ABSTRACT
Marek’s disease virus (MDV), an alphaherpesvirus, causes Marek’s disease (MD), a lymphoproliferative disease in poultry characterized by T-cell lymphomas, nerve lesions, and mortality. Vaccination is used worldwide to control MD, but increasingly virulent field strains can overcome this protection, driving a need to create new vaccines. Previous studies revealed that insertion of reticuloendotheliosis virus (REV) long terminal repeat (LTR) into a bacterial artificial chromosome (BAC) clone of a very virulent strain of MDV, Md5, rendered the resultant recombinant virus, rMd5 REV-LTR BAC, fully attenuated in maternal antibody positive (Mab+) chickens at passage 40. In the current study, the protective efficacy of rMd5 REV-LTR BAC was evaluated. First, passage 70 was identified as being fully attenuated in maternal antibody negative chickens and chosen as the optimal passage level for use in protective efficacy studies. Second, three protective efficacy trials were conducted comparing the rMd5 REV-LTR p70 BAC to the CVI988/Rispens vaccine. Groups of Mab+ and Mab− 15I5 × 71 chickens were vaccinated in ovo at 18 days of embryonation or intra-abdominally at day of hatch, and challenged at 5 days post-hatch with the vv+MDV strain 686. Vaccination at day of hatch and in ovo with rMd5 REV-LTR p70 BAC protected chickens against MDV-induced bursa and thymic atrophy, but did not provide the same level of protection against MD tumours as that afforded by the commercial vaccine, CVI988/Rispens.
Introduction
Marek’s disease virus (MDV) is classified within the Alphaherpesvirinae subfamily of the Herpesviridae family (Buckmaster et al., Citation1988; van Regenmortel et al., Citation1999; Lee et al., Citation2000), and is further subdivided into three closely related but distinct serotypes, MDV-1, MDV-2, and MDV-3, based upon virulence for chickens, ability to induce T-cell lymphomas, and antigenicity (Payne, Citation1985; Schat, Citation1985). Only viruses within serotype MDV-1 are pathogenic; however, MDV-1 viruses vary considerably in virulence ranging from “virulent” (v), “very virulent” (vv), and “very virulent plus” (vv+) (Witter, Citation1997; Witter et al., Citation2005).
Marek’s disease (MD) is controlled by vaccination of both hens and progeny. Vaccination of hens and transfer of maternal antibody to progeny provide protection against early exposure before vaccine immunity is established following subsequent in ovo vaccination at 18 days of embryonation or vaccination at day of hatch with live vaccine (Kreager, Citation1998). Since the early 1970s, herpesvirus of turkeys (HVT), a naturally occurring non-pathogenic MDV-3 virus isolated from turkeys by Kawamura et al. (Citation1969) and Witter et al. (Citation1970, Citation1972), has been widely used as a vaccine against MDV-1 in the United States; however, excessive mortality or condemnation losses from MD occasionally occurs in vaccinated flocks (Witter, Citation2001). These vaccination failures or breaks have been largely attributed to the emergence of variant MD viruses with increasing virulence (Witter, Citation1997). To protect against these more virulent strains, new vaccine viruses or virus combinations have been introduced into the United States including bivalent vaccines made by combining MDV-2 and MDV-3 strains, most notably HVT and SB-1 (Schat et al., Citation1982; Witter, Citation1982; Calnek et al., Citation1983). In the early 1990s, the CVI988/Rispens strain (Rispens et al., Citation1972a, Citation1972b), an attenuated MDV-1 strain, was recognized as having superior protective properties against challenge with vv pathotype viruses (Witter, Citation1992; Witter et al., Citation1995) and was licensed for use in the United States. Since 1972, CVI988 has been the most widely used vaccine in Europe and other parts of the world. Although a few reports of vaccine failures using CVI988 emerged in the 1990s, CVI988 remains the basis for MD control in Europe and many other parts of the world (Gimeno, Citation2008). Despite the continued success of CVI988, new vaccination strategies are needed since there is always a concern that currently available vaccines will lose efficacy in controlling MDV based upon the continued evolution of MDV towards greater virulence (Witter & Kreager, Citation2004).
Recombination between avian retroviruses and herpesviruses is one strategy currently being used for development of new MD vaccine candidates. Natural recombination is known to occur between avian retroviruses and herpesviruses, and such recombinants have been isolated in commercial chicken and turkey flocks in Israel (Davidson et al., Citation1995; Davidson & Borenshtain, Citation2001; Davidson et al., Citation2002) and in China (Cui et al., Citation2010). Retroviral insertion is not random but is highly specific, with integration occurring at the boundaries between the unique short (US) and the internal repeat short (IRS) flanking the US region of the MDV, the glycoprotein-D-coding region, and the junction of the unique long (UL) and the repeat regions (Jones et al., Citation1993). Retrovirus insertion into MDV may lead to recombinants with altered tissue tropism, spreading pattern, pathogenicity, and reduced vaccination efficacy (Davidson et al., Citation2002; Davidson & Silva, Citation2008).
