ABSTRACT
The use of antimicrobials in food animals is the major determinant for the propagation of resistant bacteria in the animal reservoir. However, other factors may also play a part, and in particular vertical spread between the generations has been suggested to be an important transmission pathway. The objective of this paper was to determine the resistance patterns of Escherichia coli isolated from newly-hatched chickens as well as to study the antibiotic pressure effect when amoxicillin was administered during their growing period. With this aim, meconium from 22 one-day-old Ross chickens was analysed. In addition, during their growth period, amoxicillin treatments at days 7, 21 and 35 were carried out. Results showed a high number of E. coli-resistant strains were isolated from the treated one-day-old chickens, and were the highest for β-lactams group, followed by quinolone and tetracyclines. After treatment with amoxicillin, the highest percentage of resistances were detected for this antibiotic compared to the others analysed, with significant differences in resistance percentages between control and treated broilers detected in relation to ampicillin, cephalothin, streptomycin, kanamycin, gentamicin, chloramphenicol and tetracycline. Differences in resistances to ciprofloxacin and nalidixic acid between control and treated animals were not observed and there was lack of resistance for amikacin and ceftriaxone. These results suggest the possibility of vertical transmission of resistant strains to newly-hatched chicks from parent flocks, and seem to indicate that the treatment with amoxicillin increased the resistance of E. coli to other antibiotics.
Introduction
Antimicrobial resistance is of main concern, since antimicrobial-resistant bacteria can pose a risk to human health as a result of potential treatment failure, loss of treatment options and increased likelihood and severity of disease. Treatment failures by multiresistant strains were responsible for half of the approximately 27,000 annual deaths from infections in the European Union (Watson, Citation2008).
The use of antimicrobials to treat and prevent diseases in animals or to promote their growth has been accompanied by the development of antimicrobial resistances. Transmission of resistance from animals to humans can take place through a variety of routes, where the food-borne route probably is the most important. Reduction in the use of antimicrobial agents can have a positive effect on the occurrence of antimicrobial resistance in humans and the use of sub-therapeutic doses in order to increase weight is completely banned in the European Union since January 2006 (Regulation Citation1831/Citation2003/EC).
Resistant strains selected by animal antimicrobial treatments can reach humans via other animals, sewage or other humans, such as farmers or slaughterers (Phillips et al., Citation2003; Miranda et al., Citation2008). Transmission of resistant isolates between animals and the environment necessitates preventive measures. In this sense, addressing zoonotic transmission of pathogens that are resistant to antimicrobials is crucial to stablize effective risk management policies. Amoxicillin is a broad spectrum β-lactam penicillin, introduced in human medicine in the early 1970s and used for the treatment of infections caused by Gram-negative and positive bacteria. In fact, amoxicillin is recommended by the European guidelines as the first-choice antibiotic for treating mild respiratory and other common infections in non-hospitalized patients. For these reasons, when investigating resistance spreading among farm animals, amoxicillin is one of the most interesting antibiotics to study.
Poultry was the most dynamic meat sector during the last decade and the total poultry meat production increased from 69 million tons in 2000 to 94 million tons in 2008, which corresponds to an increase of 35% (FAO, Citation2010). Poultry meat represents 30% of the global meat consumption and chickens, followed by turkeys, are the most common sources of poultry meat (87% and 6.7%, respectively) (FAO, Citation2010). Surveillance of resistance in commensal bacteria is important because they can be reservoirs of resistance determinants and because they are more ubiquitous than pathogens. Exchange of resistance genes occurs between pathogens and nonpathogens, even between Gram-positive and Gram-negative organisms. Of particular interest are enterococci and Escherichia coli, that can play a role in transmission of mobile resistance genes (McEwen & Fedorka-Cray, Citation2002). E. coli isolated from healthy animals, carcasses or meat provides valuable source for investigating the selective pressure exerted by the use of antimicrobials on the intestinal population of bacteria in food-producing animals and to determine the occurrence of resistance to antimicrobials. E. coli is also useful as a representative of Enterobacteriaceae to monitor the emergence and changes in the proportion of bacteria possessing extended spectrum beta-lactamase (EFSA Citation2013).
