ABSTRACT
An investigation into the aetiology and pathogenesis of adenoviral gizzard erosion has been conducted following three natural outbreaks affecting one flock of 6-week-old replacement pullets and two consecutive placements of free range layers at the age of 21 and 23 weeks. Affected flocks showed increased mortality (0.12–0.30% per week), and gizzard lesions were consistent with fowl aviadenovirus (FAdV) involvement. To substantiate the initial findings, a selection of archived formalin-fixed paraffin-embedded gizzard samples from another 12 pullet and layer flocks, for which macroscopic and histopathological diagnosis of the disease were recorded in Great Britain during the period 2009–2016, were also investigated. In situ hybridization (ISH), virology and/or PCR confirmed the presence of FAdV species-A, serotype-1 (FAdV-A, FAdV-1) DNA in gizzard samples of all 15 cases investigated. Co-infections with additional FAdV serotypes including FAdV-8a were detected by serology and/or virology in two of the pullet flocks. However, species-specific in situ hybridization revealed that pathological changes of affected gizzards were only associated with the detection of FAdV-A. A subsequent in vivo study infecting 21-day-old SPF pullets with FAdV-1 or FAdV-8a strains isolated from the 6-week-old replacement pullets revealed characteristic pathomorphological changes only in the gizzards from birds infected with FAdV-1. While infection with FAdV-8a was confirmed by virology and serology, infected SPF birds did not develop pathomorphological changes. Therefore, the aetiological involvement of the isolated FAdV-8a in the development of adenoviral gizzard erosion in commercial pullets has been ruled out.
Introduction
Adenoviral gizzard erosion (AGE) was initially documented by Tanimura et al. (Citation1993) in 10-week-old pullets. Subsequently, diagnoses of this condition associated predominantly with infection of fowl aviadenovirus species-A, serotype-1 (FAdV-A, FAdV-1) have been almost exclusively reported in broilers from Europe and Japan (Hess, Citation2013). There have been significant economic losses reported in meat-producing chickens due to growth retardation, increased mortality rates and/or slaughter house condemnations of affected gizzards (Ono et al., Citation2001, Citation2003; Domanska-Blicharz et al., Citation2011; Grafl et al., Citation2012; Schade et al., Citation2013).
Currently, only limited and fragmented data about the occurrence of AGE in pullet and layer flocks are available. A retrospective study by Manarolla et al. (Citation2009) described histological lesions in archived gizzards from one Italian layer flock with no information on the clinical signs or the nature of the adenovirus involved. In Korea, Japan and Poland, FAdV-1 has been isolated from layers between 22 and 30 weeks of age showing apathy, anorexia and increased mortality, and associated with gross lesions in the gizzard and/or the upper intestinal tract (Lim et al., Citation2012; Domanska-Blicharz et al., Citation2015; Totsuka et al., Citation2015). Most recently, the occurrence of AGE and the condition’s influence on production parameters were described in 38-week-old laying hens (Matczuk et al., Citation2017).
In general, AGE is attributed to infection with “pathogenic” FAdV-1. However, in broilers there have also been reports of AGE associated with isolation of other FAdV serotypes (Okuda et al., Citation2004). The presence of FAdVs of different serotypes and/or species has been described several times in layers (Hess, Citation2013). However, no such report has investigated the aetiological implications in the context of AGE.
The present study describes clinical signs, pathomorphological changes and the results of virological investigations documented in a selection of 15 cases of AGE diagnosed between 2009 and 2016 in Great Britain based replacement pullets and layers housed in both free range and enriched cage systems. Furthermore, the aetiological role of different FAdV serotypes (specifically –1 and −8a) in the development of AGE was investigated by means of an in vivo study in SPF pullets.
Materials and methods
Case histories, post-mortem examinations and sampling
Fifteen cases of AGE identified in replacement pullets and layers () housed in either free range or enriched cage systems were investigated. Samples originated from three natural outbreaks of AGE occurring in one pullet flock (P-A) and two consecutive flocks of laying chickens from the same farm (L-A and L-B). Post-mortem examination in these three cases revealed pale carcasses and internal organs, dilated gizzards and proventriculi often filled with dark brown contents, and occasionally perforation of the gizzard. Gizzard lesions were characterized by different sized areas of severe roughening and discolouration of the koilin layer () as well as inflammation and ulceration of the mucosa. Gizzard samples (frozen (−20°C) and formalin-fixed paraffin-embedded (FFPE)) from these three natural outbreaks together with archived FFPE gizzards from 12 referral cases for which macroscopic and histopathological changes associated with AGE had been detected by the APHA Laboratory, Lasswade, UK, during the period 2009–2016 were retrospectively investigated by employing sophisticated molecular biological techniques. For the three more recent natural outbreaks, production data and additional samples were made available (P-A, L-A and L-B).
Figure 1. Gross lesions in gizzards from 41-day-old pullets (pullet flock P-A). Erosions in the koilin layer (arrows).
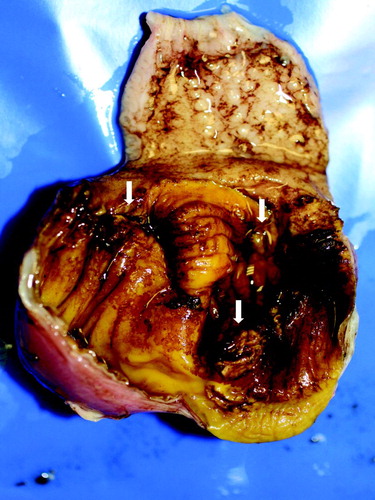
Table 1. Data on the pullet and layer flocks investigated together with results from histopathological and virological investigations of gizzards.