Previous work has shown that recombination between avian retroviruses and herpesviruses, either natural or by molecular manipulation, may lead to novel MD vaccine candidates. Co-cultivation of REV with virulent MDV (strain JM/102W) resulted in the insertion and duplication of the REV LTR into the MDV genome. The resultant virus, RM-1 (Isfort et al., Citation1992; Jones et al., Citation1993; Kost et al., Citation1993; Jones et al., Citation1996), was non-oncogenic and highly protective against challenge with vv+MDV; it however caused bursa and thymus atrophy and thus was unsuitable for commercial use (Witter et al., Citation1997). Lupiani et al., (Citation2013) inserted the REV LTR into the CVI988 cosmid genome and the resultant virus, denoted as CVRM, exhibited enhanced growth in cell culture, and superior protection against challenge with vv+MDV.
Since insertion of the REV LTR into JM102/W resulted in phenotypic changes in the resultant virus, RM1, and was highly protective against vv+MDV challenge, we hypothesized that insertion of REV LTR into an analogous site in a different MDV may result in a similar phenotypic change, leading to a protective vaccine. The REV LTR was previously cloned into the rMd5 B40 BAC clone (Kim et al., Citation2011) and the resultant recombinant virus, rMd5 REV-LTR BAC, was fully attenuated at passage 40 in Mab+ chickens (Mays et al., Citation2012). In this current study, the protective efficacy of the rMd5 REV-LTR BAC against challenge with the vv+MDV strain, 686 (Witter et al., Citation2005), was evaluated after administration at hatch or in ovo.
Materials and methods
Viruses, cells, and chickens
Viruses were propagated on primary duck embryo fibroblasts (DEF) maintained in Leibovitz L-15 medium plus McCoy 5A medium (1:1), supplemented with 2.5% bovine serum and antibiotics (Witter et al., Citation1980). All virus stocks were titrated on DEF cells by serial dilution and enumeration of plaques. Chickens were the progeny of a cross between 15I5 males and line 71 females (Bacon et al., Citation2000) with or without maternal antibody. The pathotype of the MDV challenge strain, 686, has been classified as a very virulent plus (vv+MDV) (Witter et al., Citation2005). CVI988/Rispens was purchased from Intervet USA, Inc. (Millsboro, DE) and prepared as recommended by the manufacturer.
The rMd5 REV-LTR BAC is an infectious Md5 BAC clone in which the REV LTR was inserted into the IRS region (Kim et al., Citation2011). During blind passage in DEF cultures, the initial LTR in the rMd5 REV-LTR B40 BAC was duplicated, with LTRs appearing in both the IRS and TRS regions of the MDV genome. This rMd5 REV-LTR virus was fully attenuated in Mab+ 15I5 × 71 chickens at passage 40 (Mays et al., Citation2012).
Viral DNA purification and sequence analysis
The rMd5 REV-LTR B40 BAC virus at passage 10 and passage 70 were propagated on confluent DEFs using a multiplicity of infection of 0.1. After 5 days when the cytopathic effect was approximately 80%, the cells were trypsinized and processed according to the micrococcal nuclease procedure (Volkening & Spatz, Citation2009) in order to isolate nucleocapsid DNA. The quality of the DNA was assessed by BamHI restriction digestion followed by 0.6% agarose gel electrophoresis.
High-throughput DNA sequencing
Sequencing of the rMd5 REV-LTR genome (p10 and p70) was accomplished using Illumina sequence-by-synthesis (MiSeq) on the Illumina MiSeq 2000 sequencing platform running HCS v1.4.8 (Illumina, Inc., San Diego, CA, USA) at the University of Georgia genomics centre (Athens, GA, USA).
For Illumina sequencing, 50 ng of viral DNA was simultaneously fractionated and tagged using an Illumina Nextera sample preparation kit (Illumina, Inc.). The quality of the libraries and the average fractionated size were determined using an Agilent 2100 Bioanalyzer (Agilent Technologies, Inc., Santa Clara, CA, USA). The samples were processed and indexed and 8 pM of the pool was run on the Illumina MiSeq sequencer using paired-end 150-bp sequencing. The first run sequencing used the Illumina Miseq reagent kit (300 cycles for the 2 × 150 base pair sequencing). The second run-off sequencing used Illumina MiSeq reagent kit V2 (500 cycles for the 2 × 250 base pair-end sequencing). The first read was 100 cycles in length, followed by a 6-cycle index read. Image analysis and base calling were performed using the standard Illumina Pipeline consisting of Real Time Analysis version v1.12.4.2 and Casava v1.8 with default settings.
Approximately 1 million and 2 million 250-bp paired-end MiSeq reads were generated for the early and late passage samples with the nominal insert size of 500 BP. Removal of low-quality reads and mapping with Bowtie2 version 2.2.1 (Langmead & Salzberg, Citation2012) to the reference Md5B40BAC genome (Niikura et al., Citation2011) with default paired-end parameters resulted in approximately 421,000 and 106,000 total reads, resulting in approximately a 285- and 83-fold coverage for passages 10 and 70, respectively. The LTR insertion with flanking MDV regions was assembled using Velvet version 1.2.10 (Zerbino & Birney, Citation2008) with a Columbus extension with reads blasting (Altschul et al., Citation1997) against the reference sequences, Gallid herpesvirus 2 (|NC_002229.3; Tulman et al., Citation2000) or REV (|NC_006934.1; Wang & Cui, Citation2006), with approximately 440,000 and 75,000 reads, respectively. The assemblies for both early and late passages at k values ranging from 21 to 71 step size 10 matched the LTR reference (Jones et al., Citation1996) exactly, with flanking sequence matching insertion sites at 152,216 and 171,754 within both early and late passages. A strain-specific genome was generated by inserting the LTR sequence into both regions of the parent reference and validated by mapping. Both early and late passages showed a read depth of at least 70 and 45 across the insertion sites. Consensus sequences were calculated from these alignments using SAMTools (Li et al., Citation2009). All calculations were performed at Michigan State University’s High Performance Computing Facility (East Lansing, MI, USA). The exact locations of the LTRs were confirmed using anchor PCR and Sanger sequencing.