The objective of this investigation was to determine the antibiotic resistance patterns of E. coli in newly- hatched chickens in order to assess the possibility of vertical transmission from parent flocks, and to study the antibiotic pressure effect that the administration of amoxicillin had in the development of antimicrobial resistance in the commensal gut microbiota.
Materials and methods
Breeding chickens
Twenty-two one-day-old healthy Ross male chickens were obtained from a commercial hatchery. Chicks were labelled and randomly assigned to six groups of three and one with four chicks at “Centro de Investigación Tecnológica Animal” in Segorbe, Castellón (Spain). Transmission of bacteria between groups was avoided by having a solid separation between pens. All the pens had a trough and three water nipple dispensers and the floor was covered with 10 cm of wood shavings. The chickens were fed with the same antimicrobial-free feed. All parts of this study were carried out according to EC council directives (EU Directive Citation2010/63/EU) concerning the laws, regulations and administrative provisions of the member states regarding the protection of animals used for experimental and other scientific purposes (2015/VSC/PEA/00178).
Antibiotic administration
The control group with four chicks was kept untreated. The six remaining groups were treated with amoxicillin (Neudiaval oral powder (50 × 118 g) Laboratory Mevet, Lérida, Spain) according to the manufacturer’s instructions. Chickens were kept in the experimental facilities from day of arrival (day 0) until day of slaughter (day 49) and they were weighed and treated with different doses (D) of amoxicillin: D1, 24 mg amoxicillin/kg body weight; D2, 12 mg amoxicillin/kg and D3, 8 mg amoxicillin/kg. Each dose was administered to two different groups. Dose administration was carried out with an oral syringe. The treatments (T) were administered over 3 days. Treatment one (T1) started on day 7, treatment two (T2) on day 21 and treatment three (T3) on day 35 of the chickens' life. No other antimicrobial treatment was administered during the course of the experiment.
Sample collection
Four sample collections were carried out during the experimental period, immediately before administration of antibiotic doses. The first sampling was on day 0 on meconia, the second and third samplings took place on days 21 and 35. Samples were taken directly from the cloaca using a swab (Deltalab Collection and transport system; Amies swab ps + viscose). The final sampling was on day 49 and samples were taken directly from the caecum after euthanasia with embutramide. In all cases, the samples were refrigerated until they were processed in the laboratory.
E. coli isolation
TBX agar plates (T.B.X. Medium, Oxoid Ltd, Basingstoke, England) were inoculated and incubated at 44°C for 24 h. Two randomly-selected colonies from each plate were transferred to PC agar (Plate Count Agar, Scharlau, Barcelona, Spain) and incubated to 37°C for 24 h. The isolates from the PC agar plates were then confirmed as E. coli with the API-20 E system (Biomérieux, France). Colonies identified as E. coli were selected for the antibiogram test.
Antimicrobial susceptibility
Antimicrobial susceptibility determination of isolated E. coli was completed by a standard disc diffusion assay (Antimicrobial Susceptibility Test Disc, Oxoid Ltd.,) on Mueller-Hinton agar (BBLTM Mueller-Hinton II Agar, BD, Oxoid Ltd). The minimal inhibitory concentration breakpoints and levels of resistance were determined according to the recommendations of the Clinical Laboratory Standards Institute (CLSI, Citation2012). The E. coli strains were tested against 12 antibiotics of veterinary and sanitary significance: gentamycin, 10 μg; amoxicillin/clavulanic acid, 3 μg; ampicillin, 10 μg; amikacin, 30 μg; kanamycin, 30 μg; cloramphenicol, 30 μg; cephalothin, 30 μg; ciprofloxacin, 5 μg; ceftriaxone, 30 μg; tetracycline, 30 μg; nalidixic acid, 30 μg and streptomycin, 10 μg.
