRa Biotechnology Dalian). At least three independent plasmids containing each PCR fragment were purified and sequenced in an ABI 3730 genetic analyzer (Beijing Genomics Institute, Beijing, China).
Table 1. Primers used for amplification of complete genome of the NDV isolate.
Sequencing of the 3′ and 5′ ends of viral genome
The 3′ and 5′ end sequences of both viral genomes were subjected to rapid amplification of cDNA ends by using previously described methods (Li et al., Citation2005).
Phylogenetic tree and evolutionary distance analyses
Nucleotide sequence editing and analysis, as well as amino acid sequence prediction and alignments, were conducted using the DNASTAR software suite (version 3.1; DNASTAR, Madison, WI, USA). NDV sequences representing different genotypes and sub-genotypes were downloaded from Genbank. The nucleotide and amino acid sequences of the isolates were assembled and aligned. The complete genomic sequences of the NDV isolate were deposited in Genbank (Accession number: KT223818). Nucleotide sequence similarity was processed using the Clustal W multiple alignment algorithm of the DNASTAR software suite (version 3.1; DNASTAR, Madison, WI, USA). An unrooted phylogenetic tree was constructed using the MEGA (version 6.02) software with the Kimura two-parameter model and the Maximum Likelihood method algorithm with 1000 bootstraps based on the complete sequences of the F gene (1662 bp) (Tamura et al., Citation2011; Diel et al., Citation2012). The evolutionary distances among genotype I or sub-genotypes were analysed using the Maximum Likelihood method with 1000 bootstraps in the MEGA software (version 6.02).
Results
Virus isolation and identification
After a series of passages in 10-day-old SPF chicken embryos, one allantoic fluid sample from a tracheal swab was determined as HA-positive; the HA titre could be specifically inhibited by NDV-positive serum but not by AIV-positive serum; the HA and HI titres were 27 and 23, respectively. This isolate was further purified in chicken embryo fibroblast cells by using plaque assay. This NDV isolate was obtained and designated as NDV/pigeon/China/Qinghai-01/2014 (abbreviated as pigeon/QH-01/14).
Pathogenicity assessment of the NDV isolates
This NDV isolate (Pigeon/QH-01/14) exhibited an MDT of more than 148 h in 10-day-old SPF embryonated chicken eggs, with an ICPI value of 0.00 in 1-day-old chicks. This NDV isolate shared the motif of -112E-R-Q-E-R-L117- at the F0 cleavage site (). The pathogenicity assessment and molecular characterization analysis indicated that this isolate was a lentogenic NDV strain.
Determination of the complete genome sequence
Ten overlapping fragments covering the full-length genome of this NDV isolate were amplified by RT-PCR. The genomes were assembled with the software suite DNASTAR. The detailed information of genome-length features and protein characteristics of this NDV isolate is presented in . The NDV isolate genome, with 15,198 nt in length, comprised six open reading frames (ORFs) in the order 3′-NP-P-M-F-HN-L-5′ and followed the “rule of six”, which is a common rule relied upon by other Paramyxoviridae members (Kolakofsky et al., Citation2005). In contrast to the genome sequences (15,186 nt) of the classical vaccine strain LaSota in Class II, the NDV isolate contained a 12 nt insertion site (CAGGAAACGGGG) in the 5′ coding region of the P gene between the nucleotides 2381 and 2382. Another NDV isolate (abbreviated as pigeon/QH-02/14) collected from cloacal swab sample from another pigeon at the same time was also sequenced and analysed. The isolate exhibited 99.9% nucleotide sequence homologies with isolate pigeon/QH-01/14. The variations were only found in the L gene with 99.7% nucleotide homology (data not shown).
Table 2. Genome-length features and protein characteristics of the pigeon NDV isolate from China.
Sequences of non-coding regions
The genomes generally started at the 3′-leader region, and genes were separately transcribed into mRNAs with a start–stop restart mechanism guided by the conserved gene start (GS), gene end (GE) and non-coding intergenic sequences (IGSs) located between GS and GE in the NDV genomes; these sequences affected the transcription efficiency of the six NDV genes (Krishnamurthy & Samal, Citation1998). Similar to other NDVs, the GS between NP–P–M–F and HN of this NDV isolate is ACGGGTAGAA, whereas that between the HN and L genes is ACGGGTAGGA. In addition, the GE of NP, M, F, HN and L is TTAGAAAAAA, whereas that for the P gene is TAAGAAAAAA. (Silent mutations in GE and GS are shown in bold and italics). The IGS lengths of the six structural genes of this isolate are also presented in .