Pathogenicity study
Groups of 27, day-old MDV Mab− 15I5 × 71 chickens were inoculated at hatch with 2000 plaque-forming units (PFU) rMd5 REV-LTR virus from passage levels 10, 40, 60, 70, and 80, along with the parent rMd5 BAC virus from passage level 10. Five chickens per group were bled at 1 and 3 weeks of age and evaluated for viremia. All surviving chickens were terminated at 8 weeks of age. Ratios of bursa to body weight and thymus to body weight were determined for five chickens per group at 1 and 3 weeks, and all surviving chickens at 8 weeks of age, and compared to age-matched non-infected chickens. All bird experiments were conducted under the guidance and approval of the Avian Disease and Oncology Laboratory Institutional Animal Care and Use Committee.
Protection studies
Protection efficacy studies were conducted to determine whether the rMd5 REV-LTR virus at passage 70 was protective against challenge with the vv+MDV strain, 686, at passage 10.
Vaccination at day of hatch
The protection study involved vaccination of groups of 20 chicks at day of hatch with 2000 PFU of vaccine and challenge with 500 PFU of the vv+MDV strain 686 (p10) at day 5 post-vaccination (Witter et al., Citation2005). The protective efficacy of the rMd5 REV-LTR p70 virus was analysed against the protective efficacy of the CVI988/Rispens commercial vaccine in both Mab− and Mab+ chickens. At 3 weeks post-vaccination, three chickens per group were bled and euthanized and analysed for virus isolation, bursa/body weight ratio, and thymus/body weight ratio. All remaining chickens were terminated at 8 weeks post-vaccination, bled, and analysed for virus isolation, bursa/body weight ratio, and thymus/body weight ratio. All chickens that died or were euthanized were necropsied and evaluated for pathological lesions. Data were collected from a total of four replicates.
In ovo vaccination
Additionally, three independent trials were conducted to evaluate the protective efficacy of in ovo vaccination with rMd5 REV-LTR virus p70. Subsets of 15I5 × 71 Mab− and Mab+ embryos were vaccinated at 18 days of embryonation with 0.1 ml sterile phosphate-buffered saline or 2000 PFU/0.1 ml vaccine using a 22 gauge, 1½ inch needle inserted directly into the middle of the air sac. Care was taken as to not to inject the embryo. The pinpoint hole was sealed with either Duco® Cement or Elmer’s® Glue All® Multi-Purpose Glue and the inoculated embryos were transferred to hatching trays and placed into an incubator until hatch. In ovo vaccinated chicks were challenged with 500 PFU vv+MDV strain, 686, at 5 days of age. The study parameters were the same as the protection studies.
Statistical analysis
The significance of differences in percentages was determined by Chi-square analysis and t-test analysis. Significance was assumed at the 0.05 level of probability.
Results
Sequencing
The genome of the rMd5 REV-LTR BAC was sequenced at passage 10 and at passage 70. Sequence analysis confirmed that the REV-LTR sequence was inserted within the IRS and TRS region at nucleotide position 152,216 and nucleotide position 171,754 within the rMd5 REV LTR B40 BAC sequence for both the early and late passages (). The overall sequence homology between the two passage levels was over 99%. Only four single nucleotide polymorphisms were identified between the genomes of the p10 and p70 rMd5 REV-LTR BAC viruses (see supplementary table). The complete sequences have been submitted to GenBank with accession numbers KT833851 and KT833852 for p10 and p70 sequences, respectively.
Pathogenicity study
The rMd5 REV-LTR p70 was determined as the optimal passage level for conducting further protective efficacy studies based upon uniform lack of bursa and thymic atropy at 1 week, 3 weeks, and at 8 weeks of age (), and a lack of MDV-induced lesions in Mab− 15I5 × 71 chickens ().
Figure 2. Box-plot of organ weight/body weight ratios of vaccinated Mab− chickens at one week, three weeks, and eight weeks post-vaccination. Chickens were vaccinated at day of hatch with phosphate-buffered saline (Non), rMd5 REV-LTR BAC at different passage levels or rMd5 B40 BAC at passage level 10. Five birds were analysed per group at one week and three weeks. All surviving birds were analysed at eight weeks. The error bars represent the range of values from the largest to the smallest. (a) Relative bursa/body weight and thymus/body weight ratios at one week post-vaccination. (b) Relative bursa/body weight and thymus/body weight ratios at three weeks post-vaccination. (c) Relative bursa/body weight and thymus/body weight ratios at eight weeks post-vaccination.
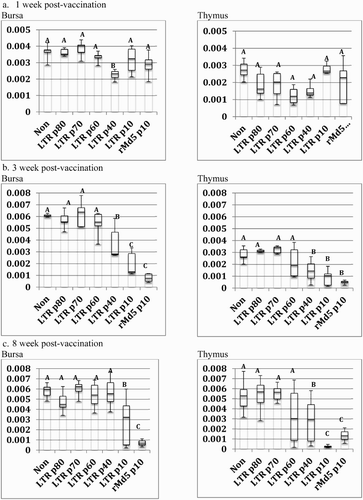
Table 1. MD lesions in maternal antibody-negative 15I5 × 71 chickens inoculated at hatch with recombinant MDV-BAC clone containing REV-LTR at various passage levels and challenged with a vv+MDV, strain 686 at 5 days of age.
