ABSTRACT
Salmonellosis ranks among the major diseases of commercial poultry, and its presence in poultry flocks is responsible for economic losses and risks related to public health. Vaccines are an important tool within integrated programmes to control salmonellosis. The purpose of this study was to assess cross-protection provided by the Poulvac® ST vaccine in the control of Salmonella Heidelberg in experimentally challenged 3- and 21-day-old birds. Eighty birds were identified and separated into four treatments (T1: vaccinated and challenged at 3 days of age, T2: unvaccinated and challenged at 3 days of age, T3: vaccinated and challenged at 21 days of age, and T4: unvaccinated and challenged at 21 days of age). The inoculum was produced from a Brazilian field strain of SH. At the end of the experiment, caecum and liver/spleen samples were collected for quantitative and qualitative analysis of SH, respectively. Analysis of the liver/spleen showed that Poulvac® ST significantly (P ≤ 0.05) reduced the percentage of SH positivity in the group challenged at 3 days of age, while in the group challenged at 21 days this difference was almost considered significant (P = 0.1818). On the other hand, there was no statistically significant difference in SH count in the caecum (CFU/g) in the group challenged at 3 days, but for the group challenged at 21 days the SH counts were significantly (P ≤ 0.05) lower in the vaccinated group when compared to the positive control.
Introduction
Salmonellosis ranks among the most critical food-borne diseases affecting international trade in poultry, and is used as a criterion for product quality (OIE, Citation2015). Bacteria of this genus have been identified as a major cause of food poisoning in humans (BRASIL, Citation2003; Mead et al., Citation2010).
Salmonella Heidelberg (SH) is a significant source of non-typhoidal Salmonella infection and has been found in several Brazilian states where industrial poultry production is widespread, causing concerns about potential risks to public health (Dickel, Citation2004; Borsoi et al., Citation2006). According to the latest results of the Pathogen Reduction Plan and European Union Rapid Alert System for Food and Feed, SH is often present in positive samples taken from Brazilian poultry products (Freitas, Citation2011; RASFF, Citation2014).
Studies examining experimental inoculation of birds have demonstrated that the behaviour of SH resembles that of Salmonella Enteritidis, which can generate bacteria counts in both the intestine (caeca) and the viscera (liver); additionally, the curve to quantify the positivity of the two analysed serotypes was almost identical 72 h after inoculation (Borsoi et al., Citation2011). For all analysis times, SH counts in the intestines exceeded those in the viscera. These results demonstrate the challenge faced by the poultry sector in attempting to control SH, and the risks related to positive testing in poultry products, since this microorganism is present in considerable quantities in both the intestine and the viscera (Piao et al., Citation2007; Wollin, Citation2007; Gantois et al., Citation2008). The SH in Brazil has asymptomatic manifestation in positive birds and its presence offers a risk just for humans.
Poulvac® ST is a genetically modified live vaccine developed from a strain of Salmonella enterica subsp. enterica serovar Typhimurium (STM-1) (Alderton et al., Citation1991; Coloe et al., Citation1994). This serovar produces antigens in the configuration of lipopolysaccharide and flagella, which are the same as those seen in other group B salmonellas (such as SH). This similarity in the somatic and flagellar antigens indicates a possibility of good heterologous immune response. The vaccine aims to protect chickens to reduce transmission to humans.
The objective of this study was to assess the effectiveness of Poulvac® ST in controlling S. enterica subsp. enterica serovar Heidelberg in broiler chickens, in both the intestines and in the viscera.
Materials and methods
Poultry and treatments
Eighty one-day-old broiler chicks of both genders were separated into four treatments (T1: vaccinated and challenged at 3 days of age; T2: unvaccinated and challenged at 3 days of age; T3: vaccinated and challenged at 21 days of age; T4: unvaccinated and challenged at 21 days of age); these chicks from a Cobb Slow line breeder lineage, commonly used in Brazilian broiler companies, came from a flock certified to be free from Salmonella spp. When the chicks arrived from the supplier, the cardboard floor of the box was analysed to assess the presence or absence of Salmonella spp., using the standard methodology indicated by the Brazilian Ministry of Agriculture, Livestock, and Food Supply (Directive 126 of November 6, Citation1995) (BRASIL, Citation1995).
