ABSTRACT
A quantitative PCR (qPCR) able to differentiate between field Mycoplasma synoviae and MS-H vaccine strain was developed, validated and evaluated. It was developed using nucleotide differences in the obg gene. Analytical specificity and sensitivity assessed using DNA from 194 M. synoviae field samples, three different batches of MS-H vaccine and from 43 samples representing four other avian Mycoplasma species proved to be 100%. The detection limit for field M. synoviae and MS-H vaccine strain was 102-3 and 102 colony-forming units PCR equivalents/g trachea mucus, respectively. The qPCR was able to detect both, field M. synoviae and MS-H vaccine strain in ratios of 1:100 determined both using spiked and field samples. One hundred and twenty samples from M. synoviae-infected non-vaccinated birds, 110 samples from M. synoviae-vaccinated birds from a bird experiment and 224 samples from M. synoviae negative (serology and PCR) birds were used to determine the relative sensitivity and specificity using a previously described M. synoviae PCR as reference. The relative sensitivity and specificity for field M. synoviae were 95.0% and 99.6%, respectively, and 94.6% and 100% for the MS-H-live vaccine, respectively. Field validation and confirmation by multi locus sequence typing revealed that the qPCR correctly distinguished between MS-H and field M. synoviae. Evaluation of the differentiating M. synoviae qPCR in three commercial flocks suggested transmission of MS-H-live vaccine from vaccinated to non-vaccinated flocks at the same farm. Furthermore, it showed evidence for the colonization with field M. synoviae in MS-H-vaccinated flocks.
Introduction
Mycoplasma synoviae has been described as the aetiological agent of airsacculitis (Kleven et al., Citation1972; Yoder et al., Citation1977), infectious synovitis (Anderson et al., Citation1956; Morrow et al., Citation1990; Landman & Feberwee, Citation2001; Kang et al., Citation2002) and eggshell apex abnormalities (Feberwee et al., Citation2009a, b; Feberwee & Landman, Citation2010). It may cause significant economic losses in commercial poultry.
In a number of countries such as the USA and UK, voluntary M. synoviae control and eradication programmes have been operating for a long time. In the Netherlands, a M. synoviae control and eradication programme was enforced by decree in 2013 (Productschap voor Pluimvee en Eieren, Citation2012, Citation2013; Kamp, Citation2014). The Dutch M. synoviae control and eradication programme is based on the early detection of infected flocks, enabled through regular monitoring and control measures. The latter are aimed at the prevention of the vertical and horizontal transmission of M. synoviae. Voluntary slaughter of infected grandparent stock, which despite its voluntary character is performed consistently will prevent its vertical spread. Slaughter of parent stock to eliminate M. synoviae is currently not economically feasible due to the high M. synoviae prevalence. Whenever elimination of infected flocks is not economically sustainable, antibiotic treatment and vaccination programmes may be used to mitigate/prevent the impact of clinical disease. Improvement of hygiene, including “practical channelling” aimed at separating M. synoviae-infected flocks and their eggs from non-infected counterparts, is implemented to avoid the horizontal transmission of M. synoviae (Landman, Citation2014).
Live M. synoviae vaccine (Vaxsafe® MS) based on the attenuated MS-H strain was introduced in Europe in 2012. This vaccine was increasingly used in our country jeopardizing M. synoviae seromonitoring, as it induces humoral antibodies, which are indistinguishable from those induced by infection with field M. synoviae by the currently used serological tests. Although MS-H vaccination may help to reduce/prevent clinical signs, it does not prevent infection with field M. synoviae (Feberwee et al., Citation2009a; Feberwee et al., Citation2017). Therefore, in order to be able to monitor vaccinated flocks for freedom of infection and be able to continue our M. synoviae control and eradication programme, there was an urgent need for a differentiation of infected from vaccinated animals (DIVA) test for M. synoviae.
The MS-H vaccine strain has a temperature-sensitive (ts+) phenotype, which was obtained by mutagenesis of a temperature-insensitive (ts−) M. synoviae field strain (Markham et al., Citation1998a, b, c; Morrow et al., Citation1998). However, reversion from a ts+ to ts− phenotype occurs under favourable conditions (Markham et al., Citation1998c) making temperature-dependent mycoplasma culture to distinguish the MS-H vaccine strain from field M. synoviae unreliable. Therefore, molecular techniques for typing M. synoviae isolates were developed.
Recently, polymorphisms in the obg gene of the MS-H-live vaccine strain have been described, which may represent important targets for the development of diagnostic differentiating PCR tests (Shahid et al., Citation2013a, b; Shahid et al., Citation2014; Kreizinger et al., Citation2015).
Here we describe the development, validation and evaluation of a quantitative PCR (qPCR) able to differentiate between the MS-H-live vaccine strain and field M. synoviae based on the obg gene. It was developed and validated using trachea swabs from chickens experimentally or naturally infected with M. synoviae.
Materials and methods
Mycoplasma strains and field samples
In this study sequence data from the obg gene and DNA from 194 M. synoviae samples (cloned pure cultures of M. synoviae field strains, three vaccine strains each originating from a different batch of MS-H-live vaccine and tracheal swab samples) were used for the development and validation of the differentiating M. synoviae qPCR. These samples originated from both chickens and turkeys, from different categories of poultry (broiler and layer reproduction stock, commercial layers, meat turkeys and turkey reproduction stock) and were collected between and including the years 1976 and 2014. In addition, they were obtained from different organs (trachea, inflamed joints or from the oviduct of chickens producing eggs with eggshell apex abnormalities). Field M. synoviae samples originated from Australia (n = 4), France (n = 10), Germany (n = 50), Greece (n = 10), Israel (n = 11), Italy (n = 13), Japan (n = 4), Poland (n = 3), Portugal (n = 4), Saudi Arabia (n = 2), Slovenia (n = 5), Spain (n = 9), the Netherlands (n = 53), UK (n = 8) and the USA (n = 8). In addition, 10 Mycoplasma meleagridis, 30 Mycoplasma gallisepticum, two Mycoplasma iowae and one Mycoplasma gallinaceum field samples were included in this study. Details regarding the samples are presented in .
Table 1. Background information of M. synoviae field isolates (n = 194), MS-H-live vaccine (n = 3) and other avian mycoplasma strains (n = 43) used for the development and validation of the differentiating M. synoviae qPCR.
