ABSTRACT
Antimicrobial resistance is recognized as one of the most important global health challenges. Broilers are an important reservoir of antimicrobial resistant bacteria in general and, more particularly, extended-spectrum β-lactamases (ESBL)/AmpC-producing Enterobacteriaceae. Since contamination of 1-day-old chicks is a potential risk factor for the introduction of antimicrobial resistant Enterobacteriaceae in the broiler production chain, the presence of antimicrobial resistant coliform bacteria in broiler hatching eggs was explored in the present study. Samples from 186 hatching eggs, collected from 11 broiler breeder farms, were inoculated on MacConkey agar with or without ceftiofur and investigated for the presence of antimicrobial resistant lactose-positive Enterobacteriaceae, particularly, ESBL/AmpC-producers. Escherichia coli and Enterobacter cloacae were obtained from the eggshells in 10 out of 11 (10/11) sampled farms. The majority of the isolates were recovered from crushed eggshells after external decontamination suggesting that these bacteria are concealed from the disinfectants in the egg shell pores. Antimicrobial resistance testing revealed that approximately 30% of the isolates showed resistance to ampicillin, tetracycline, trimethoprim and sulphonamides, while the majority of isolates were susceptible to amoxicillin-clavulanic acid, nitrofurantoin, aminoglycosides, florfenicol, neomycin and apramycin. Resistance to extended-spectrum cephalosporins was detected in eight Enterobacteriaceae isolates from five different broiler breeder farms. The ESBL phenotype was confirmed by the double disk synergy test and blaSHV–12, blaTEM–52 and blaACT–39 resistance genes were detected by PCR. This report is the first to present broiler hatching eggs as carriers and a potential source of ESBL/AmpC-producing Enterobacteriaceae for broiler chicks.
Introduction
Antimicrobial resistance is recognized as one of the most important global health challenges. The introduction of extended-spectrum cephalosporins (ESCs) improved the treatment options in both veterinary and human medicine (Pfeifer et al., Citation2010). However, resistance to these antimicrobials, mainly conferred by extended-spectrum β-lactamases (ESBL) and AmpC β-lactamases, has recently gained importance and is considered as an emerging public health concern (Reich et al., Citation2013). ESBL- and AmpC-producing Enterobacteriaceae were first associated with hospitals and institutional care centres in humans, but are now increasingly present in the community (Livermore et al., Citation2007), in food-producing animals (Dierikx et al., Citation2013a), and retail meat (Kola et al., Citation2012), suggesting organism or gene exchange between different reservoirs (Leverstein-van Hall et al., Citation2011).
Several studies have reported that the gastrointestinal tract of healthy broilers (Smet et al., Citation2008) and layer hens (Chauvin et al., Citation2013) may be an important reservoir for antimicrobial-resistant bacteria in general and, more particularly, ESBL/AmpC-producers. These birds represent a potential risk for public health, particularly via broiler meat (Kola et al., Citation2012) or table eggs (Rasheed et al., Citation2014).
The spread of antimicrobial-resistant bacteria from broiler parent birds to broilers and subsequently to chicken meat has been recently shown (Dierikx et al., Citation2013b). A number of factors are incriminated in the introduction of antimicrobial-resistant bacteria to the broiler production chain (Hiroi et al., Citation2012; Schwaiger et al., Citation2013). Faeces, litter and even dust may act as transmission sources of ESBL/AmpC-producing bacteria (Laube et al., Citation2014). One-day-old chicks are also shown to be a major risk factor for the introduction of ESBL/AmpC-producing Escherichia coli in the broiler production chain (Dierikx et al., Citation2013b).
Breeder flocks and commercial hatcheries could be the first steps in the contamination of 1-day-old chicks by ESBL/AmpC-producing bacteria. However, to the best of our knowledge, no previously published data demonstrated the origin of this contamination. In this context, understanding the role of broiler hatching eggs in the carriage of resistant bacteria is of fundamental importance.
In the current experiments, we therefore investigated the occurrence of antimicrobial-resistant, more specifically, ESC-resistant, lactose-positive Enterobacteriaceae in broiler hatching eggs and characterized their susceptibility to specific antimicrobial agents.
Materials and methods
Egg sampling
The eggshell surface, the crushed decontaminated eggshell and the egg contents of 186 broiler hatching eggs that were not disinfected on-site, collected from 11 broiler breeder farms (nine from Belgium, one from the Netherlands, and one from France), were examined individually as described by Jones et al. (Citation2002) and Chousalkar et al. (Citation2010), with slight modifications.