Analyses of F and HN amino acids
The cleavability of the precursor F0 glycoprotein into active F1 and F2 polypeptides by cellular proteases before gaining functional activity is an absolute prerequisite for the virulence of NDV (Nagai et al., Citation1976; Glickman et al., Citation1988; De Leeuw et al., Citation2003). shows the alignment of the F protein cleavage site of this NDV strain isolated in this study, as well as other typical genotypes in Classes I and II. The poly-basic amino acid sequence (112G/R-R-K/Q-K-R-F117) at the F cleavage site of NDV isolates from pigeons is often characteristic of a velogenic pathotype. However, the cleavage sites of this NDV isolate possessed the -112E-R-Q-E-R-L117- cleavage site, which is lentogenic and characterized most NDV strains in Class I.
The consensus amino acid sequences derived from NDV strains of different genotypes in Class I were compared. shows that the major functional domains of the F protein of the NDV strain isolated in this study contained seven amino acid substitutions (five at the signal peptide and one each at the induced fusion peptide and HRc). Three amino acid substitutions (A-75-Q, A-79-S and D-170-S) of the neutralizing epitopes of the F protein were also identified (). In addition, the F protein contained six highly conserved potential glycosylation sites (McGinnes et al., Citation2001) Asn-X-Ser/Thr (N-X-S/T) in the F protein at 85, 191, 366, 447 and 471 residues, which are conserved, and at 542 residues, which is not conserved. Cysteine residues play an important role in the connection between F1 and F2 subunits. The conserved cysteine in the C-terminal is also necessary to maintain the F protein structure. Cysteine residues are generally highly conserved at positions 25, 27, 76, 199, 338, 347, 362, 370, 394, 399, 401, 424, 514 and 523 of the F protein in most NDV isolates. Amino acids at these sites were used and conserved in this NDV isolate, except at 25 and 27 amino acids, which were replaced by M and G, respectively.
Table 3. Amino acid substitutions in the neutralizing epitopes and the functional domains of F protein.
The HN protein of this NDV isolate comprised 616 amino acids; this finding is consistent with those observed in the other NDV strains of Class I (Sakaguchi et al., Citation1989; Romer-Oberdorfer et al., Citation2003). It spanned the membrane once and possessed three distinct domains, including an N-terminal transmembrane domain (TM), a stalk region and a large C-terminal globular domain. The stalk of the HN protein formed a parallel tetrameric coiled-coil bundle (4 HB), which supports the head and directly interacts with the F protein. The HN–F interaction was mediated by a stretch of the conserved amino acids from 74 to 110 in the HN protein, including two conserved heptad repeats (HRA and HRB) (Stone-Hulslander & Morrison, Citation1999). indicates that the conserved heptad repeat (HR) and N-terminal TM of the HN proteins contained seven and six amino acid substitutions, respectively. Several studies have identified several neutralizing antigenic sites within the HN with monoclonal antibodies (MAb) (Iorio et al., Citation1991, Citation1992, Citation2001). Ten amino acid substitutions of the neutralizing epitopes of the C-terminal globular head of HN protein were identified, as shown in . Importantly, five amino acid substitutions (L345P, E347D, Q348H, D349E and I352V) were identified proximally to the receptor-binding site in primary linear epitopes of the HN protein of this isolate; those epitopes formed a partial α-helix and loop structures (Iorio et al., Citation1991, Citation1992; Cho et al., Citation2007). In addition, 12 cysteine residues were highly conserved at positions 123, 155, 172, 186, 196, 238, 247, 251, 344, 461, 465, 531 and 542 on the HN protein (Sakaguchi et al., Citation1989; McGinnes & Morrison, Citation1994; Pitt et al., Citation2000); these residues play an important role in the maturity of the HN protein, and most of them were conserved in this isolate, except at C-155-A. The role of N-linked carbohydrate (G1–G6) in the activities of the HN glycoprotein includes cell attachment, fusion-promotion activities, folding efficiency and mature protein formation (Ronin et al., Citation1978; McGinnes & Morrison, Citation1995). The HN protein of this isolate contained six potential N-glycosylation sites (G1–G6) N-X-S/T- at 119, 341, 433 and 481, which were conserved, and at 508 and 538, which were not conserved and used in this study.
Table 4. Amino acid constitutions in the antigenic epitopes and functional domains of the HN protein.