Sequencing and development of the differentiating M. synoviae qPCR
Nucleotide differences within the obg gene between the MS-H vaccine strain and M. synoviae field isolates of which several had already been described by Shahid et al. (Citation2014) were used for the development of a differentiating M. synoviae qPCR for direct use on trachea swabs. To confirm the presence of these nucleotide differences in a large set of 194 M. synoviae field samples (Dijkman et al., Citation2016), two separate PCRs were used to amplify the obg gene. Target and primer sequences were based on the M. synoviae strain MS53 complete genome sequence (acc. no. AE017245). Set 1 consisted of primer F1 (5′-GGGATGATTTCGTTTCGCCG-3′) and R1 (5′-TCCTTTACCTAGTGATGCGCC-3′) targeting bases 180903–181517 and set 2 consisted of primer F2 (5′-ATTGCGGATCTTCCTGGACT-3′) and R2 (5′-TCTTCCTCCCATTCAAATTTATGTT-3′) targeting bases 181470–182113.
The obg gene was amplified from the extracted DNA using 10 pmol of mentioned primers. The PCR was performed using the Kapa Sybr Green mix (Kapa Biosystems, Woburn, MA, USA) on a LightCycler 480 (Roche Applied Science, Basel, Switzerland) under the following conditions: initial denaturation/enzyme activation for 5 min at 95°C followed by 35 cycles of denaturation at 95°C for 1 min and primer annealing and extension at 60°C for 1 min. PCR products were subsequently subjected to Sanger sequencing (Baseclear, Leiden, the Netherlands) using both the forward and reverse primers.
Consensus sequences were assembled using both forward and reverse sequences, and were compared using MEGA6.0 (Tamura et al., Citation2013). Specific nucleotide changes detected only in the MS-H-live vaccine strain and not in any of the M. synoviae field isolates were used for the development of a differentiating M. synoviae qPCR (commercially available as Kylt® MS-H DIVA, produced by AniCon Labor GmbH, Hoeltinghausen, Germany). Sequences were compared to Genbank using a BLAST search for in silico specificity testing of the primers and probes used in this assay.
In vitro validation of the differentiating M. synoviae qPCR
Validation of the differentiating M. synoviae qPCR was performed on the Applied Biosystems 7500 Real-Time PCR System (Applied Biosystems, Foster City, CA, USA) using the following steps: initial denaturation for 3 min at 95°C followed by 42 cycles of denaturation for 10 s at 95°C and annealing and extension for 30 s at 60°C. Data were analysed (with 5-carboxy-X-rhodamine (ROX) Passive Reference Dye) using a normalized reporter (Delta Rn) threshold of 0.2 for both the 6-carboxyfluorescein (6-FAM) signal and the cyanine 5 (Cy5) signal. PCR cycles 3–15 were used for baseline normalization (manual baseline). Samples with cycle threshold (Ct)-values <40 were considered positive and with Ct-values >40 as negative.
Analytical sensitivity and specificity
Analytical specificity and sensitivity of the qPCR were examined using different mycoplasma samples (both, pure cultures and field samples) mentioned in .
Detection range, limit of detection, PCR efficiency and ability to detect both field M. synoviae and MS-H vaccine strain
The detection range, limit of detection (LOD) and efficiency of the qPCR were examined using trachea mucus suspensions spiked with known concentrations of either an arthropathic M. synoviae strain (chicken/NL/Dev/801979Rob/00) (Landman & Feberwee, Citation2001) or MS-H vaccine (Vaxsafe® MS; Bioproperties Pty Ltd, Glenorie, Australia). Trachea mucus was obtained from 16 specified pathogen-free (SPF) White Leghorns using a single swab (Dryswab MW142, Medical Wire, Corsham, UK) per bird. Each swab was then rinsed in 250 μl phosphate-buffered saline solution. The obtained mucus suspensions were spiked with decimally decreasing concentrations of either M. synoviae strain, which ranged from 107 colony forming units (CFU) to and including 100 CFU. Hereto, 20 µl containing inoculum with a given concentration of M. synoviae was added to 230 µl mucus suspension. The inocula for spiking were prepared as described (Feberwee et al., Citation2005) and counted according to the International Organization for Standardization (ISO21528-Citation1, Citation2004). Briefly, for both the M. synoviae field strain and the MS-H vaccine strain decimal dilutions (n = 8 for field M. synoviae and n = 8 for MS-H vaccine strain) of a M. synoviae Mycoplasma Experience (ME) broth (Avian Mycoplasma Broth, Mycoplasma Experience, Reigate, UK) culture that had been incubated for two days at 37°C, were made in duplicate (10–1 up to and including 10–7 and a negative control). Twenty microlitres of each dilution were plated on ME agar, which was incubated at 37°C in a humid environment until mycoplasma colonies were visible for counting. Subsequently, the mycoplasma concentration was calculated. The inocula used for spiking were also used as PCR quantification standards.
In addition, the ability of the qPCR to detect both field M. synoviae as well as MS-H vaccine strain in the same sample was evaluated by performing checkerboard titrations of the M. synoviae field strain and the MS-H vaccine strain. That is, by mixing equal volumes of trachea mucus suspensions containing increasing/decreasing concentrations of either strain in the opposite direction. For example, adding 1 volume with 104 CFU field M. synoviae strain to 1 volume with 106 CFU MS-H resulted in a ratio of 1:100. The concentrations used for preparing these mixtures also ranged from 107 to 100 CFU.
Extraction of DNA was performed with the Kapa Express Extract kit as described previously (Dijkman et al., Citation2013). PCR efficiency was expressed as 10(−1/slope). The quantitative results were expressed as CFU PCR equivalents (CPE)/g trachea mucus. In order to calculate the CPE/g trachea mucus, the average trachea mucus uptake by the swab type used was determined by weighing 40 single swabs before and after sampling using a calibrated analytical balance (accuracy by uncertainty of measurement: ± 0.0089 mg). The average uptake was 18.3 ± 5.9 mg. Also it was assumed that all DNA present in the swab was rinsed out during washing.
Repeatability and intra-laboratory reproducibility
Data from the LOD experiments were used to determine the repeatability and intra-laboratory reproducibility of the qPCR. Samples yielding high (107 CFU/g trachea mucus), medium (105 CFU/g trachea mucus) and low (103 CFU/g trachea mucus) concentrations of M. synoviae field strain or MS-H-live vaccine were used. These samples were obtained from culture in ME broth, subsequent CFU countings and 10-fold dilutions.
In vivo validation of the differentiating M. synoviae PCR
Relative sensitivity and specificity
The relative sensitivity was determined using MS-H-positive reference trachea swab samples (n = 110) derived from SPF chickens and trachea swab samples (n = 120) (Dryswab MW142, Medical Wire, Corsham, UK) from non-vaccinated commercial chickens infected with field M. synoviae (ELISA and qPCR (described by Raviv & Kleven, Citation2009) positive). The relative specificity was determined using M. synoviae negative reference trachea swab (Dryswab MW142) samples derived from SPF birds (n = 118) and trachea swab samples (n = 106) from non-vaccinated commercial chickens not infected with field M. synoviae (ELISA and qPCR negative). Trachea swab samples were individually analysed using the differentiating M. synoviae qPCR. The results obtained were compared with those of a TaqMan® PCR (Raviv & Kleven, Citation2009), which was used as the reference method.