In short, each intact egg was submerged in 50 ml of nutrient broth No. 2 (Oxoid, UK) for 10 min in a sterile container. After removing the egg and shaking the nutrient broth, 10 ml was transferred to a sterile 15 ml Falcon tube and incubated aerobically overnight at 37°C without shaking.
For the isolation of bacteria from eggshell pores, the eggs were first dipped in a 75% ethanol solution for 5 min to eliminate any bacteria present on the surface of the shell, and allowed to air dry in a biosafety cabinet for 5–10 min. Each egg was, then, cracked open with a sterile implement into a sterile container. The inside of the eggshells was washed with sterile phosphate-buffered saline to remove the adhering egg albumen. Egg shell and shell membranes were transferred to a 50 ml Falcon tube and crushed with a sterile laboratory tool. Twenty-five millilitres of nutrient broth No. 2 (Oxoid) was added to the 50 ml Falcon tube. The tubes were then incubated overnight at 37°C, without shaking.
The egg contents collected in the sterile containers were aseptically mixed and 3 ml of internal egg content was inoculated with 7 ml of nutrient broth No. 2 (Oxoid) and incubated overnight at 37°C, without shaking, for further processing.
Enterobacteriaceae isolation
After overnight incubation, 10 µl of each sample (egg surface, eggshell crushed after disinfection, and egg content), was plated out on MacConkey agar No. 3 (Oxoid). After overnight aerobic incubation at 37°C, from each sample, one lactose-positive colony was identified and purified for further identification and susceptibility testing.
In addition, 200 µl of each sample (egg surface, eggshell crushed after disinfection, and egg content), was plated out on MacConkey agar No. 3 (Oxoid) supplemented with 8 µg ml−1 ceftiofur (CFTIO). After overnight aerobic incubation at 37°C, all presumed Enterobacteriaceae were purified for further identification, susceptibility testing and ESBL/AmpC characterization.
Bacterial identification
Strains were provisionally identified using standard biochemical methods (Markey et al., Citation2013). The specific tests used were: glucose/lactose fermentation, gas production and H2S production on Kligler iron agar (Oxoid), aesculin hydrolysis (bile aesculin agar; Oxoid); motility, indole production and the ornithine degradation on motility-indol-ornithine media (Oxoid) (Callens et al., Citation2015). Final identification was obtained using matrix-assisted laser desorption ionization-time of flight mass spectrometry (MALDI-TOF MS); a Bruker Daltonics Biotyper instrument on ethanol-washed cell pellets extracted with formic acid and acetontirile solutions was used as recommended by the manufacturer (Bruker Daltonics Inc., Billerica, MA, USA).
Isolates confirmed as being Escherichia coli were serotyped by agglutination in micro-titre plates with monospecific antisera towards 24 different somatic O antigens: O1, O2, O5, O6, O8, O9, O11, O12, O14, O15, O17, O18, O20, O35, O36, O45, O53, O78, O81, O83, O102, O103, O115, O116, purchased from the E. coli Reference Laboratory of the University of Santiago de Compostela (Lugo, Spain), according to the procedure of Blanco et al. (Citation1996).
Antimicrobial susceptibility testing
Antimicrobial susceptibility testing was carried out on all obtained isolates by the Kirby Bauer disk diffusion test using the Neo-Sensitabs tablets (Rosco, Diagnostica, Taastrup, Denmark) with interpretation of inhibition zones according to the veterinary breakpoints, as described by the manufacturer. Briefly, after standardization of the inoculum in phosphate-buffered saline to 0.5 McFarland (Densimat; Biomérieux, Marcy l’Etoile, France), the inoculum was triple streaked (60° rotation of the round Petri dishes within streaks) on Mueller–Hinton II agar (Oxoid), and antimicrobial tablets were then brought onto the medium by means of a dispenser within 15 min after inoculation and were immediately aerobically incubated for 16–20 h at 35°C. E. coli ATCC® 25922 and E. coli ATCC® 35218 were used as internal quality control strains.
The following antimicrobials were tested: ampicillin (AM, 10 µg), amoxicillin-clavulanic acid (AMC, 20/10 µg), CFTIO, 30 µg, florfenicol (FFC, 30 µg), flumequine (FLUM, 30 µg), apramycin (APRA, 40 µg), enrofloxacin (ENRO, 10 µg), tetracycline (TET, 30 µg), gentamicin (GEN, 10 µg), neomycin (NEO, 120 µg), spectinomycin (SPECT, 200 µg), nitrofurantoine (200 µg), sulphonamides (SULF, 240 µg) and trimethoprim (TR, 5 µg) (Neo-Sensitabs; Rosco Diagnostica, Taastrup, Denmark).