Phylogenetic tree and evolutionary distance analyses
A phylogenetic tree () was constructed based on the complete sequences of the F gene by using MEGA 6.02 (Tamura et al., Citation2011; Diel et al., Citation2012). Interestingly, this NDV isolate was classified into genotype Ib in Class I, which is a genotype epidemic in wild bird and waterfowl populations. This NDV isolate showed the highest nucleotide identities (99.5%) with a lentogenic NDV strain, which was isolated from wild bird and belonged to Class I Peafowl/China/LHL/131047 (Accession number: KJ607171). This isolate also showed 97.6% identities with the lentogenic NDV strain belonging to Class I Duck/LH/LHuB/085/2013 (Accession number: KM885161); those lentogenic strains were isolated between 2005 and 2013 in the southern and eastern parts of China. However, the nucleotide sequence homologies of this isolate were 53.5% and 71.8% with the LaSota strains, which are vaccine strains widely used in China, and PPMV-1, which was isolated in China in 2013 (Accession number: KM374059), respectively. Moreover, some evolutionary distances were still present. The estimated evolutionary distance between this isolate and other genotype I NDV strains (Ia, Ib and Ic) ranged from 0.010 to 0.086 (), of which the smallest evolutionary distance was 0.010 with the NDV strain Peafowl/China/LHL/131047. These findings provide evidence of the continuous evolutionary divergence in sub-genotype I in Class I.
Figure 2. Unrooted phylogenetic tree of different NDV strains based on complete sequence of the F genes of NDVs in Class I and Class II. Genotype and sub-genotype groupings are shown on the right. The evolutionary history was inferred using the Maximum Likelihood method (Tamura et al., Citation2011; Diel et al., Citation2012). Analysis was conducted using the Kimura 2-parameter method with 1000 bootstrap replicates in MEGA 6.02. The phylogenetic tree was drawn to scale with the highest log-likelihood (–3428.94), with branch lengths measured as the number of substitutions per side. No discrete gamma distribution was used to model evolutionary rate difference among sites (uniform rates). All positions containing gaps and missing data treatment were partial deletion with a site coverage cut-off 95%. First, second, third and non-coding positions were included. Toggling the display of branch lengths was shown above the branches. The NDV strain used in this study is indicated by a filled black circle. NDV strains used in Class I and Class II are referred to in the literature (Kim et al., Citation2007; Diel et al., Citation2012; Liu et al., Citation2013; Fan et al., Citation2015; Wu et al., Citation2015).
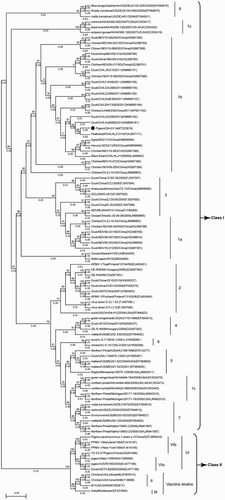
Table 5. Estimates of evolutionary distances among the pigeon NDV isolate and other genotype I NDV strains in Class I.
Discussion
ND causes high mortality and morbidity rates, resulting in great economic losses (Alexander, Citation2001). Most studies have focused on strains isolated from commercial poultry because of their severe economic impact on the poultry industry, whereas few studies investigated NDV strains circulating in wild birds. Chickens and pigeons show varied susceptibility to different NDV strains, regardless of APMV-1 or PPMV-1 infection (De Oliveira Torres Carrasco et al., Citation2008; Guo et al., Citation2014). Several APMV-1 outbreaks in chickens have been attributed to PPMV-1, which is regarded as a real threat to the poultry industry (Liu et al., Citation2006; Irvine et al., Citation2009). Most NDVs isolated from pigeons generally belong to genotype VIb or other sub-genotypes. A phylogenetic tree () was constructed based on complete F gene sequences to determine the genotype of this isolate. The phylogenetic analysis result suggested that this NDV strain belonged to genotype Ib in Class I, instead of genotype VI in Class II. Class I NDVs, which are rarely reported, mainly circulate in wild waterfowl and live bird markets in many countries, including China (Kim et al., Citation2007). Currently, Class I NDVs are also found in domestic poultry, and one isolate from this class is considered virulent (Alexander et al., Citation1992). To our knowledge, this study is the first to report Class I NDV strains isolated from pigeons. Our study provides information that the pigeon tends to be infected by different genotypes of NDV strains, rather than by genotype VIb or sub-genotypes only. The phylogenetic tree and evolutionary distance results indicated that this NDV isolate was highly similar to NDVs isolated from waterfowl and wild bird hosts. Therefore, the NDV strain isolated in the present study may be acquired from waterfowl and wild birds and spread to pigeons. Pigeons could be infected through contact with wild birds, which share similar habitats, considering that pigeons exhibit free-flying characteristics and can interact with wild bird and waterfowl species. Moreover, several viruses can continue to evolve into new genotypes from the current virus strains through homologous recombination with the circulating viruses. The current virus strains could have reversely evolved to the early genotype in Class I because of immunity pressure.