The pullet flock P-A consisted of 25,000 Lohmann Brown hens and increased mortality was documented over a period of 2 weeks starting at 41 days of age ((a)). Cumulative mortality amounted to 0.27% of the flock. The outbreak of AGE had no apparent effect on body weight and birds were on target at 15 weeks of age, when they were transferred to the layer farm. In addition to gizzard samples, serum samples from the affected pullet flock (P-A) were collected during the week mortality increased, i.e. at the same time that post-mortem examinations were performed (n = 30 samples from random birds per flock and sampling date). Further serum samples were taken approximately 6 weeks later. Serum samples from a second flock (P-B) on the farm without signs of gizzard erosions, which originated from the same parent flocks were collected at the same sampling points as the affected flock to use as reference values. No gizzard lesions were observed in pullet flock P-B at any time until transfer to the laying farm at 15 weeks of age. No organ samples were taken for investigations from flock P-B.
Figure 2. Weekly mortality (%) in (a) the affected pullet flock (P-A) and (b) the affected layer flocks (L-A and L-B - black and grey bars, respectively).
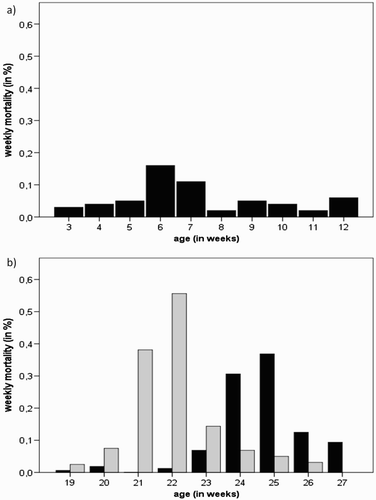
Two consecutive flocks (L-A and L-B) of 16,000 free range layers each were affected by AGE on the same farm a year apart from each other. Mortality in layer flock L-A started to increase during week 23. Losses remained slightly higher than expected for approximately 3 weeks until decreasing again to an acceptable level ((b)). During these 3 weeks cumulative mortality was 0.77%. Egg production dropped slightly (1–2%) from week 23 to 25 and the flock failed to peak as expected from the breed standards. The following flock L-B, placed on the same farm after depletion of flock L-A, also experienced an episode of increased mortality over a period of 3 weeks starting during week 21 ((b)). Mortality increased suddenly from 0 to 5 in one day. For a period of just over 2 weeks, daily mortality varied between three and 19 birds per day, with a total of 168 birds lost in 17 days; equal to 1.07% of the flock. Egg production remained on target during the period of increased mortality and the flock attained peak egg production at the expected value of 93% at 26 weeks of age. No significant changes were noted in any other production parameters. Serum samples (n = 12 samples from random birds) from layer flock L-B were made available for serological investigations at the time post-mortem examinations revealed gizzard erosions and approximately 3 weeks later.
Histological investigation and in situ hybridization
Tissue sections (3 µm) were prepared from FFPE gizzard samples of the 15 pullet and layer cases using a Microme HM360 rotary microtome (Microme Laborgeräte GmbH, Walldorf, Germany) and mounted on glass slides. Routine haematoxylin and eosin (H&E) staining was performed. In situ hybridization (ISH) for the detection of FAdV-A DNA in the gizzard sections was carried out according to a protocol previously described (Liebhart et al., Citation2006), using a specific DNA probe (5′-CGGGGTCGCAGCAGCTGCAGCTCGCGAGCGGAGAACTCG-3′) based on the FAdV-A long fibre gene. To investigate the presence of FAdV-E in the gizzard samples a specific DNA probe (5′-CGGTTCCGGTGGAAACCCGTCCCTCAACCCGCCGTT-3′) based on a conserved nucleotide sequence within the FAdV-E fibre gene (Schachner et al., Citation2016) was developed. The probes were synthesized and labelled at the 3′ end with digoxigenin by Eurofins Genomics GmbH (Ebersberg, Germany).
Sections prepared from cell culture pellets (preparation described in section “Cell culture and virus isolation”) as well as organ samples (gizzard, liver, pancreas and/or spleen) obtained from various experimental trials using control and FAdV-A, FAdV-D or FAdV-E infected SPF chickens with typical lesions of IBH or AGE (Grafl et al., Citation2013; Matos et al., Citation2016), were used as respective negative and positive controls for both probes.
Cell culture and virus isolation
Chicken embryo liver (CEL) cells were prepared from 14-day-old SPF chicken embryos (VALO Biomedia GmbH, Osterholz-Scharmbeck, Germany) according to a protocol of Schat and Sellers (Citation2008) to use for virus isolation. Frozen gizzard samples from pullet and layer cases (P-A, L-A and L-B) were homogenized in antibiotics (1 mg/ml streptomycin and 100,000 IU/ml penicillin)–phosphate buffered saline solution. Tissue homogenates (20%) were submitted to three freeze–thaw cycles, filter sterilized (0.2 µm syringe filter; VWR, Vienna, Austria) and used to inoculate nearly confluent CEL cells. Cell cultures were incubated for 5 days at 37°C in 5% CO2 atmosphere during which cells were examined for cytopathic effect (CPE). A sample was considered negative when no CPE was observed after three blind passages.
For use in the in vivo study, FAdV strains (−1 and −8a) isolated from affected gizzards were plaque purified three times and titres were determined according to the method of end point titration of Reed and Muench (Citation1938). A reverse-transcription-PCR was performed to rule out contamination by avian reovirus (Kant et al., Citation2003).