Isolates resistant to CFTIO (≤17 mm) were screened for ESBL production and AmpC production using the modified double disk synergy-tests (Jarlier et al., Citation1988), and for susceptibility to imipenem (IMI, 15 µg). For the double disk synergy-tests, disks containing CFTIO (30 µg) and cefquinome (30 µg) were used at a distance of 20 mm of a disk with AMC (20/10 µg). In addition, a cefoxitin disk (30 µg) was added to this panel to detect the AmpC phenotype. Isolates resistant to AMC (≤27 mm) and classified as intermediate or resistant to cefoxitin (≤17 mm) were suspected to be AmpC-producers (Dierikx et al., Citation2012).
Detection of β-lactamase genes
DNA was extracted from all phenotypically suspected ESBL/AmpC-producing Enterobacteriaceae by inoculating a single colony of each isolate from a blood agar plate into1 ml of nutrient broth. After overnight aerobic incubation at 37°C, cells were harvested by centrifugation at 875g for 5 min. The pellet was resuspended in 1 ml of HPLC water, and cells were lysed by heating at 95°C for 5 min. Cellular debris was removed by centrifugation at 15,000g for 5 min. PCRs for the detection of genes encoding TEM-, SHV-, CTX-M-, OXA-1, DHA-, ACC-, ACT-, MIR-, MOX-, FOX- and CMY-type enzymes were carried out as previously described (Pérez-Pérez & Hanson, Citation2002; Eckert et al., Citation2004; Dierikx et al., Citation2012).
Briefly, PCR was performed with a final volume of 25 µl. The primers used for amplification are listed in Table S1 (presented in supplementary data). The primer pair denoted as ACT-21F (5′-ATGATGAAAAAATCTCTTTGC-3′) and ACT-21R (5′-TTACTGTAGCGCGTCGAGGATA-3′) was designed from the alignment of the reference sequence for the bla ACT-21 of Enterobacter hormaechei obtained from GenBank database (http://www.ncbi.nlm.nih.gov) (Genbank access number: KF526118).
Each mixture contained 12.5 µl PCR master mix (BioMix™, Bioline), 1.5 µl of each primer (10 µM, Integrated DNA Technologies), 7.5 µl of HPLC water and 2 µl template DNA. Strains B4-25, B4-75, B5-33, B1-54, B4-16 containing genes encoding CTX-M-2, CTX-M-1, CTX-M-9, TEM-52, SHV-12/CMY-2, respectively, were used as positive controls (Smet et al., Citation2008). Subsequently, the PCR amplicons were sequenced with primers designed to flank the entire bla gene (Table S1, presented in Supplementary data). Finally, the obtained nucleotide sequences were compared with BLASTN to previously described bla genes stored in GenBank (http://www.ncbi.nlm.nih.gov/BLAST/).
Disinfectant susceptibility testing
Three agents commonly used for egg disinfection in hatcheries were selected for minimum inhibitory concentration (MIC) determination: formaldehyde (FOR, 39% wt/vol in H2O), glutaraldehyde (GLU, 50% wt/vol in H2O) and hydrogen peroxide (H2O2, 31.1% wt/vol in H2O). FOR was obtained from VWR international BVBA/SPRL (Leuven, Belgium), GLU was obtained from Fluka AG (Chemische Fabrik, Ch-9470 Buchs) and the H2O2 from Merck Schuchardt OHG (Hohenbrunn, Germany).
The MICs for all obtained isolates were determined with the broth microdilution assay according to the Clinical and Laboratory Standards Institute (Citation2013) standards, as previously described (Oosterik et al., Citation2014). Twofold dilutions of the disinfectants were added to test tubes with cation-adjusted Mueller–Hinton broth (Oxoid N.V.) after which 50 µl of the disinfectant dilution was transferred to the wells of a sterile plastic 96-well microdilution plate. Bacterial suspensions were adjusted to a concentration of 106 CFU/ml. Fifty microliters of these suspensions were added to the wells of the microdilution plate, yielding a total suspension volume of 100 µl. The plates were incubated aerobically for 24 h at 35 ± 2°C and MICs were read afterwards. The MIC was defined as the lowest concentration of disinfectant where no visible growth of the isolates could be seen. E. coli ATCC® 25922 was used as quality control strain.
Statistical analysis
Chi-square test was performed to compare the prevalence of the coliform isolates in the tested hatching broiler eggs and the percentages of the total obtained isolates from eggshell surface and crushed eggshell. Differences were considered significant at P < 0.05. All statistical analyses were performed with the R software (R.2.8.0).