Previous studies reported that the fusion activity of the F protein can be affected by amino acid substitutions in the signal peptide, induced fusion peptide, HR region or replacement of the TM domain (Morrison, Citation2003; White et al., Citation2008). This specificity between HN and F is consistent with the interaction mediated by the specific domain on both proteins. The binding to the HN cell receptor triggers its interaction with the F protein, which leads to conformational changes in the protein for fusion (Takimoto et al., Citation2002). Generally, changes in the amino acid of F and HN are relevant to the tertiary structure and charge of proteins. In this study, 7 and 13 amino acid substitutions were observed in the functional domains of the F and HN proteins, respectively, of this NDV isolate ( and ). The HR motifs of HN and F proteins mediate protein–protein interactions by inducing the formation of coils (Stone-Hulslander & Morrison, Citation1999). Amino acids at these sites were used and conserved in this NDV isolate, except at 489 amino acid of HRc of HN replaced by D, and at L-74-F, S-76-V, N-77-S, V-81-I, T-101-S, T-102-L and I-107-L of HR of F. Analysis of the neutralizing epitopes at the C-terminal globular heads of F and HN also showed 3 and 10 amino acid substitutions, respectively ( and ). Amino acid substitutions in neutralizing epitopes, which play an important role in the formation of antigenic epitopes, induced the formation of neutralizing escape variants (Iorio et al., Citation1992; Hu et al., Citation2010; Choi et al., Citation2013). Three determinant amino acids of neutralizing epitopes of F possessed unique substitutions at positions 75, 79 and 170 compared with the consensus amino acid sequence derived from NDV strains of different genotypes in Class I. Computational conformation analysis showed that seven antigenic sites (1, 2, 3, 4, 1–2, 2–3 and 1–4) form a continuum in the 3D conformation of the HN molecule (Iorio et al., Citation1991, Citation1992, Citation2001; Cho et al., Citation2007). A single amino acid substitution in the major, well-characterized, linear epitope (345LDEQDYQIR353) containing α-helix and loop structure was located in the receptor-binding pocket of HN, resulting in the evasion of the antigenic variant from neutralization by mouse MAb (Iorio et al., Citation1991, Citation1992; Cho, et al., Citation2007; Hu, et al., Citation2010). Thirteen substitutions at neutralizing antigenic sites, including primary linear epitopes (L345P, E347D, Q348H, D349E and I352V), were found in the high antigenic variability of F and HN proteins in this isolate ( and ). Therefore, antigenic variants may have evolved from different selective pressures. To determine the correlation of antigenic site characteristics with this NDV isolate and other strains, a future comparative study with cross HI and virus neutralization tests must be performed. The characteristics of the HN and F mutations of this NDV isolate should also be investigated.
In summary, this study described and analysed the complete genome of a NDV isolate which was isolated from the trachea of a healthy pigeon. Phylogenetic tree and evolutionary distance analyses revealed that the isolate belongs to genotype Ib in Class I, rather than to the traditional genotype VI in Class II. To our knowledge, this study is the first to report a Class I NDV strain isolated from a pigeon. These data presented in this study provide useful information to elucidate the distribution and evolution of Class I virus for further NDV prevention.