Statistical analysis
Statistical analyses of the data were undertaken using Statgraphics Centurion XVI.II (Statpoint Technologies, Inc. Warrenton, VA, USA). The possible relationships between treatments with amoxicillin and the increase in E. coli resistance to different groups of antibiotics were examined with a Multiple Correspondence Analysis (MCA) (Greenacre, Citation2007). In MCA, subjects (rows) and variables (columns) can be depicted simultaneously on a graphical display and a close position between different points indicates a relatively high level of association. On the contrary, when the points are in different parts of the axis, the association is low. Relative proportions were compared using the chi-squared test (χ2) and Fisher’s exact test. Comparisons of means were also performed. A probability value of less than 5% was deemed to be significant.
Results and discussion
Resistances found in day-old chickens
E. coli strains isolated from day-old chicks’ meconium showed resistance in 63.3% (14 out of 22) and, of these, multiresistance, defined as resistance to at least three different antimicrobial classes, was observed in 95% (13 out of 14 meconia). The highest resistance was found against nalidixic acid (80%), ampicillin and amoxicillin/clavulanic acid, both of them in 70% of samples, followed by tetracycline (30%) cephalothin (23.3%) and ciprofloxacin (16.7%). Lower than 10% resistances were found against streptomycin, gentamicin and kanamycin. Finally, none of the E. coli isolates was resistant to amikacin, chloramphenicol and ceftriaxone (). Similar results were found by Martins da Costa et al. (Citation2009) who found no E. coli resistant to chloramphenicol but observed resistant strains from one-day-old chicks against ampicillin, cephalothin, tetracycline, streptomycin, gentamicin and enrofloxacin. As this study was performed with chickens that had not been exposed to antimicrobial agents previously, a vertical transmission of resistant strains from parent flocks (Giovanardi et al., Citation2005) or by contamination in the hatchery environment (Dierikx et al., Citation2013) could be the main causes. Environmental exposure to antibiotics was limited by the design at the experimental farm, in which chickens were maintained in highly hygienic conditions, thus reducing as much as possible the spreading of antibiotic exposure through the environment. Thus, contamination of chickens via vertical transmission seems to be the most probable explanation for the high resistance rates found.
Figure 1. Percentage of E. coli isolates from meconia of day-old chicks with resistance (▪), intermediate resistance (
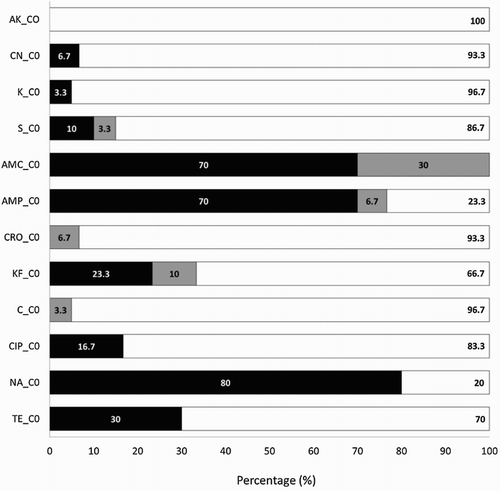
According to Petersen et al. (Citation2006) parents represent an extensive bacterial reservoir from which transmission may occur through contamination of the eggs shell during lay. The study performed by Bortolaia et al. (Citation2010) was also consistent with vertical transmission of ampicillin- and nalidixic acid-resistant E. coli through the broiler production system. These authors concluded that clones among parent and broiler flocks were indistinguishable, which indicated that transmission of ampicillin- and nalidixic acid-resistant E. coli occurred from parents to broilers. Bortolaia et al. (Citation2010) suggested, according to their findings, that resistance to β-lactams and fluoroquinolone in E. coli was due to vertical transmission through parent chickens. In the same framework, Baron et al. (Citation2014) suggested that E. coli resistance may be introduced in the hatchery facilities, either from true vertical transmission when parent poultry stocks are contaminated or from very early contamination in the hatchery itself, or during transport, which is a period when the immature digestive flora is probably very receptive to early colonization, although other possible contamination events occurring thereafter on the production farm cannot be excluded.