Preparation of cell culture pellets for ISH controls: confluent CEL cultures were infected with 106–8 median tissue culture infective doses (TCID50) of FAdV-A (strain CELO) or FAdV-E (strain YR36) in T-75 tissue culture flasks (Sarstedt, Nümbrecht, Germany). The cultures were incubated until CPE was noticed. At this point, cells were detached with a cell scraper and centrifuged for 10 min at 350 × g. The respective cell pellets were placed in biopsy embedding cassettes in 3.5% neutral buffered formalin and then embedded in paraffin. Sections of the pellets (3 µm) were used as positive controls for the respective ISH probes.
DNA extraction, PCR and sequence analyses
Two hundred microlitre of clarified supernatant from cell cultures showing CPE were used for DNA extraction with the DNeasy Blood & Tissue Kit (Qiagen, Vienna, Austria). DNA was also extracted from FFPE gizzards of all pullet and layer cases by the same method, according to the manufacturer’s instructions. DNA was stored at −20°C until use. Depending on the material of origin, extracted DNA was subjected to either a conventional PCR method (cell culture supernatant; Meulemans et al., Citation2001; Marek et al., Citation2010) or a real-time PCR (FFPE tissue; Günes et al., Citation2012), followed by sequence analysis of the amplification products obtained to facilitate typing of isolates.
Sequencing service was provided by LGC Genomics GmbH (Berlin, Germany). Assembly and nucleotide sequence alignments were conducted with Accelrys Gene, version 2.5 (Accelrys Inc., San Diego, CA, USA) and DNASTAR Lasergene 12.1 (DNASTAR Inc., Madison, WI, USA). Phylogenetic analyses were performed by comparing the obtained nucleotide sequences with available sequences from GenBank database.
Virus neutralization test
Serum samples from flocks P-A, P-B and L-B were heat inactivated for 30 min at 56°C before performing virus neutralization tests (VNT) according to a constant virus diluted serum method using 100 µl of 100 TCID50/100 µl of the FAdV-1 reference strain CELO. The VNTs were performed in 96-well plates containing CEL cells (preparation described in section “Cell culture and virus isolation”). After incubation for 5 days at 37°C in 5% CO2 atmosphere, the cells were investigated for CPE and titres were calculated. Antibody titres above 3 log2 were considered positive. Sera from pullet flocks P-A and P-B were also investigated for neutralizing antibodies against FAdV-C (FAdV-4, strain KR5) and FAdV-E (FAdV-8a, strain TR59).
Statistical analysis
Statistical differences between antibody titres against FAdV-1, FAdV-4 and/or FAdV-8a from pullet flocks with (P-A) and without gizzard erosions (P-B) and from the layer flock (L-B) at different sampling time points were analysed by the student t-test with the statistical software package SPSS Version 22 (IBM SPSS Statistics; IBM Corporation, Somer, NY, USA). Statistical differences with P < 0.05 were considered to be significant.
Pathogenicity of FAdV-1 and FAdV-8a for 21-day-old SPF pullets
FAdV-1 and FAdV-8a strains isolated from pullet flock P-A were used in the in vivo study. Embryonated SPF layer eggs (VALO) were incubated and hatched at our facility. After hatch, the chicks were individually marked (Swiftack, Heartland Animal Health Inc., Fair Play, MO, USA) and divided in three groups: one uninfected control group with six birds and two groups, of 12 chicks each, which were orally infected with 107 TCID50/chick of the respective virus at the 21st day of age. All groups were maintained separately in isolator units on wire floor with high efficiency particulate air (HEPA) filters under negative pressure (Montair Andersen bv, HM 1500, Sevenum, the Netherlands) from hatch and throughout the duration of the in vivo study. Feed and water were available ad libitum and birds were monitored daily for clinical signs. Cloacal swabs and serum samples were collected from two birds in the control group and four birds in both groups containing infected birds, at the 21st day of age and then at 7, 10 and 14 days post infection (DPI). Two control birds and four birds from each infected group were killed by intravenous administration of Thiopental Sodium followed by subsequent exsanguination after cutting the right jugular vein at 7, 10 and 14 DPI, and routine post-mortem examinations were performed. During post-mortem examinations, gross pathological changes in the koilin layer and the gizzard mucosa were assessed separately by means of a scoring system, with scores ranging from 0 (normal) to 3 (severe lesions) as described previously (Grafl et al., Citation2013). Samples of gizzard and liver were collected for histology (H&E and ISH) and virology. Viral DNA was isolated from cloacal swabs and organ samples using the DNeasy Blood and Tissue Kit (Qiagen), followed by real-time PCR investigations (Günes et al., Citation2012). Serum samples obtained during the in vivo study were investigated for neutralizing antibodies against FAdV-1 and FAdV-8a (method described in section “Virus neutralization test”).
The animal trial was discussed and approved by the institutional ethics committee and the national authority according to §26 of the Law for Animal Experiments, Tierversuchsgesetz (TVG) 2012, license number: bmwfw-68.205/0141-WF/V/3b/2016.
Results
Histopathology and ISH from pullet and layer cases
Results of the histological investigations and ISH from pullets and layers are summarized in . Histologically, gizzards showed non-suppurative inflammation with loss and degeneration of glandular epithelial cells and the occasional presence of basophilic intranuclear inclusions ((a)). Some of those cells were sloughed off from the mucosa and were found as part of a defective koilin layer. ISH revealed intranuclear inclusion bodies positive for FAdV-A DNA ((b)). However, no signal was obtained using the ISH probe for specific detection of FAdV-E DNA.