Protection studies
After an initial protection study demonstrated that the rMd5 REV-LTR p70 was protective against challenge with a vv+MDV strain, 686, in Mab+ chickens during a pilot study (data not shown), a more extensive protection efficacy study was conducted in both Mab− and Mab+ chickens to compare the protection elicited by rMd5 REV-LTR p70 relative to the “gold-standard” vaccine CVI988/Rispens.
Vaccination at day of hatch
The rMd5 REV-LTR BAC p70 virus protected against early death in both Mab− and Mab+ chickens after challenge with vv+MDV (). Only 19% of Mab− chickens vaccinated at hatch with LTR p70 virus demonstrated signs of MDV-induced lesions including bursal and thymic atrophy (BTA) as a result of MDV pathology, as compared to 18% of Mab− chickens vaccinated with CVI988/Rispens, (). Vaccination with rMd5 REV-LTR p70 provided protection against BTA as compared to non-vaccinated challenged chickens (). Overall the bursa/body weight and thymic/body weight ratios for Mab− chickens receiving the rMd5 REV-LTR p70 were not significantly different than ratios from birds vaccinated with CVI988 and challenged with 686 MDV. All but one Mab− non-vaccinated chicken challenged with MDV strain 686 died from MDV-induced lesions and pathology before the termination of the experiment at 56 days post-inoculation ().
Figure 3. Survival curves of chickens vaccinated at hatch and challenged at 5 days of age. (a) Survival curve for Mab− chickens. (b) Survival curve for Mab+ chickens.
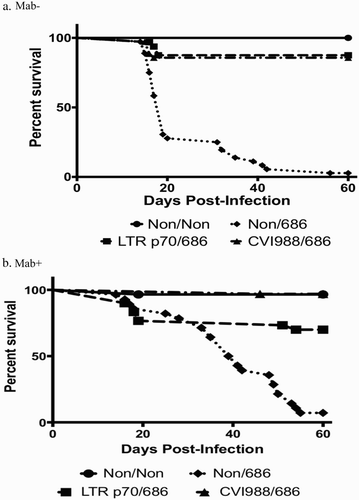
Figure 4. Box-plot of organ weight/body weight ratios of chickens vaccinated at hatch and challenged at 5 days of age. Chickens were vaccinated at day of hatch with phosphate-buffered saline (Non), rMd5 REV-LTR BAC p70, or CVI988/Rispens. The chickens were challenged with vv+MDV strain, 686, at 5 days of age. The error bars represent the range of values from the largest to the smallest. (a). Relative bursa/body weight and thymus/body weight ratios at three weeks post-challenge. (b) Relative bursa/body weight and thymus/body weight ratios at eight weeks post-challenge.
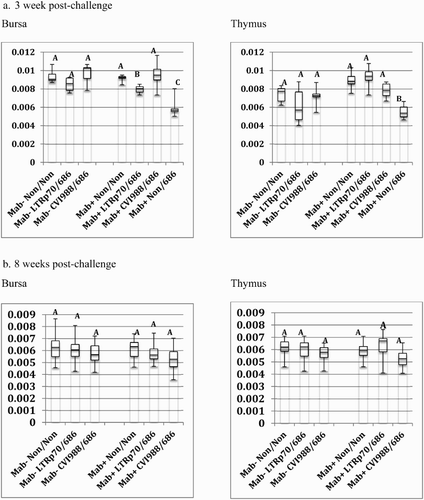
Table 2. Protective efficacy of the rMDV REV-LTR BAC virus at passage 70 in 15I5 × 71 chickens with or without maternal antibodies vaccinated in ovo or hatch and challenged with vv+MDV strain 686 at 5 days of age.
The rMd5 REV-LTR p70 BAC virus was not as protective as CVI988/Rispens in Mab+ chickens when vaccinated at hatch and challenged with vv+MDV. Only 57% of the Mab+ chickens vaccinated with LTR p70 virus were protected as compared to 95% protection with the CVI988/Rispens vaccine (). Additionally, the bursa/body weight ratio of the Mab+ chickens vaccinated with rMd5 REV-LTR p70 and challenged with 686 MDV was significantly lower than the non-vaccinated non-challenged chickens and CVI988/Rispens-challenged chickens at 3 weeks post-challenge; however, the bursa/body weight ratio was not significantly different at 8 weeks post-challenge ().
In ovo vaccination
Vaccination with rMd5 REV-LTR p70 virus at 18 days of embryonation did not produce BTA (). Additionally, in ovo vaccination with rMd5 REV-LTR p70 afforded protection against bursal and thymic atrophy in Mab-vaccinated and challenged chickens. Vaccinated Mab+ chickens experienced bursal atrophy at three weeks post-challenge; however, there was no bursal or thymic atrophy in rMd5 REV-LTR p70 in ovo vaccinated chickens at 8 weeks post-challenge. The overall bursa/body weight and thymus/body weight ratios of the in ovo vaccinated chickens were less than chickens vaccinated at hatch, including chickens sham vaccinated at 18 days of embryonation with sterile phosphate buffered saline (PBS), indicating that the process of in ovo vaccination itself may lead to reduction in immune organ size but not significantly. Although the LTR p70 virus protected against BTA following challenge with vv+MDV, the LTR p70 virus was not as protective as the CVI988/Rispens vaccine in prevention of MDV-induced early mortality () and pathology when administered in ovo at 18 days of embryonation in both Mab− and Mab+ susceptible chickens ().