Additional information
Funding
References
- Alexander, D.J. (2001). Gordon memorial lecture. Newcastle disease. British Poultry Science, 42, 5–22. doi: 10.1080/713655022
- Alexander, D.J., Campbell, G., Manvell, R.J., Collins, M.S., Parsons, G. & McNulty, M.S. (1992). Characterisation of an antigenically unusual virus responsible for two outbreaks of Newcastle disease in the Republic of Ireland in 1990. The Veterinary Record, 130, 65–68. doi: 10.1136/vr.130.4.65
- Chen, S., Hao, H., Liu, Q., Wang, R., Zhang, P., Wang, X., Du, E. & Yang, Z. (2013). Phylogenetic and pathogenic analyses of two virulent Newcastle disease viruses isolated from Crested Ibis (Nipponia nippon) in China. Virus Genes, 46, 447–453. doi: 10.1007/s11262-013-0881-7
- Cho, S.H., Kim, S.J. & Kwon, H.J. (2007). Genomic sequence of an antigenic variant Newcastle disease virus isolated in Korea. Virus Genes, 35, 293–302. doi: 10.1007/s11262-007-0078-z
- Choi, K.S., Kye, S.J., Kim, J.Y. & Lee, H.S. (2013). Genetic and antigenic variation of shedding viruses from vaccinated chickens after challenge with virulent Newcastle disease virus. Avian Diseases, 57, 303–306. doi: 10.1637/10379-092112-ResNote.1
- Collins, M.S., Strong, I. & Alexander, D.J. (1994). Evaluation of the molecular basis of pathogenicity of the variant Newcastle disease viruses termed “pigeon PMV-1 viruses”. Archives of Virology, 134, 403–411. doi: 10.1007/BF01310577
- Collins, M.S., Strong, I. & Alexander, D.J. (1996). Pathogenicity and phylogenetic evaluation of the variant Newcastle disease viruses termed “pigeon PMV-1 viruses” based on the nucleotide sequence of the fusion protein gene. Archives of Virology, 141, 635–647. doi: 10.1007/BF01718322
- Connaris, H., Takimoto, T., Russell, R., Crennell, S., Moustafa, I., Portner, A. & Taylor, G. (2002). Probing the sialic acid binding site of the hemagglutinin-neuraminidase of Newcastle disease virus: identification of key amino acids involved in cell binding, catalysis, and fusion. Journal of Virology, 76, 1816–1824. doi: 10.1128/JVI.76.4.1816-1824.2002
- Czegledi, A., Ujvari, D., Somogyi, E., Wehmann, E., Werner, O. & Lomniczi, B. (2006). Third genome size category of avian paramyxovirus serotype 1 (Newcastle disease virus) and evolutionary implications. Virus Research, 120, 36–48. doi: 10.1016/j.virusres.2005.11.009
- De Leeuw, O.S., Hartog, L., Koch, G. & Peeters, B.P. (2003). Effect of fusion protein cleavage site mutations on virulence of Newcastle disease virus: non-virulent cleavage site mutants revert to virulence after one passage in chicken brain. Journal of General Virology, 84, 475–484. doi: 10.1099/vir.0.18714-0
- De Oliveira Torres Carrasco, A., Seki, M.C., De Freitas Raso, T., Paulillo, A.C. & Pinto, A.A. (2008). Experimental infection of Newcastle disease virus in pigeons (Columba livia): humoral antibody response, contact transmission and viral genome shedding. Veterinary Microbiology, 129, 89–96. doi: 10.1016/j.vetmic.2007.11.012
- Diel, D.G., Da Silva, L.H., Liu, H., Wang, Z., Miller, P.J. & Afonso, C.L. (2012). Genetic diversity of avian paramyxovirus type 1: proposal for a unified nomenclature and classification system of Newcastle disease virus genotypes. Infection, Genetics and Evolution, 12, 1770–1779. doi: 10.1016/j.meegid.2012.07.012
- Dortmans, J.C., Koch, G., Rottier, P.J. & Peeters, B.P. (2009). Virulence of pigeon paramyxovirus type 1 does not always correlate with the cleavability of its fusion protein. Journal of General Virology, 90, 2746–2750. doi: 10.1099/vir.0.014118-0
- Dortmans, J.C., Rottier, P.J., Koch, G. & Peeters, B.P. (2010). The viral replication complex is associated with the virulence of Newcastle disease virus. Journal of Virology, 84, 10113–10120. doi: 10.1128/JVI.00097-10
- Dortmans, J.C., Rottier, P.J., Koch, G. & Peeters, B.P. (2011). Passaging of a Newcastle disease virus pigeon variant in chickens results in selection of viruses with mutations in the polymerase complex enhancing virus replication and virulence. Journal of General Virology, 92, 336–345. doi: 10.1099/vir.0.026344-0
- Fan, S., Wang, T., Gao, X., Ying, Y., Li, Y., Li, Y., Ma, J., Sun, H., Chu, D., Xu, Y., Yang, S., Li, Q., Gao, Y. & Xia, X. (2015). Phylogenetic analysis of Newcastle disease isolated from wild birds in the Poyang Lake region of China. Journal of Veterinary Medical Science, 77, 1143–1149. doi: 10.1292/jvms.14-0080
- Glickman, R.L., Syddall, R.J., Iorio, R.M., Sheehan, J.P. & Bratt, M.A. (1988). Quantitative basic residue requirements in the cleavage-activation site of the fusion glycoprotein as a determinant of virulence for Newcastle disease virus. Journal of Virology, 62, 354–356.