Figure 3. Histopathology of gizzards from 35-day-old pullets (pullet flock P3). (a) Erosion of the epithelium and infiltration of inflammatory cells in the mucosal membrane. Basophilic intranuclear inclusion bodies (arrows) can be observed in gizzard epithelial cells. H&E. Bar = 100 µm. (b) Inclusion bodies with FAdV-A DNA. ISH. Bar = 20 µm.
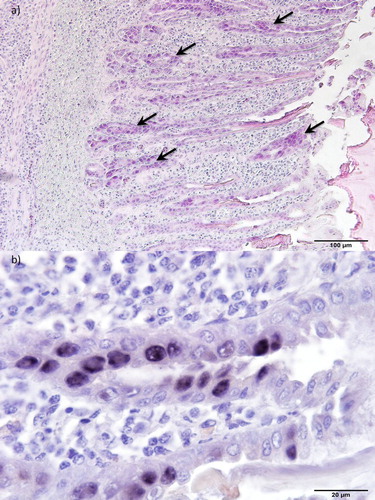
FAdV-A infected cell culture pellets and gizzard samples from a previous AGE trial (Grafl et al., Citation2013) used as ISH positive controls also showed a clear and strong signal by the FAdV-A probe, while the FAdV-E probe gave no signal. Similarly, the FAdV-E positive controls, consisting of an FAdV-E infected cell culture pellet and organ samples (liver, pancreas) collected from FAdV-E infected SPF chickens (Matos et al., Citation2016), showed strong signals within infected cells using the FAdV-E probe, while no signal was detected with the FAdV-A probe.
Virus isolation, PCR and sequencing from pullet and layer cases
Overviews of the virological, PCR and sequencing results from pullet and layer cases are given in . Virus isolation from frozen gizzard samples (P-A, L-A and L-B) revealed a characteristic CPE. Sequence analysis of the corresponding hexon loop-1 fragments consistently revealed highest nucleotide homologies (99.6%) with FAdV-A, FAdV-1 reference strain CELO. Amplification of FAdV-1 species-specific Fib-1 and Fib-2 genes was also successful from these samples. All FAdV-1 isolates in this study shared 100% nucleic acid identity in the hexon and fibre gene regions. When compared with non-pathogenic FAdV-1 reference strains OTE and CELO, the field isolates were more closely related to CELO within the hexon L1 gene region (99.6% vs. 99.0% nucleotide identity) while their fibre genes exhibited higher similarity to OTE (99.8% vs. 99.1% nucleotide identity in Fib-1, 99.7% vs. 99.1% nucleotide identity in Fib-2). Notably, the highest number of non-synonymous nucleotide substitutions was observed in the short fibre of field strains and CELO, all mapping to the earlier predicted region of the fibre head. Furthermore, the analysed portions of the long and short fibre gene, respectively, shared 99.9–100% to 99.8–100% nucleotide homology with other sequences of “pathogenic” European AGE field strains available from GenBank (FN557184, FN557185, GU952108 and GU952110), with deduced protein sequences being 100% identical. In addition to FAdV-1, gizzards from the pullet case (P-A) revealed the presence of other FAdVs; highest nucleic acid homologies were found in one sample with FAdV-C, FAdV-4 (99.6%) and in another with FAdV-E, FAdV-8a (98.6%).
Using real-time PCR, the presence of FAdV DNA was confirmed in FFPE gizzard samples of 12 out of 15 cases investigated. Based on 52 K gene analysis, highest sequence homologies were obtained with FAdV-A in all cases, with samples sharing 100% identity with each other. In addition, FAdV-E was detected in two of the pullet cases.
Virus neutralization test from pullet and layer cases
Results from VNT using sera collected during the natural outbreaks of AGE from pullet flocks P-A and P-B as well as from layer flock P-B including statistical differences are illustrated in . At 41 days of age, all serum samples collected from pullet flock P-A had neutralizing antibodies against FAdV-1 with an average titre of 6.33 ± 1.42 log2 (mean ± standard deviation (std)), whereas all samples from the “control” flock P-B without signs of gizzard erosion were negative. Approximately 6 weeks later, neutralizing antibodies were found in both flocks, P-A and P-B, with an average titre of 6.07 ± 1.53 log2 (mean ± std) and 4.83 ± 2.20 log2 (mean ± std), respectively. Neutralizing antibodies against FAdV-4 (strain KR5) and FAdV-8a (strain TR59) were observed in both pullet flocks only sporadically with low titres at the first sampling date. Overall, arithmetic means of the respective titres were negative. At the later sampling point, more birds had antibodies against FAdV-4 with relatively low titres, and neutralizing antibodies with high titres against FAdV-8a were detected in all samples investigated from both pullet flocks.
Figure 4. Box plot presentation of VNT results from pullet and layer cases. Titres (log2) of FAdV-1-, FAdV-4- and/or FAdV-8a-specific antibodies from pullet flocks with (P-A) and without gizzard erosions (P-B, “control”) and from a layer flock (L-B) collected at the time disease was diagnosed (P-A1, P-B1 & L-B1) and approximately six (P-A2, P-B2)/three (L-B2) weeks later are shown. Titres ≤ 3 log2 are considered negative. Different letters (a–d) indicate statistically significant differences (P < 0.05).
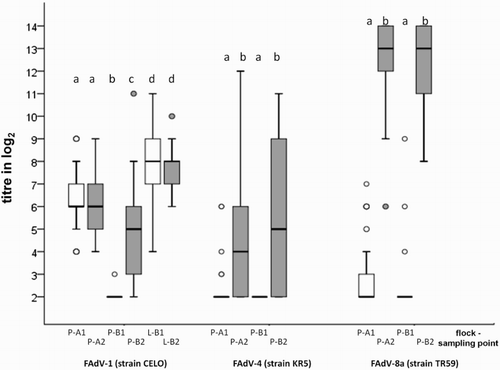
In the layer flock L-B, neutralizing antibodies against FAdV-1 were found in all samples tested, with no significant difference in the arithmetic mean of titres at different sampling points (mean ± std = 8.00 ± 1.86 log2 at the time post-mortem examinations revealed gizzard erosions; mean ± std = 7.58 ± 1.16 log2 at the sampling point approximately 3 weeks later).