The birds in all groups were identified on the day they were assigned into groups, and were fed and watered using tubular feeders and bell-type watering stations (chick food and watering stations were used up to seven days of age). Throughout the experiment, birds were given free access to food and water.
This protocol was submitted to the Zoetis Ethics and Animal Welfare Committee for approval prior to the experiment, and was approved in process 07-15-70AQO.
Vaccination and placement
The study was done in the Experimental Laboratory of Mercolab, located in Cascavel, Paraná State, Brazil. Immediately after the birds arrived, they were weighed and vaccinated (). Vaccination was conducted via spraying with Poulvac® ST (batch 002/14 manufactured 08/14, expiration 02/16). A total volume of 40 ml was sprayed on the 40 chicks (T1 and T3) on the first day of life. The number of doses was one dose/bird with a minimum amount of 3 × 107 colony-forming units (CFU)/dose. The vaccine was diluted with a free chlorine inactivator containing non-toxic blue dye (batch 045/14 manufactured May/14, expiration May/16).
Table 1. Parameters assessed and age at collection in different treatments.
The vaccinated birds were placed together with their respective control birds in two pens (T1 + T2 and T3 + T4) and were marked with paint to allow identification. Just in the second dose of the vaccine, at 14 days of age, the birds were separated in the same room by a movable fence to revaccinate only the tagged birds. One day after vaccination, the fence was removed. The purpose of allowing the birds to co-mingle was to validate the independence assumption and the analytical methods used.
The birds remained in 2.6 m² pens on new wood shavings (floor pens) in closed rooms during the experimental period. Plant pelleted feed without any Salmonella inhibitory ingredients (e.g. organic acids, prebiotics, or probiotics) was provided. Care was taken to prevent cross contamination by wearing personal protective equipment: shoe covers, gowns, hair nets, and gloves. Both rooms used were identical and located side by side with independent entrance and exit. Personal protective equipment was changed when moving between rooms following the order from T3 + T4 room to T1 + T2 room. Both the wood shavings and the rooms were sampled for the presence of Salmonella spp. before the start of the experiment.
Vaccine
Poulvac® ST is a commercial vaccine produced by Zoetis (Madison, NJ, USA). It contains a live non-virulent strain of AWC 591 Salmonella Typhimurium in lyophilized form with a titre of ≥3 × 107 CFU/dose. This variant of S. Typhimurium was altered by the deletion of two genes, aroA and serC. The deletion of these genes results in an organism that retains the structure of the cell wall and flagellum, which keeps immunizing antigens intact.
Challenge
The challenge was performed with a SH strain isolated from the field. This sample was rendered resistant to antibiotics (nalidixic acid and novobiocin), which were incorporated into brilliant green agar to inhibit gut microbiota and facilitate counting. SH was grown in BHI broth for 18–24 h at 37°C until reaching a concentration of 1.0 × 109 CFU/ml; serial dilution was then performed until a concentration of 1.0 × 106 CFU/ml was achieved (Pickler et al., Citation2012). For birds in groups 1 and 2, 0.5 ml of this culture was inoculated orally at 3 days of age, and at 21 days of age for birds in groups 3 and 4 (). This dose of challenge was administered based on previous work done with Brazilian strain of SH (pilot test). The birds and environmental conditions were monitored daily.
Laboratory analyses
In order to count the Salmonella spp, at 28 days of age, the samples collected from the caecum and liver were macerated, weighed, and serially diluted in saline solution at a proportion of 1:10 until a dilution of 10−5 was achieved. Next, 0.1 ml of each dilution was seeded into brilliant green agar (which also contained 12.5 µg/ml of nalidixic acid and 20 µg/ml of novobiocin) with a Drigalski inoculation spatula and incubated at 36 ± 1°C for 18–24 h. After incubation, the dishes were read and colonies were counted. Those that were suspected to be Salmonella were selected for biochemical and serological antigenic confirmation.
To detect SH in the organ samples (liver and spleen) at 28 days of age, the conventional procedure for isolating Salmonella (pre-enrichment) was carried out according to Brazilian directive 126 (BRASIL, Citation1995).