- Guo, H., Liu, X., Xu, Y., Han, Z., Shao, Y., Kong, X. & Liu, S. (2014). A comparative study of pigeons and chickens experimentally infected with PPMV-1 to determine antigenic relationships between PPMV-1 and NDV strains. Veterinary Microbiology, 168, 88–97. doi: 10.1016/j.vetmic.2013.11.002
- Hu, S., Wang, T., Liu, Y., Meng, C., Wang, X., Wu, Y. & Liu, X. (2010). Identification of a variable epitope on the Newcastle disease virus hemagglutinin-neuraminidase protein. Veterinary Microbiology, 140, 92–97. doi: 10.1016/j.vetmic.2009.07.029
- Iorio, R.M., Field, G.M., Sauvron, J.M., Mirza, A.M., Deng, R., Mahon, P.J. & Langedijk, J.P. (2001). Structural and functional relationship between the receptor recognition and neuraminidase activities of the Newcastle disease virus hemagglutinin-neuraminidase protein: receptor recognition is dependent on neuraminidase activity. Journal of Virology, 75, 1918–1927. doi: 10.1128/JVI.75.4.1918-1927.2001
- Iorio, R.M., Glickman, R.L. & Sheehan, J.P. (1992). Inhibition of fusion by neutralizing monoclonal antibodies to the haemagglutinin-neuraminidase glycoprotein of Newcastle disease virus. Journal of General Virology, 73, 1167–1176. doi: 10.1099/0022-1317-73-5-1167
- Iorio, R.M., Syddall, R.J., Sheehan, J.P., Bratt, M.A., Glickman, R.L. & Riel, A.M. (1991). Neutralization map of the hemagglutinin-neuraminidase glycoprotein of Newcastle disease virus: domains recognized by monoclonal antibodies that prevent receptor recognition. Journal of Virology, 65, 4999–5006.
- Irvine, R.M., Aldous, E.W., Manvell, R.J., Cox, W.J., Ceeraz, V., Fuller, C.M., Wood, A.M., Milne, J.C., Wilson, M., Hepple, R.G., Hurst, A., Sharpe, C.E., Alexander, D.J. & Brown, I.H. (2009). Outbreak of Newcastle disease due to pigeon paramyxovirus type 1 in grey partridges (Perdix perdix) in Scotland in October 2006. Veterinary Record, 165, 531–535. doi: 10.1136/vr.165.18.531
- Jacques, J.P., Hausmann, S. & Kolakofsky, D. (1994). Paramyxovirus mRNA editing leads to G deletions as well as insertions. The Embo Journal, 13, 5496–5503.
- Kaleta, E.F., Alexander, D.J. & Russell, P.H. (1985). The first isolation of the avian PMV-1 virus responsible for the current panzootic in pigeons? Avian Pathology, 14, 553–557. doi: 10.1080/03079458508436258
- Kim, L.M., King, D.J., Curry, P.E., Suarez, D.L., Swayne, D.E., Stallknecht, D.E., Slemons, R.D., Pedersen, J.C., Senne, D.A., Winker, K. & Afonson, C.L. (2007). Phylogenetic diversity among low-virulence Newcastle disease viruses from waterfowl and shorebirds and comparison of genotype distributions to those of poultry-origin isolates. Journal of Virology, 81, 12641–12653. doi: 10.1128/JVI.00843-07
- Kolakofsky, D., Roux, L., Garcin, D. & Ruigrok, R.W. (2005). Paramyxovirus mRNA editing, the “rule of six” and error catastrophe: a hypothesis. Journal of General Virology, 86, 1869–1877. doi: 10.1099/vir.0.80986-0
- Krishnamurthy, S. & Samal, S.K. (1998). Nucleotide sequences of the trailer, nucleocapsid protein gene and intergenic regions of Newcastle disease virus strain Beaudette C and completion of the entire genome sequence. Journal of General Virology, 79, 2419–2424. doi: 10.1099/0022-1317-79-10-2419