Resistance pattern changes during experimental treatments with amoxicillin
During the growth period, three amoxicillin treatments were administered. Results of resistant E. coli isolated strains showed no significant differences between them (P-value 0.1760); however, significant differences were found between isolates from control and treated broilers (P-value 0.0013). Data from the three doses were managed together since differences in the resistances found between the three doses administered were not significant (P-value 0.9025).
shows the MCA carried out to evaluate the global effect of each amoxicillin treatment on the profile of E. coli response (sensitive, intermediate and resistant) to different groups of antibiotics used. Two-dimensional MCA accounts for 23.6% of the inertia (the first dimension accounts for 15.1% and the second 8.5%). Considering the x-axis, resistance and intermediate resistance of E. coli to antibiotics were located on the left; however, antibiotic sensitivity was located on the right. Moreover, considering that the closer the position, the closer is the relationship, results showed that control samples had a higher percentage of E. coli strains sensitive to antibiotics and those obtained from treated chickens had a higher percentage of resistances. In addition, the antibiotics more related with resistance response were ampicillin, amoxicillin, cephalothin, which means that although only one antibiotic was used, it could be influencing other β-lactam antibiotics. To the right side of the figure antimicrobial-sensitive responses to ceftriaxone, kanamicin, gentamicin, ciprofloxacin and chloramphenicol were present illustrating a high antimicrobial sensitivity. Considering the y-axis, fewer differences can be observed. However, the high distance of intermediate response indicates a low percentage of intermediate resistance.
Figure 2. MCA bi-plot. Antibiotics tested: A. AMP = ampicillin; A. C = chloramphenicol; A. CN = gentamicin; A. CRO = ceftriaxone; A. K = kanamycin; A. NA = nalidixic acid; A. SXT = trimethoprim/sulphametoxazol; A. TE = Tetracycline. Before any dose administrations (T. C0), after the three dose administrations (T.T1, T.T2 and T.T3), and the same sampling for the control group (T.C1, T.C2 and T.C3). RV = (resistant values), IV = (intermediate values), SV = (sensitive values).
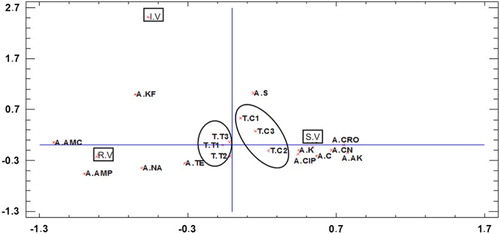
shows the relationship between broiler chickens treatment with amoxicillin and antimicrobial resistance of E. coli to β-lactams (amoxicillin, ampicillin, ceftriaxone and cephalothin); aminoglycosides (amikacin, gentamicin, kanamycin and streptomycin); phenicol (chloramphenicol); quinolones (ciprofloxacin and nalidixic acid) and tetracycline (tetracycline). E. coli strains resistant to amikacin and ceftriaxone were not isolated from either control or treated chickens. Similar results were found by Saenz et al. (Citation2001) and Bywater et al. (Citation2004). Taking into account the critical importance for human medicine of ceftriaxone (WHO, Citation2012; Domenech et al., Citation2015), the obtained results are encouraging.
Figure 3. Percentage of E. coli resistant (▪), intermediate (
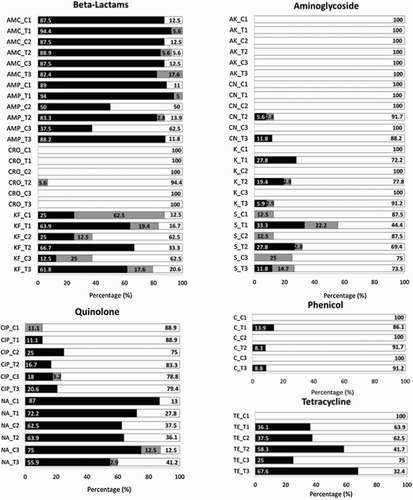
Slight differences in resistances between control and treated flocks were found for ciprofloxacin and nalidixic acid. In addition, antimicrobial resistance in control birds not exposed to antimicrobials exhibited interesting changes over time with ciprofloxacin. In these cases, no resistant strains were present in control 1 (C1), corresponding to day 7 of life, however they appeared in controls 2 and 3. In a previous study, Apajalahti et al. (Citation2004), reported that microbial composition of the chicken gut can change according to the diet and the environment, directly by providing a continuous source of bacteria, or indirectly by influencing bird defence mechanisms. In general, a large numbers of chicks bearing resistant E. coli strains shed huge numbers of resistant organisms, resulting in rapid contamination of the other individuals in the same flock and production barn environment. This high level of contamination is probably difficult to eliminate even with strict disinfection procedures, particularly on farms with outdoor runs (Baron et al., Citation2014).