To detect the Poulvac® ST vaccine strain, eight individual samples collected from cloacal swabs, in group 1 and 3, at days 2, 7, and 12, were subjected to real-time polymerase chain reaction analysis (RT-PCR) using a Rotor Gene SYBR Green PCR kit (Qiagen). Based on the sequence of the aroA gene region of the genome of the strain used in Poulvac® ST, a pair of oligonucleotides (primers) was designed that exclusively amplify a 280 bp fragment of the vaccine’s DNA and do not detect other field isolates of Salmonella spp. One of the oligonucleotides is based on the IS10 element that is only present in the vaccine strain.
The oligonucleotides used for detection of the vaccine were:
Oligonucleotide 1–5′ CGG CAT TAC CGA GAA ACA GT 3′
Oligonucleotide 2–5′ AATAA CTGCA GTGAT CATAT GACAA GATGT GT 3′
The model for each challenge day (T1 and T3) was a completely random design with bird as the experimental unit. So each challenge day was separately analysed as a completely random design with only two treatments. So there was only one possible contrast for each day. All hypotheses were conducted with significance of P ≤ 0.05 using a two-tailed test. For positive liver/spleen testing, Fisher’s exact test was used. The counts for SH colonies in the caecum (CFU/g) were analysed using a generalized linear model (negative binomial distribution). Statistical analysis of body weights was done by F test at P ≤ 0.05 using software SAS version 9.4, SAS Institute Inc., Cary, NC, USA.
Results
Samples taken from the environment, from the birds on the first day, and from the feed used in the experiment were all negative. The counts in the caecum on day 28 are shown in . The count values for these assessments are presented in CFU per gram of caecum. The count results <1.0 × 10² in caecum are expressed as zero in because of the detection limits of the technique used (count below 100/CFU/g). Samples with a negative culture result were assigned a value of 0 CFU/g in the statistical analysis. The average of quantitative counts and qualitative results in the caeca in different treatments are presented in . presents the results of the qPCR used to detect the vaccine strain in groups 1 and 3 in order to investigate shed bacteria. Faecal swabs taken at 2, 7, and 12 days after vaccination revealed that orally vaccinated chickens were excreting the vaccine only at the first two sampling times. presents the statistical assessments for isolation in spleen/liver and for body weight on day 28. SH isolation in spleen/liver is presented as positive/negative and not as bacterial burden because of difficulties in counting the low levels of contamination in viscera compared with caecum. The qualitative method was preferred to the quantitative method for spleen/liver analysis because many samples with low SH load would be considered negative as a function of the limit of the technique used (quantification only from 100 CFU/g). Normally for SH, the level of contamination in caecum is higher than in spleen/liver (Borsoi et al., Citation2011).
Figure 1. Average and distribution of SH counts in samples collected from broiler caeca at 28 days of age in the different groups (CFU/g caecum).
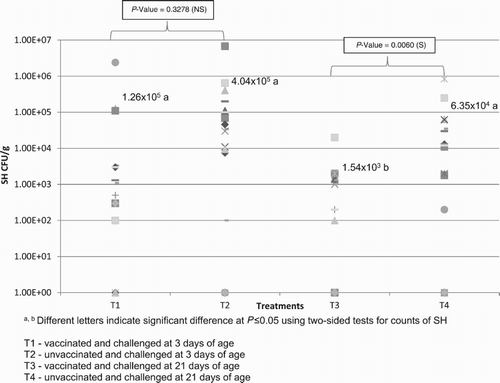
Table 2. Average of the quantitative counts (CFU) and prevalence (positive %) of SH in the caecum on day 28.
Table 3. Detection of Poulvac® ST vaccine strain using real-time PCR of cloacal swab samples collected from broilers at different times after vaccination.
Table 4. Average body weight and SH presence (%) (conventional isolation methodology) in broilers liver and spleen at 28 days of age (groups 1, 2, 3, and 4).