Results
Coliform isolates, E. coli serotyping, and antimicrobial susceptibility
Out of 186 broiler hatching eggs collected from 11 breeder farms, 30 presumptive lactose-positive colonies were isolated from the non-supplemented MacConkey agar. E. coli was most frequently identified (n = 27) followed by Enterobacter cloacae (n = 3) (P < 0.05). The isolates were obtained from 16% (30/186) of the tested eggs: 12% (22/186) were isolated from the crushed decontaminated eggshell whereas only 4% (8/186) were isolated from the eggshell surface (P < 0.05). No lactose-positive Enterobacteriaceae were isolated from any of the egg contents ().
Table 1. Prevalence of lactose-positive Enterobacteriaceae isolates from broiler hatching eggs by source.
From 27 E. coli isolates, 18 could not be serotyped with the currently used antisera. From the typed isolates, the most prevalent serogroup was O2 (n = 5), followed by O8 (n = 3) and O1 (n = 1).
All coliform (30) isolates were tested for antimicrobial susceptibility. A summary of the results of disk diffusion tests is shown in . Resistance to FFC, NEO, SPECT and APRA was not observed. Resistance to CFTIO, AMC, FLUM, GEN, nitrofurantoin and ENRO was low and ranged between 3.3% and 13.3%. Resistance rates to TR, TET, AM and sulphonamides was moderate and ranged between 26.7% and 33.3%.
Table 2. Antimicrobial susceptibility percentage with numbers in parenthesis of lactose-positive Enterobacteriaceae isolates from broiler hatching eggs.
ESC-resistant Enterobacteriaceae isolates
Eight (8) isolates resistant to ESC were obtained. Six isolates were obtained using the CFTIO supplemented medium, while two isolates were obtained using a non-supplemented MacConkey agar. The ESC resistant isolates were recovered from 5 of 11 analyzed farms and from 7/186 (3.8%) of the total tested eggs; one egg carried two isolates showing distinct antimicrobial resistance profiles. The ESC-resistant isolates were mainly obtained from the crushed decontaminated eggshell, while only one was isolated from the eggshell surface and none from the egg contents (). Out of the eight ESC-resistant isolates, seven isolates were identified as E. cloacae and one as Escherichia fergusonii. Six of eight CFTIO-resistant Enterobacteriaceae isolates (S47, S08R, S01, S02R, S20R, and S39R) were also resistant to the fourth generation cephalosporins (cefquinome) and some of the other tested non-β-lactam antimicrobial agents. No imipenem resistance was detected ().
Table 3. Genetic and phenotypic traits of CFTIO-resistant Enterobacteriaceae obtained from broiler hatching eggs.
The PCR and sequencing results are listed in . Three E. cloacae isolates (S47, S01 and S02R) carried a blaTEM–1 and blaSHV–12 gene while blaTEM–52 was only detected in E. fergusonii isolate S20R. Five of the seven E. cloacae isolates (S12R, S14R, S01, S02R and S39R) harboured an ACT-39 enzyme (GenBank accession number: KU884289. Additionally, none of the screened β-lactamase genes could be found in S08R ().
Disinfectant MICs
The MICs determined by broth microdilution assay related to the 36 obtained isolates are presented in .
Figure 1. (a) MIC of the recovered isolates from broiler hatching eggs determined for GLU per genus. (b) MIC of the recovered isolates from broiler hatching eggs determined for H2O2 per genus. (c) MIC of the recovered isolates from broiler hatching eggs determined for FOR per genus.
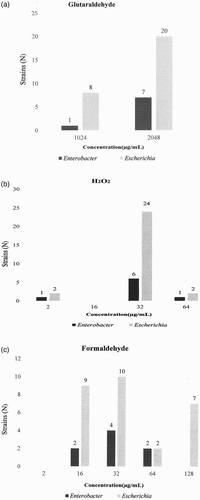
The ranges of MIC values of the retrieved isolates and quality control strains were similar to MIC ranges described earlier (Oosterik et al., Citation2014). Since no wild type cut-off values are yet available for disinfectants, acquired resistance was assumed when MIC values showed a bimodal, multimodal or tailing distribution. The distribution of the MIC values for GLU showed a unimodal distribution ((a)). The distribution of the MIC values for H2O2 showed a bimodal distribution with the majority of isolates showing MIC values of 32–64 µg ml−1 and three isolates showing a MIC value of 2 µg ml−1 ((b)). Considering previously published data (Oosterik et al., Citation2014), the isolates showing MIC values of 32–64 µg ml−1 are probably wild type strains, while the isolates showing a MIC value of 2 µg ml−1 are probably isolates with increased susceptibility. It was therefore concluded that there was no acquired resistance towards both GLU and H2O2. The MICs of FOR show a monomodal distribution, with possibly tailing towards the higher MIC values which might suggest low level acquired resistance in isolates with a MIC of 128 µg ml−1 ((c)).