- Li, Z., Yu, M., Zhang, H., Wang, H.Y. & Wang, L.F. (2005). Improved rapid amplification of cDNA ends (RACE) for mapping both the 5′ and 3′ terminal sequences of paramyxovirus genomes. Journal of Virological Methods, 130, 154–156. doi: 10.1016/j.jviromet.2005.06.022
- Lindh, E., Ek-Kommonen, C., Vaananen, V.M., Alasaari, J., Vaheri, A., Vapalahti, O. & Huovilainen, A. (2012). Molecular epidemiology of outbreak-associated and wild-waterfowl-derived Newcastle disease virus strains in Finland, including a novel class I genotype. Journal of Clinical Microbiology, 50, 3664–3673. doi: 10.1128/JCM.01427-12
- Liu, H., Wang, Z., Son, C., Wang, Y., Yu, B., Zheng, D., Sun, C. & Wu, Y. (2006). Characterization of pigeon-origin Newcastle disease virus isolated in China. Avian Diseases, 50, 636–640. doi: 10.1637/7618-042606R1.1
- Liu, H., Zhang, P., Wu, P., Chen, S., Mu, G., Duan, X., Hao, H., Du, E., Wang, X. & Yang, Z. (2013). Phylogenetic characterization and virulence of two Newcastle disease viruses isolated from wild birds in China. Infection, Genetics and Evolution, 20, 215–224. doi: 10.1016/j.meegid.2013.08.021
- Maminiaina, O.F., Gil, P., Briand, F.X., Albina, E., Keita, D., Andriamanivo, H.R., Chevalier, V., Lancelot, R., Martinez, D., Rakotondravao, R., Rajaonarison, J.J., Koko, M., Andriantsimahavandy, A.A., Jestin, V. & Servan de Almeida, R. (2010). Newcastle disease virus in Madagascar: identification of an original genotype possibly deriving from a died out ancestor of genotype IV. PLoS One, 5, 13987. doi: 10.1371/journal.pone.0013987
- Mayo, M. (2002). A summary of taxonomic changes recently approved by ICTV. Archives of Virology, 147, 1655–1656. doi: 10.1007/s007050200039
- McGinnes, L.W. & Morrison, T.G. (1994). The role of the individual cysteine residues in the formation of the mature, antigenic HN protein of Newcastle disease virus. Virology, 200, 470–483. doi: 10.1006/viro.1994.1210
- McGinnes, L.W. & Morrison, T.G. (1995). The role of individual oligosaccharide chains in the activities of the HN glycoprotein of Newcastle disease virus. Virology, 212, 398–410. doi: 10.1006/viro.1995.1497
- McGinnes, L.W., Sergel, T., Reitter, J. & Morrison, T. (2001). Carbohydrate modifications of the NDV fusion protein heptad repeat domains influence maturation and fusion activity. Virology, 283, 332–342. doi: 10.1006/viro.2001.0899
- Meulemans, G., Van den Berg, T.P., Decaesstecker, M. & Boschmans, M. (2002). Evolution of pigeon Newcastle disease virus strains. Avian Pathology, 31, 515–519. doi: 10.1080/0307945021000005897
- Morrison, T.G. (2003). Structure and function of a paramyxovirus fusion protein. Biochimica et Biophysica Acta (BBA)-Biomembranes, 1614, 73–84. doi: 10.1016/S0005-2736(03)00164-0
- Nagai, Y., Klenk, H.D. & Rott, R. (1976). Proteolytic cleavage of the viral glycoproteins and its significance for the virulence of Newcastle disease virus. Virology, 72, 494–508. doi: 10.1016/0042-6822(76)90178-1
- OIE (Office International des Epizooties). (2008). Newcastle disease. In Manual of Diagnostic Tests and Vaccines for Terrestrial Animals (pp. 576–589). Part 2, Section 2.3, Chapter 2.3.14. Paris: OIE.