Discussion
Vaccination is the prominent means of controlling non-typhoidal Salmonella sorovars; both inactivated and live vaccines are widely adopted by the poultry industry according to regional challenges and governing legislation (Van Immerseel et al., Citation2005; de Freitas Neto et al., Citation2008; Majowicz et al., Citation2010; Berghaus et al., Citation2011). However, the limited ability of vaccines to act on the different serotypes that exist in the field is well known, especially in the case of inactivated vaccines, which have a more specific action (Penha Filho et al., Citation2009). Furthermore, live vaccines offer broader protection that in turn grants more comprehensive control, since cross-immunity may occur, depending on the similarities between the involved serovars (Hassan & Curtiss, Citation1994). The results presented in demonstrate the existence of a cross-immunity conferred by Poulvac® ST, which significantly reduced positive results in the liver/spleen of birds that were experimentally inoculated at 3 days of age with a Brazilian strain of SH when compared to the control group. Studies with birds and other species have demonstrated cross-immunity of live vaccines among different serovars and the highest levels of protection are found when the serovars involved have similarity between their immunizing antigens (Heithoff et al., Citation2001; Mohler et al., Citation2008). Cross-protection of live vaccines is an important benefit for poultry farmers, particularly in this case, where it eliminates the need for another SH-specific vaccine.
However, when we analysed the SH count in the caeca of birds challenged at 3 days of age (), we found that the existing numerical reduction was not statistically significant, when compared to the control. This result agrees with studies that demonstrated a direct correlation between earlier challenge and greater difficulty in controlling infection through vaccination (Poppe, Citation1999). As the vaccination occurred very shortly before challenge, it is possible that the protection noted was due also to a non-specific increase in innate immunity in response to the vaccination. In this case as the challenge was very early, competitive exclusion effect seems to have more importance (Van Immerseel et al., Citation2005). This short window is not sufficient for the birds to produce a complete adaptive immune response. There will be no specific immunity (such secretory IgA) at this time point. This reinforces the fact that birds must be immunized as soon as possible before challenge, during the first days of life, so that they have time to develop immunity. This is often difficult, since production environments are often contaminated and flocks can be challenged as soon as they are housed (personal communication of Manfio, Citation2012).
Moreover, the statistically insignificant results of the samples taken from the caeca of the birds challenged at 3 days () also corroborate that control of Salmonella infection within the intestine is harder to achieve than in the case of infection in the viscera, since the reach of the immune response depends on local mucosal immunity. Secretory IgA participates directly in local immunity, since this antibody is secreted into the mucosal surface of the intestine and helps reduce bacterial colonization in the intestinal lumen (Pasetti et al., Citation2011). In addition, cell-based immune responses where CD8+ T cells are activated as the result of live vaccines are more effective when compared to high levels of antibodies in the serum of birds that were inoculated with inactivated vaccines (Penha Filho et al., Citation2012). Experiments with inactivated vaccines have shown significant reduction of colonization by Salmonella in organs, but the intestinal tract may still be susceptible to colonization. Therefore, it is more difficult to prevent colonization in this organ than in others (Adriaensen et al., Citation2007).
The SH count in the vaccinated group, which was challenged at 21 days of age, was significantly less (P ≤ 0.05) than in the control group (), showing the vaccine’s ability to also reduce intestinal colonization, since two doses were given before the challenge. Both the innate immune system and the specific immunity conferred by vaccination with live vaccine help prevent intestinal colonization, and the later the challenge takes place, the greater the effectiveness of the vaccination programme (McSorley, Citation2014). Although the differences in prevalences between the vaccinated and control groups were not statistically significant, there was a reduction in the percentage of positive liver/spleen results in birds inoculated at 21 days of age, indicating a beneficial trend from the use of the vaccine to reduce visceral contamination (). Insufficient colonization of the unvaccinated group (only 25%) was a limitation of this study, which, if higher, could have brought the significance values below the 0.05 threshold.
The mutation of the aroA gene of the Poulvac® ST vaccine resulted in functional loss of phosphoenolpyruvyl-shikimate-5-phosphate synthase, an enzyme required for biosynthesis of aromatic amino acids. This auxotrophic mutation can be compensated in vitro by supplementation of phenylalanine, tryptophan, and tyrosine. However, since these amino acids are not available for the Salmonella vaccine in the host tissue, this results in attenuation during growth in vivo (Coloe et al., Citation1994). Consequently, the Poulvac® ST vaccine shows self-limiting behaviour where it remains viable in the bird for a restricted time, but long enough to trigger a cellular immune response. Analysis using specific qPCR in this experiment showed the vaccine’s ability to survive for a limited time in the intestines of the birds (). These data agree with research conducted on the original STM-1 strain of the vaccine (Alderton et al., Citation1991).