Pathogenicity of FAdV-1 and FAdV-8a in 21-day-old SPF pullets
No clinical signs were observed in any of the birds during the in vivo study. Macroscopically, characteristic roughening of the gizzard koilin layer and mucosal inflammation were only observed in birds infected with FAdV-1 () from 7 DPI onwards. None of these birds showed dark brown contents or deep ulceration/perforation of the gizzards, as observed in some of the field cases. Prominent histopathological changes in FAdV-1 infected birds correlated with observations from the field cases (necrosis, degeneration and loss of epithelial cells accompanied by mild to severe inflammation of the lamina propria, submucosa and the muscular layer). Basophilic intranuclear inclusion bodies were only observed at 7 DPI in affected gizzard epithelial cells. ISH confirmed the presence of FAdV-A DNA associated with intranuclear inclusion bodies, while the FAdV-E probe gave no signal (). No gross pathological changes were observed in other organs of these birds. Birds orally infected with FAdV-8a showed no gross lesions, no pathohistological changes in gizzard or liver and no signal was obtained by ISH. In both infected groups, viral shedding was confirmed by real-time PCR and viral DNA was detected within tissue samples (). Virus neutralizing antibodies in birds inoculated with FAdV-1 and -8a during the in vivo study were detected from 7 DPI onwards with a steady increase in homologous neutralizing antibody titres (3.8–8.5 and 6.6–10.5 log2, respectively; ). No heterologous antibodies were detected in birds from the respective groups.
Figure 5. Box plot presentation of VNT results from in vivo study. Titres (log2) of FAdV-1- and FAdV-8a-specific antibodies from SPF pullets orally infected with FAdV-1 or FAdV-8a. Titres ≤ 3 log2 are considered negative.
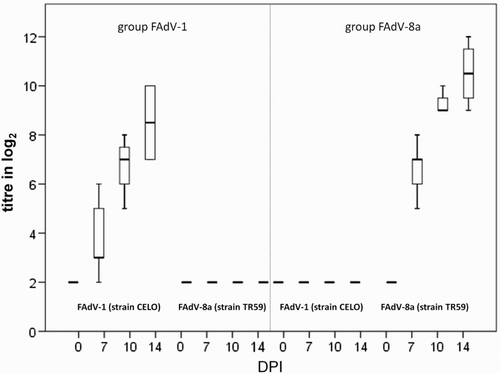
Table 2. Pathological changes in the gizzard during the in vivo study. Twenty-one-day-old SPF pullets orally infected with FAdV-1 or FAdV-8a examined at 7, 10 and 14 DPI.
Table 3. Detection of viral DNA from organs and cloacal swabs in the in vivo study. Samples from 21-day-old SPF pullets orally infected with FAdV-1 or FAdV-8a were investigated at 7, 10 and 14 DPI by real-time PCR.
In the uninfected control group, no gross or pathohistological changes were found. Cloacal swabs and organ samples from control birds examined by real-time PCR were found to be negative. Birds did not develop antibodies against FAdV-1 or -8a during the duration of the experiment.
Discussion
While it is well known, as a result of experimental infection trials in 1–53-day-old chicks (Hess, Citation2013), that SPF layers are susceptible to infection with “pathogenic” FAdV-1 with the subsequent development of AGE there is only limited knowledge about the disease in commercial pullet and layer flocks. Between 2009 and 2016 many cases of AGE affecting free range layer flocks, and more recently also young replacement pullets and layers housed in enriched cage systems, have been recorded in Great Britain. The outbreaks of AGE in two consecutive layer flocks on one farm suggest that environmental contamination and subsequent horizontal transmission to newly placed hens may play a significant role in the epidemiology of the condition in the field. Horizontal transmission of FAdV-1 and the subsequent development of gizzard erosions have been confirmed in an experimental model (Ono et al., Citation2007). The increasing number of reports of AGE in pullets and layers may be attributed to overall changes in husbandry systems, free range and barn systems replacing traditional cage systems, where horizontal transmission of the causative agent between and within flocks might be facilitated more easily.
The pullet and layer cases in the present study were reported and investigated due to increased mortality (0.12–0.30% per week). Prior to death, the affected birds did not show any distinct premonitory clinical signs. In general, elevated mortality was reported for a period of 2–3 weeks and the cumulative mortality in the flocks was up to 1.07%. Similar increases in mortality have been reported from pullet and layer flocks which were diagnosed with AGE previously (Tanimura et al., Citation1993; Lim et al., Citation2012; Domanska-Blicharz et al., Citation2015; Totsuka et al., Citation2015; Matczuk et al., Citation2017). Mortality in natural outbreaks seems to be caused by deep ulceration and even perforation of the gizzard accompanied by substantial haemorrhage. Although in some cases mortality has been observed in broiler flocks in the course of a FAdV-1 infection (Schade et al., Citation2013), the majority of documented AGE outbreaks in meat-producing chickens were presented due to growth retardation or condemnation of gizzards at slaughter in otherwise healthy birds (Ono et al., Citation2001, Citation2003; Manarolla et al., Citation2009; Domanska-Blicharz et al., Citation2011; Grafl et al., Citation2012). In the present study, the flocks investigated did not show signs of growth retardation and/or unevenness, which is likely to be more evident in meat type birds due to the genetic traits of the bird types concerned. Furthermore, it may be hypothesized that the higher numbers of losses tolerated in broiler flocks compared to egg-producing chickens could mask fatalities due to AGE and is therefore a less noticeable parameter in broilers than the substantial growth retardation.