Confirmation of differentiating M. synoviae qPCR results by multi locus sequence typing
Additionally, trachea swabs (Dryswab MW142) from broiler breeders (n = 32 pools of six swabs) and layers (n = 8, individual swabs) housed on Dutch poultry farms were analysed using the differentiating M. synoviae qPCR, after which these samples were subjected to M. synoviae multi locus sequence typing (MLST) as described elsewhere (Dijkman et al., Citation2016). Details regarding these samples are presented in .
Table 2. Results of the field validation of the differentiating M. synoviae qPCR and of MLST of trachea mucus samples from broiler breeders (pooled swabs) and layers (individual swabs).
Field evaluation
The differentiating M. synoviae qPCR was used for the analysis of trachea swabs (Dryswab MW142) obtained from three separate commercial chicken flocks housed on different farms and not linked epidemiologically to each other. On all three farms a national controlled standard biosecurity programme was included (http://www.avined.nl), which aims to reduce the spread or introduction of infectious agents. Blood samples for the detection of M. synoviae antibodies were also collected from these flocks.
Flock I (broiler breeders). This was a flock of broiler breeders housed on farm K, which had been reared on rearing farm H. An overview regarding the background information and the sampling scheme of both farms is given in .
Figure 1. Sampling scheme, housing and results of the M. synoviae RPA test and the differentiating M. synoviae qPCR of a non-M. synoviae-vaccinated broiler breeder flock (farm K) and the rearing flock of origin (farm H). At the rearing farm, birds kept in two adjoining houses (5 and 6) had been vaccinated with MS-H at 10 weeks of age. At approximately 18 weeks of age, birds from rearing houses 1, 2, 3 and 4 were transferred to farm K, while those from houses 5 and 6 were transferred to another production farm. Shortly before transfer, the non-vaccinated birds showed negative RPA test results, while the vaccinates had seroconverted. All broiler breeders of farm K sampled at 23 and 25 weeks had seroconverted also, after which trachea swabs were collected for analysis with the differentiating M. synoviae qPCR. The latter showed the presence of MS-H, field M. synoviae or mixed infections in a substantial number of trachea mucus samples. These results suggest that spread of MS-H had occurred at the rearing farm, while birds of farm K also had been infected with field M. synoviae. The number of birds per house at the rearing farm, the number of blood samples for serology and the number of trachea swabs for qPCR analysis are indicated.
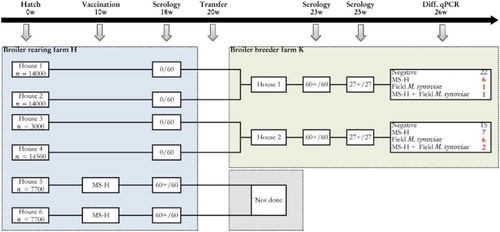
Farm K had two houses. Thirty trachea swab samples were taken per house at 26 weeks of age and analysed individually with the differentiating M. synoviae qPCR. Blood samples were also collected at 23 weeks (60 per house) and 25 weeks of age (27 samples per house) for M. synoviae serology. These birds had not been vaccinated with MS-H vaccine.
The rearing farm of origin had six houses. The birds reared for farm K had been kept in houses 1, 2, 3 and 4, while in houses 5 and 6 MS-H-vaccinated birds had been housed. The vaccination had been performed at 10 weeks of age and the birds were transferred elsewhere for production at the end of the rearing period.
Flock II (broiler breeders). This flock of broiler breeders was housed on farm B, and had been reared on rearing farm G. An overview regarding the background information and the sampling scheme of both farms is given in .
Figure 2. Sampling scheme, housing and results of the M. synoviae RPA test and the differentiating M. synoviae qPCR of a non-M. synoviae-vaccinated broiler breeder flock (farm B) and the rearing flock of origin (farm G). At the rearing farm, birds kept in two adjoining houses (3 and 4) had been vaccinated with MS-H at 10 weeks of age. At approximately 18 weeks of age, birds from rearing houses 1 and 2 were transferred to farm B, while those from houses 3 and 4 were transferred to another production farm. Shortly before transfer, the non-vaccinated birds showed negative RPA test results, while the vaccinates had seroconverted. All broiler breeders of farm B sampled at 24 and 31 weeks had seroconverted also, after which trachea swabs were collected for analysis with the differentiating M. synoviae qPCR. The latter showed the presence of MS-H in a substantial number of trachea mucus samples. These results suggest that spread of MS-H had occurred at the rearing farm. The number of birds per house at the rearing farm, the number of blood samples for serology and the number of trachea swabs for qPCR analysis are indicated.
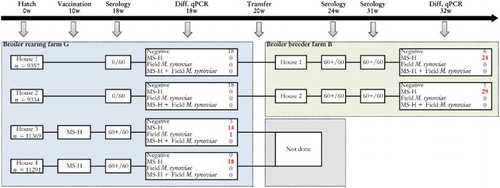
Farm B had two houses also. Eighteen trachea swab samples were taken per house at 18 and 32 weeks of age, and analysed individually with the differentiating M. synoviae qPCR. Blood samples (60 per house) were also collected at 18 weeks, 24 weeks and 31 weeks of age for M. synoviae serology. These birds had not been vaccinated with MS-H vaccine. The rearing farm of origin had four houses. The birds reared for farm B had been kept in houses 1 and 2, while in houses 3 and 4 MS-H-vaccinated birds had been housed. The vaccination had been performed at 10 weeks of age and the birds were transferred elsewhere for production at the end of the rearing period.
Flock III (layers). This flock was housed on two layer farms (O and D) and had been reared on rearing farm B in house 1. An overview regarding the background information and the sampling scheme of the three farms is given in .
Figure 3. Sampling scheme, housing and results of the M. synoviae RPA test and the differentiating M. synoviae qPCR of a non-M. synoviae-vaccinated layer flock (farm O), a vaccinated layer flock (farm D) and the rearing flock of origin (farm B). At farm B, rearing layers were kept in one house with two sections (1a and 1b) separated only by a wire mesh. The birds in section 1b had been vaccinated with MS-H at 11 weeks of age. At approximately 18 weeks of age, birds from rearing house 1a were transferred to farm O, while those from house 1b were transferred to a layer farm D. The latter farm was located at 25 meters distance from another layer farm infected with field M. synoviae. Shortly before transfer, both the non-vaccinated as well as the vaccinates were positive for MS-H in the differentiating M. synoviae qPCR, suggesting that spread of MS-H had occurred at the rearing farm. All layers sampled on layer farm O (21 weeks of age) and D (21, 25 and 29 weeks of age) and the neighbouring farm (61, 68 and 72 weeks of age) had seroconverted, after which trachea swabs were collected for analysis with the differentiating M. synoviae qPCR. The latter showed the presence of MS-H in a substantial number of trachea mucus samples from farm O and D. At week 72 of age, field M. synoviae was also detected in farm D indicating that birds had been infected with M. synoviae field strain. In trachea mucus samples collected from birds at the neighbouring farm only field M. synoviae was detected using the differentiating M. synoviae qPCR. The number of birds per house at the rearing farm, the number of blood samples for serology and the number of trachea swabs for qPCR analysis are indicated.