In this work resistance to nalidixic acid in treated chickens was 64 ± 8.2%. The resistance rates of E. coli reported by EFSA, (Citation2013) vary considerably between countries, from 0.6%, (n = 316) in Finland to 85.1% (n = 101 samples) in Spain. In relation to ciprofloxacin the finding of 16.1 ± 4.8% resistance is higher than those reported in Denmark 9% (n = 134 samples), and lower that of the European mean 53.1% (n = 1703) (EFSA, Citation2013). However, resistance of E. coli to ampicillin, cephalothin, all aminoglycosides studied, chloramphenicol and tetracycline showed high differences between treated and control and only treated birds presented resistance to gentamicin, kanamycin streptomycin and chloramphenicol. Genes encoding for antimicrobial resistance can be horizontally transferred between different bacterial species (Van den Bogaard et al., Citation2001; Binnewies et al., Citation2006). Plasmids carrying genetic determinants which confer resistance to different classes of antibiotics confer a selective advantage for the carrier strains. These plasmids are large, self-conjugative and control their copy number by regulation of the replication rate in the cell (Nordstrom, Citation2005), which could lead to their persistence and spread in the microbial community of the intestine. Miller et al. (Citation2004) showed that exposure to β-lactams induced the dpiBA operon, which inhibits the replication of the bacterial chromosome, inducing the SOS response and induce an enhancement of the genetic variability. These facts could explain the generally higher rates of resistance for the treated group versus the control group.
The higher resistance rates for tetracyclines determined in this work was 67.6%, although the mean value was 37.4%. These values were relatively low compared with a 75% of resistance reported by Sáenz et al. (Citation2001), however, around the European mean value reported by EFSA (Citation2013) 45.2% (n = 2019). Multiresistant strains were more prevalent in each successive trial. This might be explained by the persistence of farm-indigenous E. coli strains that were repeatedly exposed to antimicrobials (Smith et al., Citation2007; Furtula et al., Citation2010).
With regard to public health, amoxicillin, ampicillin and nalidixic acid are on the list of Critically Important Antimicrobials published by the WHO Advisory Group on Integrated Surveillance of Antimicrobial Resistance (WHO, Citation2012), by accomplishing the criterion 1 “the antimicrobial agent is the sole, or one of limited available therapy, to treat serious human disease” and also the criterion 2 “Antimicrobial agent is used to treat diseases caused by either: (1) organisms that may be transmitted to humans from non-human sources or, (2) human diseases causes by organisms that may acquire resistance genes from non-human sources.” Hence, the increase in antimicrobial resistance in general and resistance to these antibiotics in particular may represent a major threat to human health (EU Commission, Citation2010).
To conclude, in this work we have demonstrated the existence of a high percentage of resistant E. coli strains in one-day-old chickens not exposed previously to any antibiotic, which strongly suggests the possibility of vertical transmission from parent flocks. The influence of amoxicillin treatments in increasing resistances to β-lactams, aminoglycosides and cloramphenicol makes it critical to take immediate control measures focusing on reducing both vertical and horizontal transmission in the farm environment.
Acknowledgements
The authors would like to thank the CITA-IVIA for the provision of one-day chickens and their technical support.