Discussion
As previously published, a well-documented case report suggested that parental birds may represent an important reservoir of pathogenic E. coli strains and subsequent colibacillosis in newly hatched broiler chicks (Petersen et al., Citation2006). In the current screening of 186 eggs E. coli isolates belonged to serotypes O2, O8 and O1, known to be associated with avian colibacillosis (Schouler et al., Citation2012).
Using coliforms and ESBL-producing Enterobacteriaceae as indicator bacteria for antimicrobial resistance, strains were isolated from hatching eggs from almost all sampled broiler breeder farms (10/11). These results show that hatching eggs could be a potential source of transmission of antimicrobial-resistant bacteria or antimicrobial resistance genes in the broiler production chain. Indeed, it has been shown by Mevius et al. (Citation2009) and Dierikx et al. (Citation2013b) that 1-day-old chicks are often colonized by ESBL/AmpC-producing Enterobacteriaceae.
Antimicrobial resistance towards antimicrobial classes frequently used in veterinary medicine was tested on 30 lactose-positive Enterobacteriaceae isolates. Approximately one third of these isolates showed resistance to AM, TET, TR and sulphonamides. On the contrary, most isolates were susceptible to CFTIO, AMC, nitrofurantoin, the aminoglycosides, FFC, NEO and APRA. The presence of a small percentage of fluoroquinolone resistant Enterobacteriaceae isolates is noteworthy, but not entirely surprising considering the frequent detection of quinolone resistance in Enterobacteriaceae isolated from poultry (Vanni et al., Citation2014). SPECT was the only antimicrobial agent against which 100% susceptibility was detected. These results are in concert with data from several previous studies revealing that resistance to aminopenicillins, TETs and sulphonamides is common among E. coli strains isolated from poultry (Persoons et al., Citation2010) or commercial table eggs (Musgrove et al., Citation2006).
Resistance to ESCs, recovered in eight Enterobacteriaceae isolates, was found in five of the 11 analyzed farms. To the best of our knowledge, this report is the first to describe broiler hatching eggs as carriers and therefore as a potential source of ESBL-producing Enterobacteriaceae in broiler chicks. Previous research in laying hens (Schwaiger et al., Citation2008) or commercial table eggs (Musgrove et al., Citation2006) all reported E. coli isolates susceptible to ESCs. Molecular characterization of the ESC-resistant isolates showed that the resistance determinants were conferred by mobile elements and therefore could easily be horizontally transferred between strains, species or even genera (de Been et al., Citation2014). Half of these isolates harboured genes encoding ESBLs (SHV-12 and TEM-52) and most of E. cloacae isolates (5/7) harboured blaACT–39, a new variant of the ACT AmpC β-lactamase. SHV-12 and TEM-52 are among the most frequently detected ESBLs in Enterobacteriaceae responsible for infections in humans (Coque et al., Citation2008).
A relevant finding is the fact that the Enterobacteriaceae were mainly isolated from crushed eggshells even after extensive external decontamination. This could be related to the particular constitution of the eggshell itself, making the bacteria unreachable by the disinfection agents in the egg shell pores. The obtained Enterobacteriaceae isolates were probably concealed in the pores of the egg shells from the disinfection step. This is in agreement with previously reported results showing that the shell and membrane crush method recovers microorganisms from the pores and membranes that are missed by swabbing or rinsing methods (Musgrove et al., Citation2005). The bacterial contamination of the egg could occur while passing through the cloaca, or immediately after lay from the breeding and hatching environment, especially during the first 30–60 s, when the eggs are most vulnerable to bacterial penetration before the cuticle hardens and effectively caps the pores (Montgomery et al., Citation1999).
It is also possible that acquired resistance against disinfecting agents could lead to persistence of bacteria in or on egg shells after decontamination. However, in the current investigations, none of the investigated isolates showed clear features of acquired resistance to the three commonly used disinfectants in hatcheries. In addition, commercial disinfectants are often a mixture of active compounds, thereby hampering the selection of acquired resistance (Jones et al., Citation2000).
The phenomenon of bacterial concealment in the shell pores may be of importance in the field; (antimicrobial-resistant) bacteria that survive this process may colonize 1-day-old chicks and spread throughout the hatchery.