Due to the fact that most AGE outbreaks have been described in meat-producing chickens and experimental studies with FAdV-1 to reproduce the disease were exclusively conducted in juvenile broiler or SPF layer chickens, data on the influence of an AGE outbreak on production parameters of commercial layers are limited. No decrease in egg production was reported during one case of vertical transmission of FAdV-1 from 27- to 32 weeks old broiler breeders to their broiler progeny resulting in AGE outbreaks with substantial economic losses on multiple broiler farms (Grafl et al., Citation2012). Similarly, no influence on egg production or egg quality was observed in 24- and 30 weeks old layer flocks with AGE in Poland (Domanska-Blicharz et al., Citation2015). Other literature however indicates a drop in egg production of up to 5% and/or a negative impact of AGE on the egg size (Totsuka et al., Citation2015; Matczuk et al., Citation2017). In the present study, a slight decrease in egg production was noticed concurrent with the appearance of AGE in flock L-A, when the birds were about to attain peak of egg production. We hypothesize that FAdV-1 infections occurring at the early part of the laying cycle, when birds are almost in full production, might have a more damaging effect on laying performance. However, a number of non-infectious and infectious factors may also influence the egg production of commercial birds; thus in order to gather significant data, this assumption needs to be addressed in an experimental setting. Nonetheless, the negative impact on pullet and layer health and production documented shows the importance of monitoring and diagnosis of AGE in layer-type birds.
Pathomorphological changes in the course of AGE in the commercial pullets and layers investigated were confined exclusively to the gizzards and correlated well with previous descriptions (Hess, Citation2013). The virological investigations conducted on frozen gizzard samples (single or pooled) and/or FFPE tissues confirmed the involvement of FAdV-1 in all 15 cases of AGE in commercial pullet and layer flocks. Histopathological changes of the affected gizzards, and in particular, intranuclear inclusion bodies, were directly attributed to infection with FAdV-A following the use of a species-specific in situ DNA probe. The isolated FAdV-1 strains of this study, which shared 100% sequence identity in the hexon and fibre gene regions examined, also demonstrated high nucleotide homology within analysed portions of the long and short fibre gene to “pathogenic” isolates, characterized from other gizzard erosion outbreaks (Marek et al., Citation2010; Domanska-Blicharz et al., Citation2015). In addition, characteristic gross and histopathological changes in the gizzard could only be reproduced in an in vivo study in 21-day-old SPF pullets that were orally infected with the isolated FAdV-1 strain, but not with the FAdV-8a strain. Thus, the aetiological and pathological properties of the detected and isolated FAdV-1 in the context of AGE were confirmed.
Serological investigations of the affected commercial pullet and layer flocks (P-A, L-B) were not as conclusive. Both flocks had neutralizing antibodies at the first sampling time point, when post-mortem examinations revealed gizzard erosions. Neither flock showed a rise in FAdV-1 neutralizing antibodies in samples taken 3/6 weeks apart from the episode of gizzard erosions. As the affected pullet flock was 41 days old at the first sampling, it is unlikely that the antibodies detected were residual maternal antibodies, which can generally only be detected within the first 3–4 weeks of life (Okuda et al., Citation2001; Grafl et al., Citation2013). Previous experimental infection studies with SPF birds have shown that neutralizing antibodies usually develop within 5–10 DPI, coinciding with the time frame of the lesion development (Okuda et al., Citation2001; Grafl et al., Citation2013). Unfortunately, no blood samples were available to confirm a negative infection status of the flocks prior to the diagnosis of AGE. While the control pullet flock P-B was serologically negative at the first sampling date, a seroconversion against FAdV-1 was documented at the second sampling date. However, no signs of AGE were observed in this flock at any point. Nevertheless, simply detecting infection with FAdV-1 or other serotypes by serological and/or molecular biological methods does not in any way confirm it as the causative agent of a condition. Infection with FAdV-1 of clinically normal pullets and layers with no gizzard lesions has been described for a number of years (Yates & Fry, Citation1957; Nakamura et al., Citation2002a; Grafl et al., Citation2014) and the differentiation between “pathogenic” and “non-pathogenic” strains within FAdV-1 has been described at a molecular level, indicating that strains differ in their ability to cause AGE (Okuda et al., Citation2006; Marek et al., Citation2010). Furthermore, it has been suggested that in naturally occurring outbreaks some, so far undisclosed, predisposing factors may be required in order to establish AGE after infection with FAdV-1 (Totsuka et al., Citation2015; Matczuk et al., Citation2017). The concurrent presence of FAdVs of different serotypes and/or species in chicken flocks has also been described several times in the past (Hess, Citation2013). In the present study, both affected and unaffected pullet flocks, P-A and P-B, harboured co-infections of additional serotypes (FAdV-8a and FAdV-4), as confirmed by serological and/or molecular biological methods. As observed for FAdV-1 strains, strains of varying virulence have also been reported for other FAdV serotypes (Hess, Citation2013). Detection of additional FAdVs may be considered incidental and inconsequential to the pathogenesis of AGE; however, aetiological correlation with clinical conditions should be investigated further.