References
- Apajalahti, J., Kettunen, A. & Graham, H. (2004). Characteristics of the gastrointestinal microbial communities, with special reference to the chicken. World’s Poultry Science Journal, 60, 223–232. doi: 10.1079/WPS20040017
- Baron, S., Jouy, E., Larvor, E., Eono, F., Bougeard, S. & Kempf, I. (2014). Impact of third-generation-cephalosporin administration in hatcheries on fecal Escherichia coli antimicrobial resistance in broilers and layers. Antimicrobial Agents and Chemotherapy, 58, 5428–5434. doi: 10.1128/AAC.03106-14
- Binnewies, T.T., Motro, Y., Hallin, P.F., Lund, O., Dunn, D., La, T., Hampson, D.J., Bellgard, M., Wassenaar, T.M. & Ussery, D.W. (2006). Ten years of bacterial genome sequencing: comparative-genomics-based discoveries. Functional & Integrative Genomics, 6, 165–185. doi: 10.1007/s10142-006-0027-2
- Bortolaia, V., Bisgaard, M. & Bojesen, A. M. (2010). Distribution and possible transmission of ampicillin- and nalidixic acid-resistant Escherichia coli within the broiler industry. Veterinary Microbiology, 142, 379–386. doi: 10.1016/j.vetmic.2009.10.024
- Bywater, R., Deluyker, H., Deroover, E., Jong, A., Marion, H., McConville, M., Rowan, T., Shryock, T., Shuster, D., Thomas, V., Valle M. & J. Walters. (2004). A European survey of antimicrobial susceptibility among zoonotic and commensal bacteria isolated from food-producing animals. Journal of Antimicrobial Chemotherapy, 54, 744–754. doi: 10.1093/jac/dkh422
- CLSI. (2012). Performance Standards for Antimicrobial Disk Susceptibility Tests; Approved Standards- Eleventh Edition. CLSI document M02-A11. Wayne, PA: Clinical and Laboratory Standards Institute.
- Dierikx, C.M., Van der Goot, J.A., Smith, H.E., Kant, A. & Mevius D.J. (2013). Presence of ESBL/AmpC-producing Escherichia coli in the broiler production pyramid: a descriptive study. PLoS One, 8, e79005. doi: 10.1371/journal.pone.0079005
- Doménech, E., Jimenez-Belenguer, A., Perez, R., Ferrus, M.A. & Escriche, I. (2015). Risk characterization of antimicrobial resistance of Salmonella in meat products. Food Control, 57, 18–23. doi: 10.1016/j.foodcont.2015.04.001
- EFSA. (2013). The European Union summary report on antimicrobial resistance in zoonotic and indicator bacteria from humans, animals and food in 2011. European Food Safety Authority Journal, 11, 3196. Retrieved October, 2015, from http://www.efsa.europa.eu/sites/default/files/scientific_output/files/main_documents/3196.pdf
- EU Commission. (2010). Progress report on the Action plan against the rising threats from antimicrobial resistance Brussels, 11.3.2015 SWD(2015) 59 final. Retrieved September, 2015, from http://ec.europa.eu/health/antimicrobial_resistance/docs/2015_amr_progress_report_en.pdf
- EU Directive 2010/63/EU of the European Parliament and of the Council of 22 September 2010 on the protection of animals used for scientific purposes text with EEA relevance. Retrieved from http://eurlex.europa.eu/legalcontent/ES/TXT/PDF/?uri=CELEX:32010L0063&from=EN.
- FAO. (2010). Agribusiness handbook poultry meat & eggs. Retrieved September, 2015, from http://www.eastagri.org/publications/pub_docs/6_Poultry_web.pdf
- Furtula, V., Farrell, E.G., Diarrassouba, F., Rempel, H., Pritchard, J. & Diarra, M.S. (2010). Veterinary pharmaceuticals and antibiotic resistance of Escherichia coli isolates in poultry litter from commercial farms and controlled feeding trials. Poultry Science, 89, 180–188. doi: 10.3382/ps.2009-00198
- Giovanardi, D., Campagnari, E., Ruffoni, L.S., Pesente, P., Ortali, G. & Furlattini, V. (2005). Avian pathogenic Escherichia coli transmission from broiler breeders to their progeny in an integrated poultry production chain. Avian Pathology, 34, 313–318. doi: 10.1080/03079450500179046
- Greenacre, M. (2007). Correspondence Analysis in Practice 2nd edn. London: Chapman & Hall.