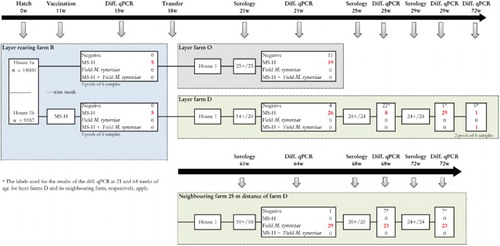
On rearing farm B, an MS-H vaccinated (at 11 weeks of age) and a non-vaccinated flock were reared in the same house (unit a and b, respectively) only separated by a wire mesh. Prior to transfer of the flock to two different layer farms (farms O and D), 30 trachea swab samples per house were taken at 15 weeks of age, pooled per six samples and analysed with the differentiating M. synoviae qPCR.
The non-vaccinated flock (house 1a) was transferred to layer farm O (one house) at 18 weeks of age. At 21 weeks of age, 30 trachea samples were collected and analysed individually with the differentiating M. synoviae qPCR and 25 blood samples were taken for M. synoviae serology.
The MS-H-vaccinated flock (house 1b) was transferred to a free range layer farm D (one house) at 18 weeks of age. A neighbouring layer farm was situated at 25 m. At 21 weeks, 25 weeks and 29 weeks of age, 30 trachea swab samples were collected and analysed individually with the differentiating M. synoviae qPCR. At the same time points 24 blood samples were taken for M. synoviae serology. At 72 weeks of age, the end of the production period, 12 trachea samples (two pools of six swabs) were taken for analysis with the differentiating M. synoviae qPCR.
The flock on the neighbouring farm was 61 weeks of age at first sampling (at which time birds of layer farms O and D were 21 weeks of age), not MS-H vaccinated and had free range. From this flock, at 64 week, 68 weeks and 72 weeks of age 30 trachea samples were taken and analysed individually with the differentiating M. synoviae qPCR. In addition, 10–24 blood samples were taken for M. synoviae serology at 61 weeks, 68 weeks and 72 weeks of age.
Mycoplasma culture and DNA extraction
Mycoplasma culture was performed on ME agar (Avian Mycoplasma Solid Medium) as described earlier (Feberwee et al., 2005). In brief, samples were plated on ME agar plates and subsequently incubated at 37°C in a humid environment and examined for colony growth every 2–3 days up to 28 days. One separate colony was plated out on fresh ME agar, and from ME agar with colonies two pieces of approximately 1 cm × 0.5 cm were removed using a sterile scalpel blade and transferred to 5 ml ME broth (Avian Mycoplasma Liquid Medium; Mycoplasma Experience), which was then incubated at 37°C until the colour changed from red to orange. Broth cultures were subsequently used for DNA extraction. DNA was extracted from the mycoplasma cultures using the QiaAmp DNA mini kit (Qiagen, Hilden, Germany) according to the manufacturer’s instructions. In short, M. synoviae cultures were concentrated by centrifugation, pellets were suspended in 200 µl phosphate-buffered saline and DNA was extracted using the QiaAmp DNA mini kit according to the cell culture protocol.
Extraction of DNA from tracheal swabs was performed using the Kapa Express Extract kit (Kapa Biosystems, Wilmington, NC, USA). Individual swabs were washed in 250 µl 0.5 × Kapa lysis mix containing 12.5 µl Kapa Express Extract buffer (10×), 3 µl Kapa Express Extract enzyme mix and 234.5 µl of sterile water. In case of pooled samples, the individual swabs were placed in a single tube containing 500 µl of the previously described 0.5 × Kapa lysis mix. The samples (both individual and pooled samples) were incubated at 70°C for 10 min followed by incubation at 95°C for 5 min. After centrifugation at 10,000 × g for 5 min, the samples were ready for PCR analysis.
M. synoviae rapid plate agglutination test
Sera were analysed using in the rapid plate agglutination (RPA) test (Soleil, Veterinary Labor LTD, Hungary) within 24 h after collecting the blood samples. Samples were examined by mixing 25 µl of 1:2 in phosphate-buffered saline diluted sera with 25 µl of M. synoviae antigen on a white Perspex plate. The plate was then gently swirled and visible agglutination reactions that occurred within 2 min were considered positive.
Statistical analysis
The sensitivity and specificity of the differentiating M. synoviae qPCR for swab samples were calculated using the binary classification test (Altman & Bland, Citation1994). The proportion of agreement between the differentiating M. synoviae qPCR and the M. synoviae TaqMan® PCR described by Raviv & Kleven (Citation2009) was determined using the Kappa agreement test (Landis & Koch, Citation1977). Strength of agreement was indicated as follows: <0 very poor, 0–0.20 poor, 0.21–0.40 fair, 0.41–0.60 moderate, 0.61–0.80 good and 0.81–1.0 very good. Repeatability and intra-laboratory reproducibility of the differentiating M. synoviae qPCR for both field M. synoviae and MS-H-live vaccine strain were calculated according to ISO standard 5725 (ISO5725, Citation1994) guidelines and were presented as coefficient of variation CVr(%) and CVR(%) for repeatability and intra-laboratory reproducibility, respectively.
Results
In vitro validation of the differentiating M. synoviae qPCR
Analytical sensitivity and specificity
A BLAST search revealed that the primers and probes selected were specific for M. synoviae and no potential cross-reactivity with other mycoplasma species or micro-organisms commonly found in chicken was expected based on this in silico analysis (data not shown). In addition, field samples were tested.
All field samples (n = 194) were positive for field M. synoviae using the differentiating M. synoviae qPCR. Other mycoplasma species or the MS-H vaccine strain were not found resulting in an analytical sensitivity for field M. synoviae of 100% (95% CI: 98.1–100%) and an analytical specificity for field M. synoviae of 100% (95% CI: 91.8–100%). The three vaccine strains originating from different batches of MS-H-live vaccine were detected as MS-H vaccine resulting in an analytical sensitivity for MS-H of 100% (95% CI: 29.2–100%). None of the M. synoviae field isolates, M. gallisepticum, M. meleagridis, M. iowae or M. gallinaceum isolates, were detected as MS-H-live vaccine using the differentiating M. synoviae qPCR resulting in an analytical specificity for MS-H of 100% (95% CI: 98.5–100%).