In conclusion, the present results revealed that broiler hatching eggs can be carriers of broad-spectrum β-lactamase-producing Enterobacteriaceae. The origin of this contamination remains speculative and further studies are warranted. The phenomenon of bacteria concealment revealed in the current results increases the hatching eggs as a potential factor in antibiotic resistance dissemination.
Supplemental data
Download MS Word (64.5 KB)Acknowledgements
The authors would like to thank Dr P. Wattiau, Coda-Cerva Brussels, Belgium for the identification of all isolates by MALDI-TOF MS and the serotyping of the E. coli isolates.
Disclosure statement
No potential conflict of interest was reported by the authors.
ORCID
F. Haesebrouck http://orcid.org/0000-0002-1709-933X
Additional information
Funding
References
- de Been, M., Lanza, V.F., de Toro, M., Scharringa, J., Dohmen, W., Du, Y., Hu, J., Lei, Y., Li, N., Tooming-Klunderud, A., Heederik, D. J., Fluit, A. C., Bonten, M.J., Willems, R.J., de la Cruz, F. & van Schaik, W. (2014). Dissemination of cephalosporin resistance genes between Escherichia coli strains from farm animals and humans by specific plasmid lineages. PLoS Genetics, 10, e1004776. doi: 10.1371/journal.pgen.1004776
- Blanco, J.E., Blanco, M., Blanco, J., Mora, A., Balaguer, L., Mouriňo, J. & Jansen, W.H. (1996). O serogroups, biotypes and eae genes in Escherichia coli strains isolated from diarrheic and healthy rabbits. Journal of Clinical Microbiology, 34, 3101–3107.
- Callens, B., Faes, C., Maes, D., Catry, B., Boyen, F., Francoys, D., de Jong, E., Haesebrouck, F. & Dewulf, J. (2015). Presence of antimicrobial resistance and antimicrobial use in sows are risk factors for antimicrobial resistance in their offspring. Microbial Drug Resistance, 21, 50–58. doi: 10.1089/mdr.2014.0037
- Chauvin, C., Le Devendec, L., Jouy, E., Le Cornec, M., Francart, S., Marois-Créhan, C. & Kempf, I. (2013). National prevalence of resistance to third-generation cephalosporins in Escherichia coli isolates from layer flocks in France. Antimicrobial Agents and Chemotherapy, 57, 6351–6353. doi: 10.1128/AAC.01460-13
- Chousalkar, K.K., Flynn, P., Sutherland, M., Roberts, J.R. & Cheetham, B.F. (2010). Recovery of Salmonella and Escherichia coli from commercial egg shells and effect of translucency on bacterial penetration in eggs. International Journal of Food Microbiology, 142, 207–213. doi: 10.1016/j.ijfoodmicro.2010.06.029
- Clinical and Laboratory Standards Institute. (2013). Performance standards for antimicrobial disk and dilution susceptibility tests for bacteria isolated from animals; approved standard. 4th ed. Supplements Vet01-A4. Wayne, PA: Clinical and Laboratory Standards Institute.
- Coque, T.M., Baquero, F. & Canton, R. (2008). Increasing prevalence of ESBL-producing Enterobacteriaceae in Europe. Euro Surveillance, 13, 5437–5453.