After a period of 12 days post-vaccination of first dose, the birds were not detected as bearers of the agent from the Poulvac® ST vaccine (). It was possible to detect the “vaccine uptake” up to the seventh day of life in the birds when the specific PCR was conducted on Poulvac® ST vaccine. This length of time would be sufficient to stimulate local and systemic immunity, preferably prior to the field challenge. In addition, studies show that during the time that live vaccines remain in the intestine, there is a potential mechanism of protection by bacteriological competitive exclusion (Van Immerseel et al., Citation2005). Future studies should investigate if live vaccines mimic field infection, invading the host organism and reaching internal organs in the same way the field strain does. For some time, great efforts have been made towards developing this type of vaccine with gene deletion because even though these are live salmonellas and therefore retain the field challenging behaviour during initial infection, they are also extremely safe as they do not persist in poultry or even in the environment for long periods of time (Hoiseth & Stocker, Citation1981; Barrow et al., Citation1990; Hormaeche et al., Citation1991; Cooper et al., Citation1994; Tan et al., Citation1997; Van Immerseel et al., Citation2002). Thus, the great advantage of genetically modified live vaccines is the low risk of reversion to virulence in comparison to vaccines made from attenuated rough strains, for example (Frey, Citation2007; Matsuda et al., Citation2010; Penha Filho et al., Citation2010; Matsuda et al., Citation2011a, b; Shehata et al., Citation2013; Van Immerseel et al., Citation2013).
In the assessed experimental conditions, there was no statistical difference (P > 0.05) for the variable weight in the different treatments, even in birds that were only challenged with SH (). These results are in agreement with recent studies indicating that various non-typhoidal Salmonella serovars are highly adapted to the host and do not cause a reduction in performance parameters (Setta et al., Citation2012; Muniz et al., Citation2015). This asymptomatic behaviour makes the control of these serovars more difficult because contaminated flocks generally are infected without demonstrating clinical signs that are visible to producers.
The results of this experimental study demonstrate that genetically modified live vaccines can be a useful and safe tool for controlling SH in poultry. Vaccination with Poulvac® ST was able to partially decrease the bacterial load of SH in both the caecum and liver/spleen after oral challenge. However, it is important to know their characteristics and limitations, in order to use them effectively. According to the results of this trial, the efficacy of cross-protection to SH depends on at least two applications of the vaccine. Future studies are recommended to indicate the duration of this immunity, as the test was only conducted 7 days following vaccination.
Acknowledgements
The authors wish to acknowledge the assistance provided by Dario K. Filho, Vânia M. C. A. Bernardes and Thiago P. Ribeiro during the monitoring of vaccination procedures and handling the birds at the Mercolab Laboratory. Special thanks to John Dickson for running the statistical analysis.
Disclosure statement
No potential conflict of interest was reported by the authors.
References
- Adriaensen, C., De Greve, H., Tian, J.Q., De Craeye, S., Gubbels, E., Eeckhaut, V., Van Immerseel, F., Ducatelle, R., Kumar, M. & Hernalsteens, J.P. (2007). A live Salmonella enterica serovar Enteritidis vaccine allows serological differentiation between vaccinated and infected animals. Infection and Immunity, 75, 2461–2468. doi: 10.1128/IAI.01357-06
- Alderton, M.R., Fahey, K.J. & Coloe, P.J. (1991). Humoral responses and salmonellosis protection in chickens given a vitamin-dependent Salmonella typhimurium mutant. Avian Diseases, 35, 435–442. doi: 10.2307/1591205
- Barrow, P.A., Hassan, J.O. & Lovell, M.A., Berchieri, A. (1990). Vaccination of chickens with aroA and other mutants of Salmonella typhimurium and S. enteritidis. Research in Microbiology, 141, 851–853. doi: 10.1016/0923-2508(90)90120-F
- Berghaus, R.D., Thayer, S.G., Maurer, J.J. & Hofacre, C.L. (2011). Effect of vaccinating breeder chickens with a killed Salmonella vaccine on Salmonella prevalences and loads in breeder and broiler chicken flocks. Journal of Food Protection, 74, 727–734. doi: 10.4315/0362-028X.JFP-10-542
- Borsoi, A., Moraes, H.S., Salle, C.T.P., Bettiol, G. & Nascimento, V.P. (2006). Sorovares de Salmonella isolados de carcaça de frango resfriadas e swabs de arrasto. Revista Brasileira de Ciência Avícola, 8, 229–229.