Detection range, LOD, PCR efficiency and ability to detect both, field M. synoviae and MS-H vaccine strain
The 10-fold dilutions in swab extracts were tested in triplicate on three different days using the differentiating M. synoviae qPCR. Detection of the PCR ranged from 102 to 107 CPE/g trachea mucus. The detection limit of the differentiating M. synoviae qPCR was 102-3 CPE/g trachea mucus for the M. synoviae field strain and 102 CPE/g trachea mucus for the MS-H-live vaccine strain using a Ct cut-off value of 40. Based on the results of the 10-fold dilutions, the mean PCR efficiencies were 92% and 97% for the M. synoviae field strain and MS-H-live vaccine strain, respectively. The checkerboard titrations demonstrated that the differentiating M. synoviae qPCR was able to detect both field M. synoviae and MS-H-live vaccine strain in the same sample in ratios of 1:100 and 100:1, respectively.
Repeatability and intra-lab reproducibility
The repeatability of the differentiating M. synoviae qPCR for field M. synoviae was 0.93%, 1.12% and 0.99% for the high, medium and low concentration (CFU/g trachea mucus), respectively. The intra-laboratory reproducibility of the differentiating M. synoviae qPCR for field M. synoviae was 0.93%, 1.12% and 0.99% for the high, medium and low concentration, respectively. For the MS-H-live vaccine strain, the repeatability of the differentiating M. synoviae qPCR was 1.14%, 1.00% and 0.52% for the high, medium and low concentration, respectively. The intra-laboratory reproducibility of the differentiating M. synoviae qPCR for MS-H-live vaccine strain was 1.31%, 1.46% and 1.46% for the high, medium and low concentration, respectively.
In vivo validation of the differentiating M. synoviae qPCR
Relative sensitivity and specificity for swab samples
Compared to the results of the M. synoviae TaqMan® PCR (Raviv & Kleven, Citation2009), the differentiating M. synoviae qPCR was able to detect 114 of 120 M. synoviae positive field samples resulting in a relative sensitivity of 95% (95% CI: 89.4–98.1) for field M. synoviae. The relative specificity of the differentiating M. synoviae qPCR for field M. synoviae was 99.6% (95% CI: 97.5–99.9) as 223 of 224 samples, which were M. synoviae negative using the TaqMan® PCR, were also negative using the differentiating M. synoviae qPCR. For detection of field M. synoviae, this resulted in a very good agreement Kappa value 0.95 (95% CI: 0.92–0.99) using the TaqMan® PCR as the reference method.
Regarding the detection of MS-H-live vaccine strain, the relative sensitivity of the differentiating M. synoviae qPCR, again comparing it to the M. synoviae TaqMan® PCR, was 94.6% (95% CI: 88.5–98.0) as 104 of 110 positive samples were detected. The relative specificity was 100% (95% CI: 98.3–100.0) as 212 of 212 samples were negative. The Cohen’s Kappa value was 0.96 (95% CI: 0.93–0.99) indicating a very good agreement.
Confirmation of differentiating M. synoviae qPCR results by MLST
Analysis of 40 field samples (trachea swabs) using the differentiating M. synoviae qPCR, showed that the MS-H-live vaccine strain was present in 12 samples, field M. synoviae in 13 samples and both the MS-H-live vaccine strain and field M. synoviae in 15 samples. MS-H-live vaccine strain was detected in amounts ranging from 3.51 to 7.35 log10 CPE/g trachea mucus, while field M. synoviae was found in concentrations ranging from 1.55 to 6.98 log10 CPE/g trachea mucus.
MLST sequencing resulted in reliable typing results for 35 of the 40 field samples. In a phylogenetic tree of the concatenated sequences of the MLST alleles for the 35 field samples and the MS-H vaccine strain is presented. Genotyping by MLST revealed that the 12 samples in which only the MS-H-live vaccine strain was detected (indicated as ♦) belonged to sequence type (ST) 2, which is identical to that of the MS-H-live vaccine strain (Dijkman et al., Citation2016). The 13 samples in which only field M. synoviae was detected (indicated as •) were typed as ST55 (n = 3), ST56 (n = 8) and ST57 (n = 2). Furthermore, as all five MLST alleles of these STs differ from those of the MS-H-live vaccine strain, these isolates were regarded as field M. synoviae. In 15 field samples, both the MS-H-live vaccine strain and field M. synoviae were detected (indicated as □). In five of these samples, sequencing resulted in mixed traces hindering reliable typing by MLST (). In the other 10 samples, only the most abundant strain could be typed. In nine of 10 samples the MS-H-live vaccine strain was the most abundant strain based on the quantitative data obtained using the differentiating M. synoviae qPCR. This was confirmed by the typing results where the detected isolate belonged to ST2 (MS-H). In the remaining sample field M. synoviae was found. Based on MLST this isolate was found to belong to ST56.
Figure 4. Phylogenetic tree of 35 M. synoviae field isolates and the MS-H vaccine strain including its re-isolates constructed from the concatenated sequences of upgA, uvrA, lepA, nanA and ruvB genes, which are used for the M. synoviae MLST. Samples in which only MS-H (♦) or field M. synoviae (•) or both, MS-H and field M. synoviae (□) were detected by the differentiating M. synoviae qPCR are indicated with the corresponding symbols. Sample identification (indicated by MS and a figure or additional text) corresponds to the sample identification used in . ST sequence type.
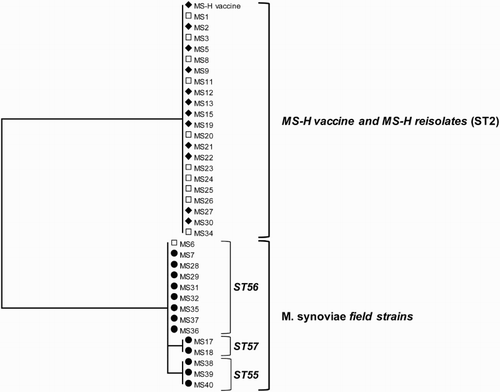
Field evaluation
Flock I (broiler breeders). The results of serology and qPCR of flock I are presented in . At the rearing farm chickens vaccinated with MS-H had seroconverted at 18 weeks of age, while the non-vaccinated birds had not. All broiler breeders of production farm K sampled at 23 and 25 weeks had seroconverted also.
At 26 weeks of age trachea swab samples of the broiler breeders analysed using the differentiating M. synoviae qPCR showed MS-H, field M. synoviae or both, field M. synoviae as well as the MS-H vaccine strain in a substantial number of samples (). In the qPCR results for both houses of farm K are given. The mean M. synoviae concentrations found in birds of house 1 were 4.8 log10 CPE/g trachea mucus for both field M. synoviae and MS-H vaccine strain. The mean M. synoviae concentrations found of birds in house 2 were 4.2 log10 CPE/g trachea mucus and 5.5 log10 CPE/g trachea mucus for field M. synoviae and the MS-H vaccine strain, respectively.