- Dierikx, C.M., van Duijkeren, E., Schoormans, A.H., van Essen-Zandbergen, A., Veldman, K., Kant, A., Huijsdens, X.W., van der Zwaluw, K., Wagenaar, J.A. & Mevius, D.J. (2012). Occurrence and characteristics of extended-spectrum-β-lactamase- and AmpC-producing clinical isolates derived from companion animals and horses. Journal of Antimicrobial Chemotherapy, 67, 1368–1374. doi: 10.1093/jac/dks049
- Dierikx, C.M., van der Goot, J., Fabri, T., van Essen-Zandbergen, A., Smith, H. & Mevius, D.J. (2013a). Extended-spectrum-β-lactamase- and AmpC-β-lactamase-producing Escherichia coli in Dutch broilers and broiler farmers. Journal of Antimicrobial Chemotherapy, 68, 60–67. doi: 10.1093/jac/dks349
- Dierikx, C.M., van der Goot, J.A., Smith, H.E., Kant, A. & Mevius, D. J. (2013b). Presence of ESBL/AmpC-producing Escherichia coli in the broiler production pyramid: a descriptive study. PLoS One, 8, e79005. doi: 10.1371/journal.pone.0079005
- Eckert, C., Gauthier, V., Saladin-Allard, M. Hidri, N., Verdet, C., Ould-Hocine, Z., Barnaud, G., Delisle, F., Rossier, A., Lambert, T., Philippon, A. & Arlet, G. (2004). Dissemination of CTX-M-type β-lactamases among clinical isolates of Enterobacteriaceae in Paris, France. Antimicrobial Agents and Chemotherapy, 48, 1249–1255. doi: 10.1128/AAC.48.4.1249-1255.2004
- Hiroi, M., Matsui, S., Kubo, R., Iida, N., Noda, Y., Kanda, T., Sugiyama, K., Hara-Kudo, Y. & Ohashi, N. (2012). Factors for occurrence of extended spectrum beta-lactamase-producing Escherichia coli in broilers. The Journal of Veterinary Medical Science, 74, 1635–1637. doi: 10.1292/jvms.11-0479
- Jarlier, V., Nicolas, M.H., Fournier, G. & Philippon A. (1988). Extended broad-spectrum β-lactamases conferring transferable resistance to newer β-lactam agents in enterobacteriaceae: hospital prevalence and susceptibility patterns. Clinical Infectious Diseases, 10, 867–878. doi: 10.1093/clinids/10.4.867
- Jones, D.R., Anderson, K.E., Curtis, P.A. & Jones, F.T. (2002). Microbial contamination in inoculated shell eggs: I. Effects of layer strain and hen age. Poultry Science, 81, 715–720. doi: 10.1093/ps/81.5.715
- Jones, D.R., Jampani, H.B., Newman, J.L. & Lee, A.S. (2000). Triclosan: a review of effectiveness and safety in health care settings. American Journal of Infection Control, 28, 184–196. doi: 10.1067/mic.2000.102378
- Kola, A., Kohler, C., Pfeifer, Y., Schwab, F., Kühn, K., Schulz, K., Balau, V., Breitbach, K., Bast, A., Witte, W., Gastmeier, P. & Steinmetz, I. (2012). High prevalence of extended-spectrum-β-lactamase-producing Enterobacteriaceae in organic and conventional retail chicken meat, Germany. Journal of Antimicrobial Chemotherapy, 67, 2631–2634. doi: 10.1093/jac/dks295
- Laube, H., Friese, A., von Salviati, C., Guerra, B. & Rösler, U. (2014). Transmission of ESBL/AmpC-producing Escherichia coli from broiler chicken farms to surrounding areas. Veterinary Microbiology, 172, 519–527. doi: 10.1016/j.vetmic.2014.06.008
- Leverstein-van Hall, M.A., Dierikx, C.M., Cohen Stuart, J., Voets, G.M., van den Munckhof, M.P., van Essen-Zandbergen, A., Platteel, T., Fluit, A. C., van de Sande-Bruinsma, N., Scharinga, J., Bonten, M.J., Mevius, D.J. & National ESBL Surveillance Group. (2011). Dutch patients, retail chicken meat and poultry share the same ESBL genes, plasmids and strains. Clinical Microbiology and Infection, 17, 873–880. doi: 10.1111/j.1469-0691.2011.03497.x
- Livermore, D.M., Canton, R., Gniadkowski, M., Nordmann, P., Rossolini, G.M., Arlet, G., Ayala, J., Coque, T.M., Kern-Zdanowicz, I., Luzzaro, F., Poirel, L. & Woodford, N. (2007). CTX-M: changing the face of ESBLs in Europe. Journal of Antimicrobial Chemotherapy, 59, 165–174. doi: 10.1093/jac/dkl483
- Markey, B.K., Leonard, F. C., Archambault, M., Cullinane, A. & Maguire, D. (2013). Differentiation of the Enterobacteriaceae. In Clinical Veterinary Microbiology (2nd ed., pp. 239–254). Mosby: Edinburgh.
- Mevius, D.J.K., Koene, M.G.J., Witt, B., van Pelt, W. & Bondt, W. (2009). Monitoring of antimicrobial resistance and antibiotic usage in animals in the Netherlands in 2009. Wageningen: Central Veterinary Institute of Wageningen University and Research Centre.
- Montgomery, R.D., Boyle, C.R., Lenarduzzi, T.A. & Jones, L.S. (1999). Consequences to chicks hatched from Escherichia coli-inoculated embryos. Avian Diseases, 43, 553–563. doi: 10.2307/1592656
- Musgrove, M.T., Jones, D.R., Northcutt, J.K., Cox, N.A. & Harrison, M.A. (2005). Shell rinse and shell crush methods for the recovery of aerobic microorganisms and Enterobacteriaceae from shell eggs. Journal of Food Protection, 68, 2144–2148.