Figure 5. Box-Plot of organ weight/body weight ratios of in ovo vaccinated chickens. Chickens were vaccinated at 18 days of embryonation and challenged at 5 days of age with vv+MDV strain, 686. The error bars represent the range of values from the largest to the smallest. (a) Bursa/body weight and thymus/body weight ratios at 3 weeks post-challenge. (b) Bursa/body weight and thymus/body weight ratios at 8 weeks post-challenge.
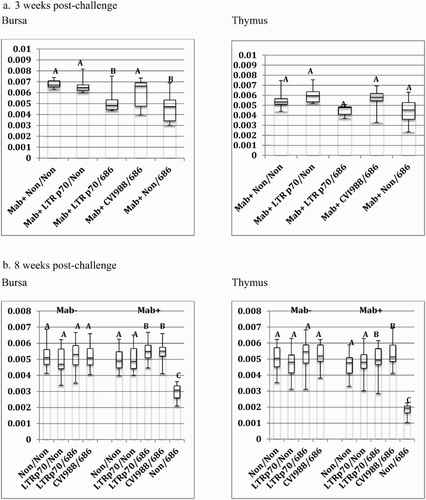
Discussion
The rMd5 REV-LTR p70 BAC virus was fully attenuated and did not induce bursal or thymic atrophy in specific pathogen free (SPF) Mab− chickens when administered both in ovo at 18 days of embryonation and at hatch. This is important since current USDA licensing regulations require vaccine safety tests conducted in SPF chickens lacking maternal antibody against MDV. Retroviral LTR insertion into MDV does not necessarily lead to attenuation although it may alter the phenotype upon passage of the resultant recombinant virus. For example, early passages of the rMd5 REV-LTR BAC (passage 10) were as pathogenic as the very virulent Md5 B40 BAC parental virus. Serial passage in DEF cells led to attenuation of the rMd5 REV-LTR BAC virus at passage 70, but over-attenuation was noted for higher passages (passage 90) (data not shown). In contrast, the RM1 virus was highly attenuated at passage 40, but induced severe bursa/thymus atrophy and immunosuppression similar to that seen with very virulent pathotypes of MDVs (Witter et al., Citation1997). Interestingly, two natural recombinants, GX0101 and GD0202 (Zhang & Cui, Citation2005), containing a single 539 bp REV LTR insert located 267 bp upstream of the sorf2 gene (Su et al., Citation2013) of an MDV virus, were more pathogenic than the virulent MDV GA strain, but less pathogenic than the very virulent MDV Md5 strain (Sun et al., Citation2010). In fact, these LTR-containing MDV strains were less pathogenic than a recombinant (GX0101 Δ LTR BAC) in which the LTR was deleted, further demonstrating that insertion of an REV LTR into the genome of MDV can not only alter the pathogenicity (e.g. immunosuppression, mortality, and tumourigenicity) of the resultant viruses, but also modify the growth (Sun et al., Citation2009, Citation2010). The viraemia levels in the GX0101 Δ LTR-infected group were significantly lower than that of the GX010 group that contained the REV LTR (Sun et al., Citation2010). Only after deletion of the meq gene was the natural MDV REV LTR recombinant, GX0101, fully attenuated (Su et al., Citation2015). Insertion of an REV LTR into the genome of a vaccine strain CVI988 BAC virus, which is fully attenuated, did not alter the pathogenicity; however growth of the recombinant was altered. Lupiani et al. (Citation2013) reported that the recombinant virus, CVRM, replicated to higher titres in cell culture than the parental virus CVI988 BAC.
The rMd5 REV-LTR p70 BAC was less protective against vv+MDV challenge than CVI988. Highly protective MD vaccines are capable of replicating better in the lymphoid organs than less protective MD vaccines (Gimeno et al., Citation2004; Gimeno et al., Citation2011). However, the most protective vaccine candidates sometimes induce severe lymphoid organ atrophy in chickens lacking maternal antibodies against MDV (Witter & Kreager, Citation2004; Lee et al., Citation2008; Lee et al., Citation2010). Viremia data indicated that the LTR p70 virus replicated as well as CVI988 in vaccinated chickens when administered at hatch (data not shown).
Additionally, the LTR p70 virus did not induce bursal or thymic atrophy when administered to both Mab− and Mab+ at hatch or at 18 days of embryonation. These data are especially important for the commercial broiler industry where the majority of MD vaccines are administered in ovo. In this current study, the CVI988 vaccine was equally effective in both Mab− and Mab+ chickens with in ovo vaccination, whereas the LTR p70 BAC vaccine was less effective in Mab− chickens. It is known that the presence of Mab provides early protection against MDV infection (Sharma & Graham, Citation1982).
In conclusion, although vaccination at day of hatch and in ovo vaccination with rMd5 REV LTR p70 BAC protected chickens against MDV-induced BTA, the level of protection conferred by this vaccine candidate against MD tumours was lower than that conferred by the commercial vaccine, CVI988/Rispens, following challenge with a highly virulent vv+MDV strain.