Figure 5. Quantitative results of the differentiating M. synoviae qPCR performed on trachea swabs of birds from houses 1 and 2 of the broiler breeder production farm K. Field M. synoviae (◊), MS-H (♦) and mean quantitative results for field M. synoviae (□) and MS-H (▪) are indicated.
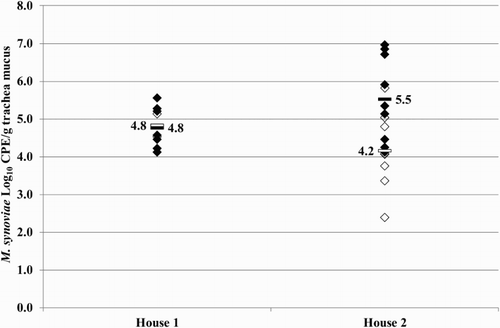
Flock II (broiler breeders). The results of serology and qPCR of flock II are presented in . At the rearing farm chickens vaccinated with MS-H had seroconverted at about 18 weeks of age, while the non-vaccinated birds had not. M. synoviae field strain was detected in house 3 of the rearing farm. However, no follow-up was possible for the birds that were housed in houses 3 and 4 as they were shipped to a production farm elsewhere.
All broiler breeders of production farm B sampled at 24 and 31 weeks had seroconverted also.
At 32 weeks of age, trachea swab samples of the broiler breeders analysed using the differentiating M. synoviae qPCR showed the presence of MS-H in a substantial number of samples (). In the qPCR results for both houses of farm B are given. The mean MS-H concentrations found in birds of houses 1 and 2 were 5.2 log10 CPE/g trachea mucus and 6.3 log10 CPE/g trachea mucus, respectively.
Figure 6. Quantitative results of the differentiating M. synoviae qPCR performed on trachea swabs of birds from broiler breeder farm B. MS-H (♦) and mean quantitative results (▪) are indicated.
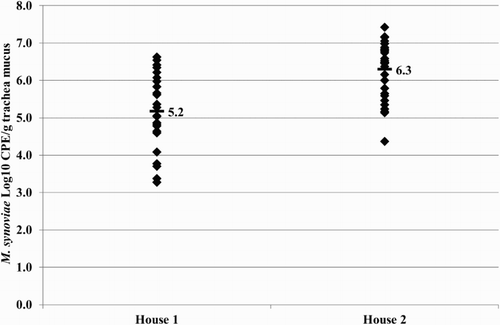
Flock III (layers). The results of serology and qPCR of flock III are presented in . At the rearing farm both MS-H-vaccinated and non-vaccinated birds were positive for MS-H by the differentiating M. synoviae qPCR at 15 weeks of age. All layers of production farm O and D and the neighbouring farm had seroconverted at 21 weeks, 25 weeks and 61 weeks of age, respectively.
At 21 weeks of age, trachea swab samples of the layers from farm O analysed using the differentiating M. synoviae qPCR showed MS-H in a substantial number of samples. At 21 weeks, 25 weeks and 29 weeks of age trachea swab samples from farm D analysed using the differentiating M. synoviae qPCR showed MS-H vaccine in a substantial number of samples (). At 64 weeks, 68 weeks and 72 weeks of age, trachea swab samples from the neighbouring farm analysed using the differentiating M. synoviae qPCR showed field M. synoviae in a substantial number of samples (). In the qPCR results for farm D and the neighbouring farm are given for trachea swab samples collected at three different time points (17 July, 15 August and 13 September 2013). The mean M. synoviae concentrations found in birds of the neighbouring farm were 6.1 log10 CPE/g trachea mucus, 5.0 log10 CPE/g trachea mucus and 5.7 log10 CPE/g trachea mucus for field M. synoviae at 64 weeks, 68 weeks and 72 weeks of age, respectively. The mean M. synoviae concentrations found in birds in farm D were 6.9 log10 CPE/g trachea mucus, 4.5 log10 CPE/g trachea mucus and 6.3 log10 CPE/g trachea mucus for the MS-H vaccine strain at 21 weeks, 25 weeks and 29 weeks of age, respectively. Finally, at 72 weeks of age trachea swab samples analysed using the differentiating M. synoviae qPCR showed both MS-H and field M. synoviae.
Figure 7. Quantitative results of the differentiating M. synoviae qPCR performed on trachea swabs of birds from layer farm D (MS-H vaccinated (MS-H vac)) and the neighbouring layer farm (non-vaccinated (non-vac)) at three different time points. Field M. synoviae (◊), MS-H (♦) and mean quantitative results for field M. synoviae (□) and MS-H (▪) are indicated.
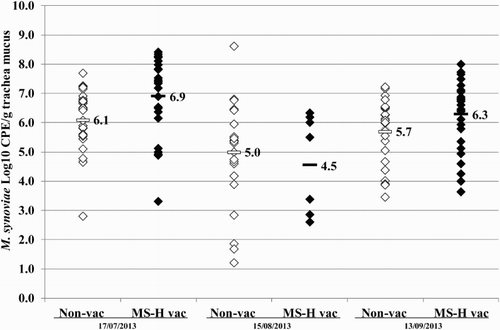
Discussion
Recently, it was shown that the MS-H vaccine strain could be distinguished from most M. synoviae field isolates by a nested-obg PCR-HRM assay (Shahid et al., Citation2014), making this gene a suitable candidate for the differentiation of MS-H from field M. synoviae. However, as mentioned by the authors, evaluation of the full potential of the assay for direct examination of samples from M. synoviae-infected birds should be performed, as it was used solely for the examination of M. synoviae clones after culture (Shahid et al., Citation2014).
Furthermore, Kreizinger and others described a melt-curve and agarose gel based Mismatch Amplification Mutation Assays (Kreizinger et al., Citation2015). Although this assay was able to distinguish between the ts+ MS-H vaccine strain, its ts– re-isolates and wild-type M. synoviae isolates could be used directly on tracheal swab samples; genotyping in case of mixtures of strains (the presence of both field M. synoviae as well as MS-H vaccine strain) was unreliable as ambiguous melting curves were obtained.