- Borsoi, A., Santos, L.R., Rodrigues, L.B., Moraes, H.L.S., Salle, C.T.P. & Nascimento, V.P. (2011). Behavior of Salmonella Heidelberg and Salmonella Enteritidis strains following broiler chick inoculation: evaluation of cecal morphometry, liver and cecum bacterial counts and fecal excretion patterns. Brazilian Journal of Microbiology, 42, 266–273. doi: 10.1590/S1517-83822011000100034
- BRASIL (1995). Ministério da Agricultura e Abastecimento. Portaria no 126 publicada em 06 de novembro de 1995. Aprova as Normas de credenciamento e Monitoramento de Laboratórios de Diagnóstico das Salmoneloses Aviárias (S. Enteritidis, S. Gallinarum, S. Pullorum e S. Typhimurium e os métodos analíticos Oficiais para Análises Microbiológicas para Controle de Produtos de Origem Animal e Água. Ministério da Agricultura Pecuária e Abastecimento, Brasília.
- BRASIL (2003). Ministério da Agricultura, Pecuária e Abastecimento. Instrução Normativa 78 publicada em 05 de novembro de 2003. Programa Nacional de Sanidade Avícola. Diário Oficial da União, República Federativa do Brasil, Brasília – DF.
- Coloe, P.J., Gerraty, N.L., Christopher, W., Alderton, M.R. & Smith, S.C. (1994). Vaccination of poultry with Salmonella typhimurium STM-1. Laboratory and field evaluations. Proceedings of the 43rd Western Poultry Disease Conference (pp. 37–39). Sacramento, CA, USA.
- Cooper, G.L., Venables, L.M., Woodward, M.J. & Hormaeche, C.E. (1994). Invasiveness and persistence of Salmonella enteritidis, Salmonella typhimurium, and a genetically defined S. enteritidis aroA strain in young chickens. Infection and Immunity, 62, 4739–4746.
- Dickel, E.L. (2004). Usage of conventional microbiological technique, polymerase chain reaction (PCR) and enzyme-linked immunoenzymatic assay (ELISA) in Salmonella monitoring in poultry carcasses for hygienic health control of the slaughter process. [Doctoral Thesis] Porto Alegre (RS): Universidade Federal do Rio Grande do Sul.
- Freitas, J. (2011). Evolução de sorovares Modelo de Banco de Cepas. In: International Seminar on Avian Salmonellosis, Rio de Janeiro.
- de Freitas Neto, O.C., Mesquita, A.L., de Paiva, J.B., Zotesso, F. & Berchieri Júnior, A. (2008). Control of Salmonella enterica serovar Enteritidis in laying hens by inactivated Salmonella Enteritidis vaccines. Brazilian Journal of Microbiology, 39, 390–396. doi: 10.1590/S1517-83822008000200034
- Frey, J. (2007). Biological safety concepts of genetically modified live bacterial vaccines. Vaccine, 25, 5598–5605. doi: 10.1016/j.vaccine.2006.11.058
- Gantois, I., Eeckhaut, V., Pasmans, F., Haesebrouck, F., Ducatelle, R. & Van Immerseel, F. (2008). A comparative study on the pathogenesis of egg contamination by different serotypes of Salmonella. Avian Pathology, 37, 399–406. doi: 10.1080/03079450802216611
- Hassan, J.O. & Curtiss III, R. (1994). Development and evaluation of an experimental vaccination program using a live avirulent Salmonella typhimurium strain to protect immunized chickens against challenge with homologous and heterologous Salmonella serotypes. Infection and Immunity, 62, 5519–5527.
