ABSTRACT
Clinicopathological diagnosis of mucopolysaccharidosis type IIIB (MPS IIIB; Sanfilippo syndrome B), an inherited autosomal recessive lysosomal storage disease, as a cause of losses in a commercial emu flock and screening breeders using a mutation-specific DNA test are described. Between 2012 and 2015, ∼5–10 juvenile emus from a few weeks to several months of age developed progressive neurological signs and died while others in the flock remained healthy. Necropsy of two affected siblings revealed multiple sites of haemorrhage, cytoplasmic periodic acid-Schiff and Luxol fast blue-positive inclusions in neurons, and aggregates of foamy macrophages in visceral organs. Affected emus were homozygous for the two-base deletion in the α-N-acetylglucosaminidase gene that causes MPS IIIB in emus. Mutation-specific DNA tests for MPS IIIB in emus were developed. Screening blood samples from 78 breeding emus revealed 14 (18%; 9 males, 4 females, and 1 unknown gender) carriers; an overall 0.09 mutant α-N-acetylglucosaminidase allele frequency. A “test and cull male carriers” programme, in which carrier males are culled but carrier females are retained, was proposed to avoid breeding affected emus together, ultimately eliminating the disease from future broods, and preserving the gene pool with as much breeding stock as possible. Molecular genetic diagnostic tests are simple, precise, and permit screening of all breeders for the mutant allele in any flock and can be used to eliminate MPS IIIB-related emu losses through informed breeding.
Introduction
Emus (Dromaius novaehollandiae) are primarily raised commercially for their oil, which is used for medicinal (pain control, wound healing, and musculoskeletal disorders) and cosmetic products. Potentially less-allergenic, low-fat red meat and egg products, leather, feathers, eggshells, nails, bones, and manure are additional marketable products (Minnaar & Minnaar, Citation1992; Whitehouse et al., Citation1998; Sales, Citation2007; Maehashi et al., Citation2010). Emus are typically processed at around 18 months of age; their value is approximately USD500–800 depending on the market, breeding value, size of the bird, and pounds of harvestable fat. In this respect, modern commercial ratite farming more closely resembles cattle production with long-term investment before market as well as an inability to effect rapid genetic change.
Emus are generally healthy, but are susceptible to many of the same viral, bacterial, fungal, and parasitic infections, toxicities, nutritional deficiencies, and management errors that affect a broad range of avian species, including poultry (Tully & Shane, Citation1996; Shane, Citation1998; Kummrow, Citation2015). Only one hereditary disorder is known to affect emus: a lysosomal storage disease that was initially thought to be a gangliosidosis (Bermudez et al., Citation1995, Citation1997; Kim et al., Citation1996; Freischütz et al., Citation1997). Subsequently, the disease was determined to be mucopolysaccharidosis type IIIB (MPS IIIB), also known as Sanfilippo syndrome B (Aronovich et al., Citation2001; Palmieri et al., Citation2015). The prevalence of MPS IIIB has not been assessed in any flocks and its economic impact is unknown.
Mucopolysaccharidoses are a group of inherited metabolic diseases characterized by defective or missing enzymes that catabolize glycosaminoglycans (GAGs; formerly known as mucopolysaccharides). Inability to degrade GAGs enzymatically leads to their progressive accumulation in lysosomes and impaired cell function (Haskins, Citation2007; Haskins & Giger, Citation2008). MPS IIIB in emus is caused by a deletion (c.1098_1099delGG) in the α-N-acetylglucosaminidase (NAGLU) gene, which results in a severe deficiency of this lysosomal acid hydrolase activity and heparan sulphate accumulation (Aronovich et al., Citation2001).
We report here the identification of MPS IIIB as the cause of recurring progressive neurological disease in a commercial emu flock, clinicopathological features of diseased emus, genetic screening of breeding stock using a mutation-specific DNA test, and proposal of a “test and cull male carriers” control strategy to avoid future production of affected emus and economic losses.
Materials and methods
History
A commercial emu flock in central North Carolina was evaluated because of a sporadic, progressive, fatal neurologic disease that had occurred during the previous 2 years. Five to 10 young birds either had died or were euthanized annually resulting in economic losses. Ages at the onset of clinical signs ranged from 30 days to 1 year. Clinical signs began as head tremors and retrograde walking, and progressed to ataxia and recumbency over ∼2 weeks. Adult breeding emus and other young birds were not affected. There was no response to empirical antimicrobial and antiparasitic treatments, and no infectious disease was identified. No cause for the neurologic disease had been identified prior to submission of two affected emu siblings to the NCSU CVM for diagnosis.
Flock
The flock of ∼230 emus comprised 78 adult breeders with the remaining young birds (<18 months) being raised for meat and oil production. Colony breeders were kept on pastures in groups of ∼30 emus with a 1:1 male to female ratio. Additionally, 13 breeder pairs were maintained separately in individual pens. Young emus intended for oil and other markets were kept in three groups of 70–100 birds. A commercial diet formulated for emus and water was provided ad libitum, and there was continuous access to grazing and sandy soil. Emus were not vaccinated, but were regularly treated for parasites with 1% levamisole via water. Investigation of the flock was done as a clinical service provided by the Poultry Health Management Team, North Carolina State University, College of Veterinary Medicine with consent by the producer. Specific animal research study permissions were not required for herd management.