Therefore, in this study, single nucleotide polymorphisms (SNPs) in the obg gene of M. synoviae field isolates and MS-H-live vaccine were evaluated for their usefulness to differentiate field M. synoviae from the vaccine strain directly on trachea mucus samples taken using a sterile cotton swab, and also in the case of mixtures of both field M. synoviae and MS-H vaccine strain in the same sample. The differentiating M. synoviae qPCR was developed and validated using almost 200 M. synoviae trachea mucus samples with different background from 15 different countries. All M. synoviae field isolates were correctly identified. The MS-H-live vaccine strain and two of its re-isolates were identified correctly as well. This resulted in an analytical sensitivity of the assay for both the MS-H vaccine strain and field M. synoviae of 100%. Furthermore, none of the other mycoplasma species (M. gallisepticum, M. meleagridis, M. iowae and M. gallinaceum) were detected using this qPCR indicating an analytical specificity of 100%. The relative sensitivity of the assay for field M. synoviae determined using positive field samples and using the M. synoviae TaqMan® PCR described by Raviv and Kleven (Citation2009) as reference method was 95% and the relative specificity of the assay for field M. synoviae was 99.6%. For MS-H vaccine the relative sensitivity of the assay, determined using M. synoviae positive samples from a bird experiment, was 94.6% and the relative specificity was 100% indicating that the PCR was very sensitive, while no cross-reactivity was observed with field M. synoviae.
Whenever a differentiating PCR test, which targets a SNP, is widely used, additional assessment of results is required. Mutation of SNPs might occur and lead to false-positive and/or false-negative results. It is therefore essential to re-evaluate the results obtained with the differentiating M. synoviae qPCR on a regular basis by sequencing more genes of positive samples detected (both, MS-H and field M. synoviae positive). Samples that were found positive for MS-H by the differentiating M. synoviae qPCR were genotyped using MLST as ST 2 (Dijkman et al., Citation2016), to which MS-H and MS-H re-isolates belong. In contrast, samples that were found positive for field M. synoviae were genotyped by the MLST as ST55, ST56 or ST57. These STs are included in the dominant cluster of Dutch M. synoviae field strains (Dijkman et al., Citation2016).
It was difficult to detect different clones by MLST in samples with both the MS-H-live vaccine strain and field M. synoviae, as this often resulted in mixed sequence traces of which only the most abundant can be detected. The results of the confirmatory MLST sequencing show the restrictions of direct sequencing for strain differentiation in case of presence of both field M. synoviae and MS-H vaccine strain in the same sample and indicate that for the monitoring of M. synoviae-vaccinated flocks a differentiating M. synoviae qPCR is the method of choice.
Analysis of the 40 field samples used for confirmatory analysis by MLST and the field evaluation with the differentiating M. synoviae qPCR revealed that low concentrations of field M. synoviae could be detected. This was also the case in vaccinated flocks shedding MS-H, further demonstrating its ability to detect both field M. synoviae and MS-H vaccine strain in the same sample. DNA from both field M. synoviae and MS-H vaccine strain in individual samples detected by PCR has been demonstrated by our group in a vaccination-challenge study as well (Feberwee et al., Citation2017). Variation in the shedding levels of the MS-H vaccine () in the vaccinated birds of flock III (layers) with time was observed. However, an explanation for this variation in shedding levels could not be found.
The results of the field evaluation of the differentiating M. synoviae qPCR also suggest that the MS-H-live vaccine strain can spread from vaccinated to non-vaccinated birds within and between houses on the same farm. It was also shown that the concentration of MS-H in the trachea mucus of the birds that likely were vaccinated by spread ranged from 4.8 log10 to 6.3 log10 CPE/g trachea mucus, which was similar to that of MS-H-vaccinated birds (4.5 log10 to 6.9 log10 CPE/g trachea mucus).
The results showed that the MS-H vaccine can spread between houses on a poultry farm with a standard biosecurity programme aiming at the prevention of introduction or spread of poultry pathogens. The spread of the MS-H vaccine strain from vaccinated to non-vaccinated birds has been shown earlier to occur via direct contact experimentally (Markham et al., Citation1998a) and via indirect contact between houses of the same farm in the field (Markham et al., Citation1998c). The spread between poultry houses was explained by the introduction of MS-H-contaminated feathers, MS-H-contaminated manure and/or farm personnel handling MS-H-vaccinated birds prior to non-vaccinated counterparts (Markham et al., Citation1998c).
The horizontal spread of MS-H was not unexpected as live vaccines tend to spread (Gharaibeh et al., Citation2011), which can sometimes even be regarded as beneficial (Tannock & Shafren, Citation1994), however, this should be taken into account during the management of poultry flocks.
Acknowledgements
We thank Constance Reugebrink for her excellent technical assistance.
Disclosure statement
No potential conflict of interest was reported by the authors.
Additional information
Funding
References
- Altman, D.G. & Bland, J.M. (1994). Diagnostic tests. 1: sensitivity and specificity. British Medical Journal, 308, 1552. doi: 10.1136/bmj.308.6943.1552
- Anderson, G.C., Bletner, J.K., Munro, D.A., Olson, N.O. & Shelton, D.C. (1956). Studies of infectious synovitis in chickens. American Journal of Veterinary Research, 17, 747–754.
- Dijkman, R., Feberwee, A. & Landman, W.J.M. (2013). Validation of a previously developed quantitative polymerase chain reaction for the detection and quantification of Mycoplasma synoviae in chicken joint specimens. Avian Pathology, 42, 100–107. doi: 10.1080/03079457.2013.766669
- Dijkman, R., Feberwee, A. & Landman, W.J.M. (2016). Development and evaluation of a multi-locus sequence typing scheme for Mycoplasma synoviae. Avian Pathology, 45, 426–442. doi: 10.1080/03079457.2016.1154135
- Feberwee, A., Dijkman, R., Klinkenberg, D. & Landman, W.J.M. 2017. Quantification of the horizontal transmission of Mycoplasma synoviae in non-vaccinated and MS-H vaccinated layers. Avian Pathology, published online http://www.tandfonline.com/doi/full/10.1080/03079457.2017.1282602.
- Feberwee, A. & Landman, W.J.M. (2010). Induction of eggshell apex abnormalities in broiler breeder hens. Avian Pathology, 39, 133–137. doi: 10.1080/03079451003657637
- Feberwee, A., Mekkes, D.R., de Wit, J.J., Hartman, E.G. & Pijpers, A. (2005). Comparison of culture, PCR, and different serologic tests for detection of Mycoplasma gallisepticum and Mycoplasma synoviae infections. Avian Diseases, 49, 260–268. doi: 10.1637/7274-090804R
- Feberwee, A., Morrow, C.J., Ghorashi, S.A., Noormohammadi, A.H. & Landman, W.J.M. (2009a). Effect of a live Mycoplasma synoviae vaccine on the production of eggshell apex abnormalities induced by a M. synoviae infection preceded by an infection with infectious bronchitis virus D1466. Avian Pathology, 38, 333–340. doi: 10.1080/03079450903183652
- Feberwee, A., de Wit, J.J. & Landman, W.J.M. (2009b). Induction of eggshell apex abnormalities by Mycoplasma synoviae: field and experimental studies. Avian Pathology, 38, 77–85. doi: 10.1080/03079450802662772
- Gharaibeh, S., Laibinis, V., Wooten, R., Stabler, L. & Ferguson-Noel, N. (2011). Molecular characterization of Mycoplasma gallisepticum isolates from Jordan. Avian Diseases, 55, 212–216. doi: 10.1637/9526-091510-Reg.1
- ISO5725. (1994). Accuracy (trueness and precision) of measurement methods and result, part 2: basic methods for the determination of repeatability and reproducibility of a standard measurement methods. Geneva, Switzerland: International Organization for Standardization.