Nakamura et al. (Citation2002b) described gizzard lesions in broilers associated with hepatitis-hydropericardium syndrome, a primary disease of broilers caused by FAdV-4 (Hess, Citation2013). Unfortunately, these authors applied immunohistochemistry which was based on antibodies raised against various serotypes, preventing a clear designation of a specific causative FAdV. However, in general experimental infection studies with virulent FAdV-4 strains have not reported gross lesions in the gizzard (Hess, Citation2013). In the present study, no paraffin-embedded tissue from the single bird harbouring FAdV-4 in the gizzard was available for histological investigations.
An outbreak of gizzard erosions diagnosed from broilers in the course of slaughter house examinations has been reported concurrent to the isolation of FAdV-8, but gizzard lesions from experimental reproduction studies using a natural infection route were sporadic and quite mild (Okuda et al., Citation2004). In the present study, pullets from two of the investigated cases were harbouring FAdV-E, FAdV-8a in addition to FAdV-1. However, PCR positive gizzards containing FAdV-E DNA did not react with a species-specific ISH. Moreover, the in vivo study in 21-day-old SPF pullets confirmed that while infection with the FAdV-8a strain took place, birds did not develop pathomorphological changes in the gizzard.
In conclusion, our investigations confirmed that FAdV-8a did not play an aetiological role in the development of AGE lesions in commercial pullet flocks, and hence all AGE outbreaks were solely attributed to infection with FAdV-1.
Acknowledgements
We thank Patricia Wernsdorf and Evelyn Berger for their excellent technical support. We further thank Ian Cameron for providing laying flock samples and background information of affected flocks and to the veterinary practices who submitted the samples which were used for the retrospective investigation of cases.
Disclosure statement
No potential conflict of interest was reported by the authors.
References
- Domanska-Blicharz, K., Bartczak, R., Opolska, J., Lisowska, A. & Minta, Z. (2015). Adenoviral gizzard erosions in commercial layer chickens. In H. Swart (Ed.), Proceedings of the 19th World Veterinary Poultry Association Congress (pp. 464–468). Cape Town: WVPA.
- Domanska-Blicharz, K., Tomczyk, G., Smietanka, K., Kozaczynski, W. & Minta, Z. (2011). Molecular characterization of fowl adenoviruses isolated from chickens with gizzard erosions. Poultry Science, 90, 983–989. doi: 10.3382/ps.2010-01214
- Grafl, B., Aigner, F., Liebhart, D., Marek, A., Prokofieva, I., Bachmeier, J. & Hess, M. (2012). Vertical transmission and clinical signs in broiler breeders and broilers experiencing adenoviral gizzard erosion. Avian Pathology, 41, 599–604. doi: 10.1080/03079457.2012.740614
- Grafl, B., Liebhart, D., Günes, A., Wernsdorf, P., Aigner, F., Bachmeier, J. & Hess, M. (2013). Quantity of virulent fowl adenovirus serotype 1 correlates with clinical signs, macroscopical and pathohistological lesions in gizzards following experimental induction of gizzard erosion in broilers. Veterinary Research, 44, 38. doi: 10.1186/1297-9716-44-38
- Grafl, B., Prokofieva, I., Wernsdorf, P., Steinborn, R. & Hess, M. (2014). Infection with an apathogenic fowl adenovirus serotype-1 strain (CELO) prevents adenoviral gizzard erosion in broilers. Veterinary Microbiology, 172, 177–185. doi: 10.1016/j.vetmic.2014.05.020
- Günes, A., Marek, A., Grafl, B., Berger, E. & Hess, M. (2012). Real-time PCR assay for universal detection and quantitation of all five species of fowl adenoviruses (FAdV-A to FAdV-E). Journal of Virological Methods, 183, 147–153. doi: 10.1016/j.jviromet.2012.04.005
- Hess, M. (2013). Aviadenovirus infections. In D.E. Swayne, J.R. Glisson, L.R. McDougald, L.K. Nolan, D.L. Suarez & V. Nair (Eds.), Diseases of Poultry 13th edn (pp. 290–300). Ames: Wiley-Blackwell.
- Kant, A., Balk, F., Born, L., van Roozelaar, D., Heijmans, J., Gielkens, A. & ter Huurne, A. (2003). Classification of Dutch and German avian reoviruses by sequencing the sigma C protein. Veterinary Research, 34, 203–212. doi: 10.1051/vetres:2002067
- Liebhart, D., Weissenböck, H. & Hess, M. (2006). In-situ hybridization for the detection and identification of Histomonas meleagridis in tissues. Journal of Comparative Pathology, 135, 237–242. doi: 10.1016/j.jcpa.2006.08.002
- Lim, T.H., Kim, B.Y., Kim, M.S., Jang, J.H., Lee, D.H., Kwon, Y.K., Lee, J.B., Park, S.Y., Choi, I.S. & Song, C.S. (2012). Outbreak of gizzard erosion associated with fowl adenovirus infection in Korea. Poultry Science, 91, 1113–1117. doi: 10.3382/ps.2011-02050
- Manarolla, G., Pisoni, G., Moroni, P., Gallazzi, D., Sironi, G. & Rampin, T. (2009). Adenoviral gizzard erosions in Italian chicken flocks. Veterinary Record, 164, 754–756. doi: 10.1136/vr.164.24.754