- Musgrove, M.T., Jones, D.R., Northcutt, J.K., Cox, N.A., Harrison, M.A., Fedorka-Cray, P.J. & Ladely, S.R. (2006). Antimicrobial resistance in Salmonella and Escherichia coli isolated from commercial shell eggs. Poultry Science, 85, 1665–1669. doi: 10.1093/ps/85.9.1665
- Oosterik, L.H., Peeters, L., Mutuku, I., Goddeeris, B.M. & Butaye, P. (2014). Susceptibility of avian pathogenic Escherichia coli from laying hens in belgium to antibiotics and disinfectants and integron prevalence. Avian Diseases, 58, 271–278. doi: 10.1637/10680-100113-RegR
- Pérez-Pérez, F.J. & Hanson, N.D. (2002). Detection of plasmid-mediated AmpC β-lactamase genes in clinical isolates by using multiplex PCR. Journal of Clinical Microbiology, 40, 2153–2162. doi: 10.1128/JCM.40.6.2153-2162.2002
- Persoons, D., Dewulf, J., Smet, A., Herman, L., Heyndrickx, M., Martel, A., Catry, B., Butaye, P. & Haesebrouck, F. (2010). Prevalence and persistence of antimicrobial resistance in broiler indicator bacteria. Microbial Drug Resistance, 16, 67–74. doi: 10.1089/mdr.2009.0062
- Petersen, A., Christensen, J.P., Kuhnert, P., Bisgaard, M. & Olsen, J.E. (2006). Vertical transmission of a fluoroquinolone-resistant Escherichia coli within an integrated broiler operation. Veterinary Microbiology, 116, 120–128. doi: 10.1016/j.vetmic.2006.03.015
- Pfeifer, Y., Cullik, A. & Witte, W. (2010). Resistance to cephalosporins and carbapenems in Gram-negative bacterial pathogens. International Journal of Medical Microbiology, 300, 371–379. doi: 10.1016/j.ijmm.2010.04.005
- Rasheed, M.U., Thajuddin, N., Ahamed, P., Teklemariam, Z. & Jamil, K. (2014). Antimicrobial drug resistance in strains of Escherichia coli isolated from food sources. Revista do Instituto de Medicina Tropical de São Paulo, 56, 341–346. doi: 10.1590/S0036-46652014000400012
- Reich, F., Atanassova, V. & Klein, G. (2013). Extended-spectrum β-lactamase- and AmpC-producing enterobacteria in healthy broiler chickens, Germany. Emerging Infectious Diseases, 19, 1253–1259. doi: 10.3201/eid1908.120879
- Schouler, C., Schaeffer, B., Brée, A., Mora, A., Dahbi, G., Biet, F., Oswald, E., Mainil, J., Blanco, J. & Moulin-Schouleur, M. (2012). Diagnostic strategy for identifying avian pathogenic Escherichia coli based on four patterns of virulence genes. Journal of Clinical Microbiology, 50, 1673–1678. doi: 10.1128/JCM.05057-11
- Schwaiger, K., Bauer, J. & Hölzel, C.S. (2013). Selection and persistence of antimicrobial-resistant Escherichia coli including extended-spectrum β-lactamase producers in different poultry flocks on one chicken farm. Microbial Drug Resistance, 19, 498–506. doi: 10.1089/mdr.2012.0257
- Schwaiger, K., Schmied, E.M. & Bauer, J. (2008). Comparative analysis of antibiotic resistance characteristics of Gram-negative bacteria isolated from laying hens and eggs in conventional and organic keeping systems in Bavaria, Germany. Zoonoses and Public Health, 55, 331–341. doi: 10.1111/j.1863-2378.2008.01151.x
- Smet, A., Martel, A., Persoons, D., Dewulf, J., Heyndrickx, M., Catry, B., Herman, L., Haesebrouck, F. & Butaye, P. (2008). Diversity of extended-spectrum beta-lactamases and class C beta-lactamases among cloacal Escherichia coli Isolates in Belgian broiler farms. Antimicrobial Agents and Chemotherapy, 52, 1238–1243. doi: 10.1128/AAC.01285-07
- Vanni, M., Meucci, V., Tognetti, R., Cagnardi, P., Montesissa, C., Piccirillo, A., Rossi, A.M., Di Bello, D. & Intorre, L. (2014). Fluoroquinolone resistance and molecular characterization of gyrA and parC quinolone resistance-determining regions in Escherichia coli isolated from poultry. Poultry Science, 93, 856–863. doi: 10.3382/ps.2013-03627