- Martins da Costa, P., Belo, A., Gonçalves, J. & Bernardo, F. (2009). Field trial evaluating changes in prevalence and patterns of antimicrobial resistance among Escherichia coli and Enterococcus spp. isolated from growing broilers medicated with enrofloxacin, apramycin and amoxicillin. Veterinary Microbiology, 139, 284–292. doi: 10.1016/j.vetmic.2009.06.006
- McEwen, S. A. & Fedorka-Cray, P. J. (2002). Antimicrobial use and resistance in animals. Clinical Infectious Diseases, 34, S93–S106. doi: 10.1086/340246
- Miller, C., Thomsen, L. E., Gaggero, C., Mosseri, R., Ingmer, H. & Cohen, S. N. (2004). SOS response induction by β-lactams and bacterial defense against antibiotic lethality. Science, 305, 1629–1631. doi: 10.1126/science.1101630
- Miranda, J. M., Vázquez, B. I., Fente, C. A., Barros-Velázquez, J., Cepeda, A. & Franco, C. M. (2008). Evolution of resistance in poultry intestinal Escherichia coli during three commonly used antimicrobial therapeutic treatments in poultry. Poultry Science, 87, 1643–1648. doi: 10.3382/ps.2007-00485
- Nordstrom, K. (2005). Plasmid R1 – replication and its control. Plasmid, 55, 1–26. doi: 10.1016/j.plasmid.2005.07.002
- Petersen, A., Christensen, J. P., Kuhnert, P., Bisgaard, M. & Olsen, J. E. (2006). Vertical transmission of a fluoroquinolone-resistant Escherichia coli within an integrated broiler operation. Veterinary Microbiology, 116, 120–128. doi: 10.1016/j.vetmic.2006.03.015
- Phillips, I., Casewell, M., Cox, T., De Groot, B., Friis, C., Jones, R., Nightingale, C., Preston, R. & Waddell, J. (2003). Does the use of antibiotics in food animals pose a risk to human health? A critical review of published data. Journal of Antimicrobial Chemotherapy, 53, 28–52. doi: 10.1093/jac/dkg483
- Regulation 1831/2003/EC on additives for use in animal nutrition, replacing Directive 70/524/EEC on additives in feeding-stuffs. Retrieved November, 2015, from http://eur-lex.europa.eu/legal-content/EN/TXT/?uri=CELEX:32003R1831.
- Sáenz, Y., Zarazaga, M., Briñas, L., Lantero, M., Ruiz-Larrea, F. & Torres, C. (2001). Antibiotic resistance in Escherichia coli isolates obtained from animals, foods and humans in Spain. International Journal of Antimicrobial Agents, 18, 353–358. doi: 10.1016/S0924-8579(01)00422-8
- Smith, J. L., Drum, D. J. V., Dai, Y., Kim, J. M., Sanchez, S., Maurer, J. J., Hofacre, C. L. & Lee, M. D. (2007). Impact of antimicrobial usage on antimicrobial resistance in commensal Escherichia coli strains colonizing broiler chickens. Applied and Environmental Microbiology, 73, 1404–1414. doi: 10.1128/AEM.01193-06
- Van den Bogaard, A. E., London, N., Driessen, C. & Stobberingh, E. E. (2001). Antibiotic resistance of faecal Escherichia coli in poultry, poultry farmers and poultry slaughterers. Journal of Antimicrobial Chemotherapy, 47, 763–771. doi: 10.1093/jac/47.6.763
- Watson, R. (2008). Multidrug resistance responsible for half of deaths from healthcare associated infections in Europe. British Medical Journal, 336, 1266–1267. doi: 10.1136/bmj.39601.623808.4E
- WHO. (2012). Critically Important Antimicrobials for Human Medicine 3rd Revision 2011. Geneva, Switzerland: Press, World Health Organization. Retrieved September, 2015, from http://apps.who.int/iris/bitstream/10665/77376/1/9789241504485_eng.pdf