Supplementary_Table.docx
Download MS Word (12.6 KB)Acknowlegements
The authors thank Dr Hans Cheng for his advice and expertise on compiling the rMd5 REV-LTR BAC genome sequences. The authors thank Dr Masahiro Niikura for the generous gift of the rMd5 B40 MDV-BAC clone. In addition, the authors thank Mr Lonnie Milam and Ms Melanie Flesberg for their excellent technical assistance. Mention of trade names or commercial products in this publication is solely for the purpose of providing specific information and does not imply recommendation or endorsement by the Department of Agriculture.
Disclosure statement
No potential conflict of interest was reported by the authors.
References
- Altschul, S.F., Madden, T.L., Scaffer, A.A., Zhang, J., Zhang, Z., Miller, W. & Lipman, D.J. (1997). Gapped BLAST and PSI-BLAST: a new generation of protein database search programs. Nucleic Acids Research, 25, 3389–3402. doi: 10.1093/nar/25.17.3389
- Bacon, L.D., Hunt, H.D. & Cheng, H.H. (2000). A review of the development of chicken lines to resolve genes determining resistance to diseases. Poultry Science, 79, 1082–1093. doi: 10.1093/ps/79.8.1082
- Buckmaster, A.E., Scott, S.D., Sanderson, M.J., Boursnell, M.E.G., Ross, L.J.N. & Binns, M.M. (1988). Gene sequence and mapping data from Marek’s disease virus and herpesvirus of turkeys: implications for herpesvirus classification. Journal of General Virology, 69, 2033–2042. doi: 10.1099/0022-1317-69-8-2033
- Calnek, B.W., Schat, K.A., Peckham, M.C. & Fabricant, J. (1983). Field trials with a bivalent vaccine (HVT and SB-1) against Marek’s disease. Avian Diseases, 27, 844–849. doi: 10.2307/1590330
- Cui, Z., Zhuang, G., Xu, X., Sun, A. & Su, S. (2010). Molecular and biological characterization of a Marek’s disease virus field strain with reticuloendotheliosis virus LTR insert. Virus Genes, 40, 236–243. doi: 10.1007/s11262-009-0437-z
- Davidson, I. & Borenshtain, R. (2001). In vivo events of retroviral long terminal repeat Integration into Marek’s disease virus in commercial poultry: detection of chimeric molecules as a marker. Avian Diseases, 45, 102–121. doi: 10.2307/1593017
- Davidson, I., Borenshtain, R., Kung, H.-J. & Witter, R.L. (2002). Molecular indications for in vivo integration of the avian leukosis virus, subgroup J-long terminal repeat into the Marek’s disease virus in experimentally dually-infected chickens. Virus Genes, 24, 173–180. doi: 10.1023/A:1014528818179
- Davidson, I., Borovskaya, A., Perl, S. & Malkinson, M. (1995). User of the polymerase chain reaction for the diagnosis of natural infection of chickens and turkeys with Marek’s disease virus and reticuloendotheliosis virus. Avian Pathology, 24, 69–94. doi: 10.1080/03079459508419050
- Davidson, I. & Silva, R.L. (2008). Creation of diversity in the animal virus world by inter-species and intra-species recombinations: lessons learned from poultry viruses. Virus Genes, 36, 1–9. doi: 10.1007/s11262-007-0165-1
- Gimeno, I.M. (2008). Marek’s disease vaccines: a solution for today but a worry for tomorrow? Vaccine, 26 Suppl. 3, C31–41. doi: 10.1016/j.vaccine.2008.04.009
- Gimeno, I.M., Witter, R.L., Cortes, A.L. & Reed, W.M. (2011). Replication ability of three highly protective Marek’s disease vaccines: implications in lymphoid organ atrophy and protection. Avian Pathology, 40, 573–579. doi: 10.1080/03079457.2011.617725
- Gimeno, I.M., Witter, R.L., Hunt, H.D., Reddy, S.M. & Reed, W.M. (2004). Biocharacteristics shared by highly protective vaccines against Marek’s disease. Avian Pathology, 33, 57–66. doi: 10.1080/0307945031000163264
- Isfort, R., Jones, D., Kost, R., Witter, R. & Kung, H.-J. (1992). Retrovirus insertion into herpesvirus in vitro and in vivo. Proceedings of the National Academy of Sciences, 89, 991–995. doi: 10.1073/pnas.89.3.991
- Jones, D., Brunovskis, P., Witter, R. & Kung, H-J. (1996). Retroviral insertional activation in a herpesvirus: transcriptional activation of Us genes by an integrated long terminal repeat in a Marek’s disease virus clone. Journal of Virology, 70, 2460–2467.