- ISO21528-1. (2004). Microbiology of food and animal feeding stuffs – horizontal methods for the detection and enumeration of Enterobacteriaceae – part 1: detection and enumeration by MPN technique with pre-enrichment. Geneva, Switzerland: International Organization for Standardization.
- Kamp, H.G.J. (2014). Regeling van de Minister van Economische Zaken van 10 december 2014, nr. WJZ/14139630, houdende wijziging van diverse regelingen in verband met de opheffing van de bedrijfslichamen en de overname van taken. In M.v.E. Zaken (Ed.). ‘s Gravenhage: Staatscourant.
- Kang, M.S., Gazdzinski, P. & Kleven, S.H. (2002). Virulence of recent isolates of Mycoplasma synoviae in turkeys. Avian Diseases, 46, 102–110. doi: 10.1637/0005-2086(2002)046[0102:VORIOM]2.0.CO;2
- Kleven, S.H., King, D.D. & Anderson, D.P. (1972). Airsacculitis in broilers from Mycoplasma synoviae: effect on air-sac lesions of vaccinating with infectious bronchitis and Newcastle virus. Avian Diseases, 16, 915–924. doi: 10.2307/1588772
- Kreizinger, Z., Sulyok, K.M., Pásztor, A., Erdélyi, K., Felde, O., Povazsán, J., Kőrösi, L. & Gyuranecz, M. (2015). Rapid, simple and cost-effective molecular method to differentiate the temperature sensitive (ts+) MS-H vaccine strain and wild-type Mycoplasma synoviae isolates. PLoS One, 10, e0133554. doi: 10.1371/journal.pone.0133554
- Landis, J.R. & Koch, G.G. (1977). The measurement of observer agreement for categorical data. Biometrics, 33, 159–174. doi: 10.2307/2529310
- Landman, W.J.M. (2014). Is Mycoplasma synoviae outrunning Mycoplasma gallisepticum? A viewpoint from the Netherlands. Avian Pathology, 43, 2–8. doi: 10.1080/03079457.2014.881049
- Landman, W.J.M. & Feberwee, A. (2001). Field studies on the association between amyloid arthropathy and Mycoplasma synoviae infection, and experimental reproduction of the condition in brown layers. Avian Pathology, 30, 629–639. doi: 10.1080/03079450120092125
- Markham, J.F., Morrow, C.J., Scott, P.C. & Whithear, K.G. (1998a). Safety of a temperature-sensitive clone of Mycoplasma synoviae as a live vaccine. Avian Diseases, 42, 677–681. doi: 10.2307/1592702
- Markham, J.F., Morrow, C.J. & Whithear, K.G. (1998b). Efficacy of a temperature-sensitive Mycoplasma synoviae live vaccine. Avian Diseases, 42, 671–676. doi: 10.2307/1592701
- Markham, J.F., Scott, P.C. & Whithear, K.G. (1998c). Field evaluation of the safety and efficacy of a temperature-sensitive Mycoplasma synoviae live vaccine. Avian Diseases, 42, 682–689. doi: 10.2307/1592703
- Morrow, C.J., Bell, I.G., Walker, S.B., Markham, P.F., Thorp, B.H. & Whithear, K.G. (1990). Isolation of Mycoplasma synoviae from infectious synovitis of chickens. Australian Veterinary Journal, 67, 121–124. doi: 10.1111/j.1751-0813.1990.tb07726.x
- Morrow, C.J., Markham, J.F. & Whithear, K.G. (1998). Production of temperature-sensitive clones of Mycoplasma synoviae for evaluation as live vaccines. Avian Diseases, 42, 667–670. doi: 10.2307/1592700
- Productschap voor Pluimvee en Eieren. (2012). Besluit onderzoeksprogramma Mycoplasma gallisepticum, M. synoviae en M. meleagridis in de pluimveesector.
- Productschap voor Pluimvee en Eieren. (2013). Besluit tot wijziging van het besluit onderzoeksprogramma M. gallisepticum, M. synoviae en M. meleagridis in de pluimveesector 2012.
- Raviv, Z. & Kleven, S.H. (2009). The development of diagnostic real-time TaqMan PCRs for the four pathogenic avian mycoplasmas. Avian Diseases, 53, 103–107. doi: 10.1637/8469-091508-Reg.1
- Shahid, M.A., Ghorashi, S.A., Agnew-Crumpton, R., Markham, P.F., Marenda, M.S. & Noormohammadi, A.H. (2013). Combination of differential growth at two different temperatures with a quantitative real-time polymerase chain reaction to determine temperature-sensitive phenotype of Mycoplasma synoviae. Avian Pathology, 42, 185–191. doi: 10.1080/03079457.2013.779363
- Shahid, M.A., Markham, P.F., Marenda, M.S., Agnew-Crumpton, R. & Noormohammadi, A.H. (2014). High-resolution melting-curve analysis of obg gene to differentiate the temperature-sensitive Mycoplasma synoviae vaccine strain MS-H from non-temperature-sensitive strains. PLoS One, 9, e92215. doi: 10.1371/journal.pone.0092215
- Shahid, M.A., Markham, P.F., Markham, J.F., Marenda, M.S. & Noormohammadi, A.H. (2013). Mutations in GTP binding protein Obg of Mycoplasma synoviae vaccine strain MS-H: implications in temperature-sensitivity phenotype. PLoS One, 8, e73954. doi: 10.1371/journal.pone.0073954
- Tamura, K., Stecher, G., Peterson, D., Filipski, A. & Kumar, S. (2013). MEGA6: molecular evolutionary genetics analysis version 6.0. Molecular Biology and Evolution, 30, 2725–2729. doi: 10.1093/molbev/mst197
- Tannock, G.A. & Shafren, D.R. (1994). Avian encephalomyelitis: a review. Avian Pathology, 23, 603–620. doi: 10.1080/03079459408419031
- Yoder, H.W., Jr., Drury, L.N. & Hopkins, S.R. (1977). Influence of environment on airsacculitis: effects of relative humidity and air temperature on broilers infected with Mycoplasma synoviae and infectious bronchitis. Avian Diseases, 21, 195–208. doi: 10.2307/1589340