- Heithoff, D.M., Enioutina, E.Y., Daynes, R.A., Sinsheimer, R.L., Low, D.A. & Mahan, M.J. (2001). Salmonella DNA adenine methylase mutants confer cross-protective immunity. Infection and Immunity, 69:6725–6730. doi: 10.1128/IAI.69.11.6725-6730.2001
- Hoiseth, S.K. & Stocker, B.A.D. (1981). Aromatic-dependent Salmonella typhimurium are non-virulent and effective as live vaccines. Nature, 291, 238–239. doi: 10.1038/291238a0
- Hormaeche, C.E., Joysey, H.S., Desilva, L., Izhar, M. & Stocker, B.A. (1991). Immunity conferred by Aro-Salmonella live vaccines. Microbial Pathogenesis, 10, 149–158. doi: 10.1016/0882-4010(91)90075-L
- Majowicz, S.E., Musto, J., Scallan, E., Angulo, F.J., Kirk, M., ÓBrien, S.J., Jones, T.F., Fazil, A. & Hoekstra, R.M. (2010). The global burden of nontyphoidal Salmonella gastroenteritis. Clinical Infectious Diseases, 50, 882–889. doi: 10.1086/650733
- Manfio, L. (2012). Doux FrangoSul, Montenegro – RS. Personal communication.
- Matsuda, K., Chaudhari, A.A., Kim, S.W., Lee, K.M. & Lee, J.H. (2010). Physiology, pathogenicity and immunogenicity of lon and/or cpxR deleted mutants of Salmonella Gallinarum as vaccine candidates for fowl typhoid. Veterinary Research, 41, 59. doi: 10.1051/vetres/2010031
- Matsuda, K., Chaudhari, A.A. & Lee, J.H. (2011a). Evaluation of safety and protection efficacy on cpxR and lon deleted mutant of Salmonella Gallinarum as a live vaccine candidate for fowl typhoid. Vaccine, 29, 668–674. doi: 10.1016/j.vaccine.2010.11.039
- Matsuda, K., Chaudhari, A.A. & Lee, J.H. (2011b). Comparison of the safety and efficacy of a new live Salmonella Gallinarum vaccine candidate JOL916, with the SG9R vaccine in chickens. Avian Diseases, 55, 407–412. doi: 10.1637/9680-020611-Reg.1
- McSorley, SJ. (2014). Immunity to intestinal pathogens: lessons learned from Salmonella. Immunological Reviews, 260, 168–182. doi: 10.1111/imr.12184
- Mead, G., Lammerding, A.M., Cox, N., Doyle, M.P., Humbert, F., Kulikovskiy, A., Panin, A., do Nascimento, V.P. & Wierup, M. (2010). Scientific and technical factors affecting the setting of Salmonella criteria for raw poultry: a global perspective. Journal of Food Protection, 73, 1566–1590. doi: 10.4315/0362-028X-73.8.1566
- Mohler, V.L., Heithoff, D.M., Mahan, M.J., Walker, K.H., Hornitzky, M.A., Shuma, L.H.C, Makin, K.J. & House, J.K. (2008). Cross-protective immunity conferred by a DNA adenine methylase deficient Salmonella enterica serovar Typhimurium vaccine in calves challenged with Salmonella serovar Newport. Vaccine, 26, 1751–1758. doi: 10.1016/j.vaccine.2008.01.018
- Muniz, E.C., Pickler, L., Lourenço, M.C., Kraieski, A.L., Mesa, D. & Santin, E. (2015). Avaliação da resposta imunológica da mucosa intestinal de frangos de corte desafiados com diferentes sorovares de salmonella. Pesquisa Veterinária Brasileira, 35, 241–248. doi: 10.1590/S0100-736X2015000300006
- OIE, (2015). World Animal Health Information System.
- Pasetti, M.F., Simon, J.K., Sztein, M.B. & Levine, M.M. (2011). Immunology of gut mucosal vaccines. Immunological Reviews, 239, 125–148. doi: 10.1111/j.1600-065X.2010.00970.x
- Penha Filho, R.A.C., de Paiva J.B., Argüello, Y.M., da Silva, M.D., Gardin, Y., Resende, F., Berchieri Junior, A.B. & Sesti, L. (2009). Efficacy of several vaccination programmes in commercial layer and broiler breeder hens against experimental challenge with Salmonella enterica serovar Enteritidis. Avian Pathology, 38, 367–375. doi: 10.1080/03079450903183645
- Penha Filho, R.A. C., de Paiva, J.B., da Silva, M.D., de Almeida, A.M. & Berchieri Junior, A. (2010). Control of Salmonella enteritidis and Salmonella Gallinarum in birds by using live vaccine candidate containing attenuated Salmonella Gallinarum mutant strain. Vaccine, 28, 2853–2859. doi: 10.1016/j.vaccine.2010.01.058
- Penha Filho, R.A., Moura, B.S., de Almeida, A.M., Montassier, H.J., Barrow, P.A., Berchieri JR, A (2012). Humoral and cellular immune response generated by different vaccine programs before and after Salmonella Enteritidis challenge in chickens. Vaccine, 30, 7637–7643.