- Jones, D., Isfort, R., Witter, R., Kost, R. & Kung, H.-J. (1993). Retroviral insertions into a herpesvirus are clustered at the junctions of the short repeat and short unique sequences. Proceedings of the National Academy of Sciences of the United States of America, 90, 3855–3859. doi: 10.1073/pnas.90.9.3855
- Kawamura, H., King, Jr., D.J. & Anderson, D.P. (1969). A herpesvirus isolated from kidney cell culture of normal turkeys. Avian Diseases, 13, 853–863. doi: 10.2307/1588592
- Kim, T., Mays, J., Fadly, A. & Silva, R.F. (2011). Artificially inserting a reticuloendotheliosis virus long terminal repeat into a bacterial artificial chromosome clone of a Marek’s disease virus (MDV) alters expression of nearby MDV genes. Virus Genes, 42, 369–376. doi: 10.1007/s11262-011-0585-9
- Kost, R., Jones, D., Isfort, R., Witter, R. & Kung, H.-J. (1993). Retrovirus insertion into herpesvirus: characterization of a Marek’s disease virus harboring a solo LTR. Virology, 192, 161–169. doi: 10.1006/viro.1993.1018
- Kreager, K.S. (1998). Chicken industry strategies for control of tumor virus infections. Poultry Science, 77, 1213–1216. doi: 10.1093/ps/77.8.1213
- Langmead, B. & Salzberg, S. (2012). Fast gapped-read alignment with Bowtie2. Nature Methods, 9, 357–359. doi: 10.1038/nmeth.1923
- Lee, L., Lupiani, B., Silva, R.F., Kung, H.-J. & Reddy, S.M. (2008). Recombinant Marek’s disease virus (MDV) lacking the Meq oncogene confers protection against challenge with a very virulent plus strain of MDV. Vaccine, 26, 1887–1892. doi: 10.1016/j.vaccine.2008.01.046
- Lee, L.F., Kreager, K.S., Arango, J., Paraguassu, A., Beckman, B., Zhang, H., Fadly, A., Lupiani, B. & Reddy, S.M. (2010). Comparative evaluation of vaccine efficacy of recombinant Marek’s disease virus vaccine lacking Meq oncogene in commercial chickens. Vaccine, 28, 1294–1299. doi: 10.1016/j.vaccine.2009.11.022
- Lee, L.F., Wu, P., Sui, D., Ren, D., Kamil, J., Kung, H.J. & Witter, R.L. (2000). The complete unique long sequence and the overall genomi organization of the GA strain of Marek’s disease virus. Avian Diseases, 47, 549–558. doi: 10.1637/6073
- Li, H., Handsaker, B., Wysoker, A., Fennell, T., Ruan, J., Homer, N., Marth, G., Abecasis, G. Durbin, R. & 1000 Genome Project Data Processing Subgroup. (2009). The sequence alignment/map (SAM) format and SAMtools. Bioinformatics, 25, 2078–2079. doi: 10.1093/bioinformatics/btp352
- Lupiani, B., Lee, L.F., Kreager, K.S., Witter, R.L. & Reddy, S.M. (2013). Insertion of reticuloendotheliosis virus long terminal repeat into the genome of CVI988 strain of Marek’s disease virus results in enhanced growth and protection. Avian Diseases, 57, 427–431. doi: 10.1637/10445-110412-ResNote.1
- Mays, J.K., Silva, R.F., Kim, T. & Fadly, A. (2012). Insertion of reticuloendotheliosis virus long terminal repeat into a bacterial artificial chromosome clone of a very virulent Marek’s disease virus alters its pathogenicity. Avian Pathology, 41, 259–265. doi: 10.1080/03079457.2012.675428
- Niikura, M., Kim, T., Silva, R.F., Dodgson, J. & Cheng, H.H. (2011). Virulent Marek’s disease virus generated from infectious bacterial artificial chromosome clones with complete DNA sequence and the implication of viral genetic homogeneity in pathogenesis. Journal of General Virology, 92, 598–607. doi: 10.1099/vir.0.026864-0
- Payne, L.N. (1985). Pathology. In L.N. Payne (Ed.), Marek’s disease (pp. 43–76). Hingham, MA: Kluwer Academic Publishers.
- Rispens, B.H., Van Vloten, H., Mastenbroek, N., Maas, H.J.L. & Schat, K.A. (1972a). Control of Marek’s disease in the Netherlands. I. Isolation of an avirulent Marek’s disease virus strain (CVI 988) of Marek’s disease virus. Avian Diseases, 16, 108–125. doi: 10.2307/1588905
- Rispens, B.H., Van Vloten, H., Mastenbroek, N., Maas, H.J.L. & Schat, K.A. (1972b). Control of Marek’s disease in the Netherlands. II. Field trials on vaccination with an avirulent strain (CVI 988) of Marek’s disease virus. Avian Diseases, 16, 126–138. doi: 10.2307/1588906
- Schat, K.A. (1985). Characteristics of the virus. In L.N. Payne (Ed.), Marek’s disease (pp. 77–112). Hingham, MA: Kluwer Academic Publishers.
- Schat, K.A., Calnek, B.W. & Fabricant, J. (1982). Characterization of two highly oncogenic strains of Marek’s disease virus. Avian Pathology, 11, 593–605. doi: 10.1080/03079458208436134
- Sharma, J.M. & Graham, C.K. (1982). Influence of maternal antibody on efficacy of embryo vaccination with cell-associated and cell-free Marek’s Disease vaccine. Avian Diseases, 26, 860–870. doi: 10.2307/1589874
- Su, S., Cui, N., Sun, A., Li, Y., Ding, J., Chen, Z., Zhao, P. & Cui, Z. (2013). Sequence analysis of the whole genome of a recombinant Marek’s disease virus strain, GX0101, with a reticuloendotheliosis virus LTR insert. Archives of Virology, 158, 2007–2014. doi: 10.1007/s00705-013-1671-1
- Su, S. Cui, N., Zhou, Y., Chen, Z., Li, Y., Ding, J., Wang, Y., Duan, L., and Cui, Z. (2015). A recombinant field strain of Marek’s disease (MD) virus with reticuloendotheliosis virus long terminal repeat insert lacking the meq gene as a vaccine against MD. Vaccine, 33, 596–603. doi: 10.1016/j.vaccine.2014.12.057
- Sun, A.-J., Petherbridge, L., Zhao, Y.-G., Li, Y.-P., Nair, V.K. & Cui, Z.-Z. (2009). A BAC clone of MDV strain GX0101 with REV-LTR integration retained its pathogenicity. Chinese Science Bulletin, 54, 2641–2647.