- Pitt, J.J., Da Silva, E. & Gorman, J.J. (2000). Determination of the disulfide bond arrangement of Newcastle disease virus hemagglutinin neraminidase correlation with a beta-sheet propeller structural fold predicted for paramyxoviridae attachment proteins. The Journal of Biological Chemistry, 275, 6469–6478. doi: 10.1074/jbc.275.9.6469
- Romer-Oberdorfer, A., Werner, O., Veits, J., Mebatsion, T. & Mettenleiter, T.C. (2003). Contribution of the length of the HN protein and the sequence of the F protein cleavage site to Newcastle disease virus pathogenicity. Journal of General Virology, 84, 3121–3129. doi: 10.1099/vir.0.19416-0
- Ronin, C., Bouchilloux, S., Granier, C. & van Rietschoten, J. (1978). Enzymatic N-glycosylation of synthetic Asn--X--Thr containing peptides. FEBS Letters, 96, 179–182. doi: 10.1016/0014-5793(78)81089-8
- Sakaguchi, T., Toyoda, T., Gotoh, B., Inocencio, N.M., Kuma, K., Miyata, T. & Nagai, Y. (1989). Newcastle disease virus evolution. I. Multiple lineages defined by sequence variability of the hemagglutinin-neuraminidase gene. Virology, 169, 260–272. doi: 10.1016/0042-6822(89)90151-7
- Smietanka, K., Olszewska, M., Domanska-Blicharz, K., Bocian, A.L. & Minta, Z. (2014). Experimental infection of different species of birds with pigeon paramyxovirus type 1 virus-evaluation of clinical outcomes, viral shedding, and distribution in tissues. Avian Diseases, 58, 523–530. doi: 10.1637/10769-011514-Reg.1
- Snoeck, C.J., Owoade, A.A., Couacy-Hymann, E., Alkali, B.R., Okwen, M.P., Adeyanju, A.T., Komoyo, G.F., Nakoune, E., Le, Faou, A. & Muller, C.P. (2013). High genetic diversity of Newcastle disease virus in poultry in West and Central Africa: cocirculation of genotype XIV and newly defined genotypes XVII and XVIII. Journal of Clinical Microbiology, 51, 2250–2260. doi: 10.1128/JCM.00684-13
- Steward, M., Vipond, I.B., Millar, N.S. & Emmerson, P.T. (1993). RNA editing in Newcastle disease virus. Journal of General Virology, 74, 2539–2547. doi: 10.1099/0022-1317-74-12-2539
- Stone-Hulslander, J. & Morrison, T.G. (1999). Mutational analysis of heptad repeats in the membrane-proximal region of Newcastle disease virus HN protein. Journal of Virology, 73, 3630–3637.
- Takimoto, T., Taylor, G.L., Connaris, H.C., Crennell, S.J. & Portner, A. (2002). Role of the hemagglutinin-neuraminidase protein in the mechanism of paramyxovirus-cell membrane fusion. Journal of Virology, 76, 13028–13033. doi: 10.1128/JVI.76.24.13028-13033.2002
- Tamura, K., Peterson, D., Peterson, N., Stecher, G., Nei, M. & Kumar, S. (2011). MEGA5: molecular evolutionary genetics anaylsis using maximum likelihood, evolutionary distance, and maximum parsimony methods. Molecular Biology and Evolution, 28, 2731–2739. doi: 10.1093/molbev/msr121
- Toyoda, T., Gotoh, B., Sakaguchi, T., Kida, H. & Nagai, Y. (1988). Identification of amino acids relevant to three antigenic determinants on the fusion protein of Newcastle disease virus that are involved in fusion inhibition and neutralization. Journal of Virology, 62, 4427–4430.
- Ujvari, D., Wehmann, E., Herczeg, J. & Lomniczi, B. (2006). Identification and subgrouping of pigeon type Newcastle disease virus strains by restriction enzyme cleavage site analysis. Journal of Virological Methods, 131, 115–121. doi: 10.1016/j.jviromet.2005.07.012
- Umali, D.V., Ito, H., Shirota, K., Katoh, H. & Ito, T. (2014). Charaterization of complete genome sequence of genotype VI and VII velogenic Newcastle disease virus from Japan. Virus Genes, 49, 89–99. doi: 10.1007/s11262-014-1075-7
- White, J.M., Delos, S.E., Brecher, M. & Schornberg, K. (2008). Structures and mechanisms of viral membrane fusion proteins: multiple variations on a common theme. Critical Reviews in Biochemistry and Molecular Biology, 43, 189–219. doi: 10.1080/10409230802058320
- Wu, W., Liu, H., Zhang, T., Han, Z., Jiang, Y., Xu, Q., Shao, Y., Li, H., Kong, X., Chen, H. & Liu, S. (2015). Molecular and antigenic characteristics of Newcastle disease virus isolates from domestic ducks in China. Infection, Genetics and Evolution, 32, 34–43. doi: 10.1016/j.meegid.2015.02.016
- Yosoff, K., Nesbit, M., McCartney, H., Meulemans, G., Alexander, D.J., Collins, M.S., Emmerson, P.T. & Samson, A.C. (1989). Location of neutralizing eptitopes on the fusion protein of Newcastle disease virus strain Beaudette C. Journal of General Virology, 70, 3105–3109. doi: 10.1099/0022-1317-70-11-3105