- Piao, Z., Toyota-Hanatani, Y., Ohta, H., Sasai, K., Tani, H. & Baba, E. (2007). Effects of Salmonella enterica subsp. enterica serovar Enteritidis vaccination in layer hens subjected to S. Enteritidis challenge and various feed withdrawal regimens. Veterinary Microbiology, 125, 111–119. doi: 10.1016/j.vetmic.2007.05.008
- Pickler, L., Hayashi, R.M., Lourenço, M.C., Miglino, L.B., Caron, L.F., Beirão, B.C.B., Silva, A.V.F. & Santin, E. (2012). Avaliação microbiológica, histológica e imunológica de frangos de corte desafiados com Salmonella Enteritidis e Minnesota e tratados com ácidos orgânicos. Pesquisa Veterinária Brasileira, 32, 27–36. doi: 10.1590/S0100-736X2012000100006
- Poppe, C. (1999). Epidemiology of Salmonella enterica serovar Enteritidis. In: A.M. Saeed, R.K. Gast, M.E. Potter (Eds.). Salmonella enterica serovar Enteritidis in humans and animals (pp. 3–18). Ames: Iowa State University Press.
- RASFF. (2014). The rapid alert system for food and feed. Annual Report of European Commission. 56p.
- Setta, A.M., Barrow, P.A., Kaiser, P. & Jones, M.A. (2012). Early immune dynamics following infection with Salmonella enterica serovars Enteritidis, Infantis, Pullorum and Gallinarum: cytokine and chemokine gene expression profile and cellular changes of chicken cecal tonsils. Comparative Immunology, Microbiology and Infectious Diseases, 35, 397–410. doi: 10.1016/j.cimid.2012.03.004
- Shehata, A.A., Sultan, H., Hafez, H.M. & Kruger, M. (2013). Safety and efficacy of a metabolic drift live attenuated Salmonella Gallinarum vaccine against fowl typhoid. Avian Diseases, 57, 29–35. doi: 10.1637/10287-062112-Reg.1
- Tan, S., Gyles, C.L. & Wilkie, B.N. (1997). Evaluation of an aro-A mutant Salmonella typhimurium vaccine in chickens using modified semisolid Rappaport Vassiliadis medium to monitor faecal shedding. Veterinary Microbiology, 54, 247–254. doi: 10.1016/S0378-1135(96)01279-5
- Van Immerseel F., De Buck, J., De Smet, I., Mast, J., Haesebrouck, F. & Ducatelle, R. (2002). The effect of vaccination with a Salmonella Enteritidis aroA mutant on early cellular responses in caecal lamina propria of newly-hatched chickens. Vaccine, 20, 3034–3041. doi: 10.1016/S0264-410X(02)00227-X
- Van Immerseel, F., Mehner, U., Rychlik, I., Nagy, B., Veige, P., Martin, G. Foster, N., Ducatelle, R. & Barrow, P. (2005). Vaccination and early protection against non-host-specific Salmonella serotypes in poultry: exploitation of innate immunity and microbial activity. Epidemiology and Infection, 133, 959–978. doi: 10.1017/S0950268805004711
- Van Immerseel, F., Studholme, D.J., Eeckhaut, V., Heyndrickx, M., Dewulf, J., Dewaele, I., Van Hoorebeke, S., Haesebrouck, F., Van Meirhaeghe, H., Ducatelle, R., Pazkiewicz, K. & Titball, R.W. (2013). Salmonella Gallinarum field isolates from laying hens are related to the vaccine strain SG9R. Vaccine, 31, 4940–4945. doi: 10.1016/j.vaccine.2013.08.033
- Wollin, R. (2007). A study of invasiveness of different Salmonella serovars based on analysis of the Enter-netdatabase. European Surveillance, 12, 5–8.