- Sun, A.-J., Xu, X.-Y., Petherbridge, L., Zhao, Y.-G., Nair, V. & Cui, Z.-Z. (2010). Functional evaluation of the role of reticuloendotheliosis virus long terminal repeat (LTR) integrated into the genome of a field strain of Marek’s disease virus. Virology, 397, 270–276. doi: 10.1016/j.virol.2009.11.017
- Tulman, E.R., Afonso, C.L., Lu, Z., Zsak, L., Rock, D.L. & Kutish, G.F. (2000). The genome of a very virulent Marek’s disease virus. Journal of Virology, 74, 7980–7988. doi: 10.1128/JVI.74.17.7980-7988.2000
- van Regenmortel, M.H.V., Fauquet, C.M., Bishop, D.H.L., Carstens, E., Estes, M.K., Lemon, S., Maniloff, J., Mayo, M.A., McGeoch, D., Pringle, C.R. & Wickner, R.B. (Ed.). (1999). Virus taxonomy. Seventh Report of the International Committee on Taxonomy of Viruses. New York, NY: Academic Press.
- Volkening, J.D. & Spatz, S.J. (2009). Purification of DNA from the cell-associated herpesvirus Marek’s disease virus for 454 pyrosequencing using micrococcal nuclease digestion and polyethylene glycol precipitation. Journal of Virological Methods, 157, 55–61. doi: 10.1016/j.jviromet.2008.11.017
- Wang, Y. & Cui, Z. (2006). Sequence analysis for the complete proviral genome of reticuloendotheliosis virus Chinese strain HA9901. Science in China Series C Life Sciences, 49, 149–157.
- Witter, R.L. (1972). Turkey herpesvirus: lack of oncogenicity for turkeys. Avian Diseases, 16, 666–670. doi: 10.2307/1588684
- Witter, R.L. (1982). Protection by attenuated and polyvalent vaccines against highly virulent strains of Marek’s disease virus. Avian Pathology, 11, 49–62. doi: 10.1080/03079458208436081
- Witter, R.L. (1992). Safety and comparative efficacy of the CVI988/Rispens vaccine strain. In G. deBoer & S.H.M. Jeurissen (Eds.), Proceedings of the 4th International Symposium on Marek’s Disease (pp. 315–319). Wageningen: Ponsen & Looijen.
- Witter, R.L. (1997). Increased virulence of Marek’s disease virus field isolates. Avian Diseases, 41, 149–163. doi: 10.2307/1592455
- Witter, R.L. (2001). Protective efficacy of Marek’s disease vaccines. Current Topics of Microbiology and Immunology, 255, 57–90.
- Witter, R.L., Calnek, B.W., Buscaglia, C., Gimeno, I.M. & Schat, K.A. (2005). Classification of Marek’s disease viruses according to pathotype: philosophy and methodology. Avian Pathology, 34, 75–90. doi: 10.1080/03079450500059255
- Witter, R.L. & Kreager, K.S. (2004). Serotype 1 viruses modified by backpassage or insertional mutagenesis: approaching the threshold of vaccine efficacy in Marek’s disease. Avian Diseases, 48, 768–782. doi: 10.1637/7203-050304R
- Witter, R.L., Lee, L.F. & Fadly, A.M. (1995). Characteristics of CVI988/Rispens and R2/23, two prototype vaccine strains of serotype 1 Marek’s disease virus. Avian Diseases, 39, 269–284. doi: 10.2307/1591868
- Witter, R.L., Li, D., Jones, D., Lee, L.F. & Kung, H.-J. (1997). Retroviral insertional mutagenesis of a herpesvirus: A Marek’s disease virus mutant attenuated for oncogenicity but not for immunosuppression or in vivo replication. Avian Diseases, 41, 407–421. doi: 10.2307/1592197
- Witter, R.L., Nazerian, K., Purchase, H.G. & Burgoyne, G.H. (1970). Isolation from turkeys of a cell-associated herpesvirus antigenically related to Marek’s disease virus. American Journal Veterinary Research, 31, 525–538.
- Witter, R.L., Sharma, J.M. & Fadly, A.M. (1980). Pathogenicity of variant Marek’s disease virus isolants in vaccinated and unvaccinated chickens. Avian Diseases, 24, 210–232. doi: 10.2307/1589781
- Zerbino, D.R. & Birney, E. (2008). Velvet: algorithms for de novo short read assembly using de Bruijn graphs. Genome Research, 18, 821–829. doi: 10.1101/gr.074492.107
- Zhang, Z. & Cui, Z. (2005). Isolation of recombinant field strains of Marek’s disease virus integration with reticuloendotheliosis virus genome fragments. Science in China. Series C, Life Sciences, 48, 81–88. doi: 10.1360/03yc0270