- Marek, A., Schulz, E., Hess, C. & Hess, M. (2010). Comparison of the fibers of fowl adenovirus a serotype 1 isolates from chickens with gizzard erosions in Europe and apathogenic reference strains. Journal of Veterinary Diagnostic Investigation, 22, 937–941. doi: 10.1177/104063871002200613
- Matczuk, A.K., Niczyporuk, J.S., Kuczkowski, M., Woźniakowski, G., Nowak, M. & Wieliczko, A. (2017). Whole genome sequencing of fowl aviadenovirus A – a causative agent of gizzard erosion and ulceration, in adult laying hens. Infection, Genetics and Evolution, 48, 47–53. doi: 10.1016/j.meegid.2016.12.008
- Matos, M., Grafl, B., Liebhart, D., Schwendenwein, I. & Hess, M. (2016). Selected clinical chemistry analytes correlate with the pathogenesis of inclusion body hepatitis experimentally induced by fowl aviadenoviruses. Avian Pathology, 45, 520–529. doi: 10.1080/03079457.2016.1168513
- Meulemans, G., Boschmans, M., Van den Berg, T.P. & Decaesstecker, M. (2001). Polymerase chain reaction combined with restriction enzyme analysis for detection and differentiation of fowl adenoviruses. Avian Pathology, 30, 655–660. doi: 10.1080/03079450120092143
- Nakamura, K., Ohyama, T., Yamada, M., Abe, T., Tanaka, H. & Mase, M. (2002a). Experimental gizzard erosions in specific-pathogen-free chicks by serotype 1 group I avian adenoviruses from broilers. Avian Diseases, 46, 893–900. doi: 10.1637/0005-2086(2002)046[0893:EGEISP]2.0.CO;2
- Nakamura, K., Tanaka, H., Mase, M., Imada, T. & Yamada, M. (2002b). Pancreatic necrosis and ventricular erosion in adenovirus-associated hydropericardium syndrome of broilers. Veterinary Pathology, 39, 403–406. doi: 10.1354/vp.39-3-403
- Okuda, Y., Ono, M., Shibata, I. & Sato, S. (2004). Pathogenicity of serotype 8 fowl adenovirus isolated from gizzard erosions of slaughtered broiler chickens. Journal of Veterinary Medical Science, 66, 1561–1566. doi: 10.1292/jvms.66.1561
- Okuda, Y., Ono, M., Shibata, I., Sato, S. & Akashi, H. (2006). Comparison of the polymerase chain reaction-restriction fragment length polymorphism pattern of the fiber gene and pathogenicity of serotype-1 fowl adenovirus isolates from gizzard erosions and from feces of clinically healthy chickens in Japan. Journal of Veterinary Diagnostic Investigation, 18, 162–167. doi: 10.1177/104063870601800204
- Okuda, Y., Ono, M., Yazawa, S., Shibata, I. & Sato, S. (2001). Experimental infection of specific-pathogen-free chickens with serotype-1 fowl adenovirus isolated from a broiler chicken with gizzard erosions. Avian Diseases, 45, 19–25. doi: 10.2307/1593007
- Ono, M., Okuda, Y., Shibata, I., Sato, S. & Okada, K. (2007). Reproduction of adenoviral gizzard erosion by the horizontal transmission of fowl adenovirus serotype 1. Journal of Veterinary Medical Science, 69, 1005–1008. doi: 10.1292/jvms.69.1005
- Ono, M., Okuda, Y., Yazawa, S., Shibata, I., Sato, S. & Okada, K. (2003). Outbreaks of adenoviral gizzard erosion in slaughtered broiler chickens in Japan. Veterinary Record, 153, 775–779.
- Ono, M., Okuda, Y., Yazawa, S., Shibata, I., Tanimura, N., Kimura, K., Haritani, M., Mase, M. & Sato, S. (2001). Epizootic outbreaks of gizzard erosion associated with adenovirus infection in chickens. Avian Diseases, 45, 268–275. doi: 10.2307/1593040
- Reed, L.J. & Muench, H. (1938). A simple method of estimating fifty percent endpoints. American Journal of Epidemiology, 27, 493–497. doi: 10.1093/oxfordjournals.aje.a118408
- Schachner, A., Marek, A., Grafl, B. & Hess, M. (2016). Detailed molecular analyses of the hexon loop-1 and fibers of fowl aviadenoviruses reveal new insights into the antigenic relationship and confirm that specific genotypes are involved in field outbreaks of inclusion body hepatitis. Veterinary Microbiology, 186, 13–20. doi: 10.1016/j.vetmic.2016.02.008
- Schade, B., Schmitt, F., Böhm, B., Alex, M., Fux, R., Cattoli, G., Terregino, C., Monne, I., Currie, R.J.W. & Olias, P. (2013). Adenoviral gizzard erosion in broiler chickens in Germany. Avian Diseases, 57, 159–163. doi: 10.1637/10330-082312-Case.1
- Schat, K.A. & Sellers, H.S. (2008). Cell-culture methods. In L. Dufour-Zaval, D.E. Swayne, J.R. Glisson, J.E. Pearson, W.M. Reed, M.W. Jackwood & P. Woolcock (Eds.), A Laboratory Manual for the Isolation and Identification of Avian Pathogens 5th edn (pp. 195–203). Kennet Square, PA: American Association of Avian Pathologists.
- Tanimura, N., Nakamura, K., Imai, K., Maeda, M., Gobo, T., Nitta, S., Ishihara, T. & Amano, H. (1993). Necrotizing pancreatitis and gizzard erosion associated with adenovirus infection in chickens. Avian Diseases, 37, 606–611. doi: 10.2307/1591697
- Totsuka, M., Goda, M., Isogai, K., Yamamoto, Y., Sukigara, S. & Suzuki, T. (2015). Gizzard erosion of brown layer chicken with fowl adenovirus infection. Journal of Japanese Society for Poultry Diseases, 51, 11–12.
- Yates, V. J. & Fry, D. E. (1957). Observations on a chicken embryo lethal orphan (CELO) virus. American Journal of Veterinary Research, 18, 657–660.