Emus
The index case (Case 1) was an 8-month-old male referred for clinical evaluation and necropsy due to worsening neurologic disease like that seen in previously affected emus on the farm. Physical and neurologic examinations, complete blood count, serum biochemistry, and magnetic resonance imaging of the head and upper neck were performed. A female sibling (Case 2) showing the same neurologic signs as the index case was subsequently submitted for euthanasia and necropsy. Additionally, a juvenile emu found recumbent in the field was submitted for euthanasia and necropsy (Case 3). Clinical neurological signs in this emu resulted from traumatic spinal injury without any evidence of storage disease. Tissues from this bird served as normal histologic controls.
Samples
Ethylenediaminetetraacetic acid anticoagulated blood samples and fresh splenic tissue were obtained from the index case, sibling, and traumatically injured emu from the same farm for MPS IIIB genotyping. Subsequently, approximately 1–2 ml of ethylenediaminetetraacetic acid anticoagulated blood samples were collected from the right external jugular vein of 78 breeders and shipped chilled to PennGen at the University of Pennsylvania for genetic screening.
Pathology
Both the index case and sibling were necropsied immediately after euthanasia. Following gross evaluation, the entire brain and samples of spinal cord and visceral organs were placed into 10% neutral buffered formalin, fixed for 72 h, trimmed, embedded in paraffin, sectioned at 5 µm, and stained with haematoxylin and eosin (HE). Selected tissues were stained with periodic acid-Schiff (PAS with and without diastase) and Luxol fast blue (LFB).
Genotyping
Genomic DNA was extracted (DNeasy Blood and Tissue Kit, QIAamp DNA Blood Mini Kit, QIAquick Gel Extraction Kit, Qiagen, Hilden, Germany) and samples were genotyped for the previously reported two-base deletion (c.1098_1099delGG) in NAGLU (Aronovich et al., Citation2001) using direct DNA sequencing, a polymerase chain reaction (PCR)-based restriction fragment length polymorphism (RFLP) assay, and real-time PCR. Genomic DNA surrounding the deletion was amplified by PCR with flanking primers (5ʹ-TCTGCCCAAACTGGCTCCTC-3ʹ and 5ʹ-GTTGATAGCCTCCACGGTGC-3ʹ) using an annealing temperature of 68.5°C. The PCR reaction mixture was prepared to a total volume of 50 µl containing 35.5 µl of DNAse-free water, 5 µl of 10× Ex Taq buffer (Takara Bio Inc., Shiga, Japan), 5 µl of dNTPs buffer (2.5 mM each) (Takara Bio Inc.), 1 µl each of the 50 µM forward and reverse primers, 0.5 µl of a Takara Ex Taq HS polymerase (5 U/µl) (Takara Bio Inc.), and 2 µl of the gDNA template. PCR products were purified for direct DNA sequencing with the above primers. The 2-bp deletion was too small for direct identification by gel electrophoresis of the PCR product. For the PCR–RFLP assay, PCR products were digested for 2 h at 37°C with the restriction enzyme MspI (New England Biolabs Inc., Ipswich, MA, USA) and separated by electrophoresis on a 6% polyacrylamide gel. For screening purposes, a real-time PCR assay was developed using allele-specific TaqMan MGB probes (Applied Biosystems, Foster City, CA, USA) for the wild-type (5ʹ-CAGCCGGACTTCT-3ʹ) and mutant (5ʹ-CACCAGCCACTTCT-3ʹ) alleles and a dedicated primer pair (5ʹ-CAGGGCTGGCTCTTCCAA-3ʹ and 5ʹ-AGCGCCCGCACCT-3ʹ).
Results
Clinical findings
The index case (Case 1), an 8-month-old male emu, presented with head tremors and marked ataxia, but was otherwise bright, alert, and responsive. It became extremely agitated during restraint and examination. The emu was normothermic, mildly tachycardic (∼150 beats per minute), and tachypneic (∼50 breaths per minutes); auscultation was normal. It weighed less than expected (14.6 kg; normal weight range 16–20 kg), but had normal body condition. No muscle atrophy or other musculoskeletal abnormality that would account for the locomotor problems was identified. Head tremors, marked ataxia, retrograde walking, weak gait, and frequent stumbling and falling indicated a possible cerebellar disease. No lesions that could account for the neurologic signs were identified by magnetic resonance imaging.
The complete blood count revealed a mild regenerative anaemia, leucocytosis, heterophilia, and lymphocytosis (). No leukocyte inclusions were identified. Serum creatine kinase (CK) and lactic dehydrogenase (LDH) activities were mildly increased ().
Table 1. Complete blood count done on admission of an emu (index case) with progressive neurologic disease due to MPS IIIB.
Table 2. Serum biochemistry panel on admission of an emu (index case) with progressive neurologic disease due to MPS IIIB.
Pathology
Gross pathology of the index case showed multifocal, acute, subcapsular hepatic haemorrhages with focal rupture and haemoabdomen, mild regionally extensive subcutaneous haemorrhage along the ventral sternum and body wall, and adequate subcutaneous and visceral adipose tissue. Liver was considered enlarged (470 g; 3.2% of body weight); however, no reference for normal liver size for emus could be found. A large blood clot (446 g) was attached to the torn capsule of the right liver lobe, and ∼60 ml of unclotted blood was in the body cavity. Brain and other organs appeared normal. Gross lesions in the affected sibling emu (Case 2) were similar and included haemoabdomen, hepatic haemorrhages, fibrin and clotted blood on the capsule of the liver, and haematomas in various stages of resolution in the leg muscles, subcutaneous tissues, and body cavity.
Microscopically, neurons throughout the brain, spinal cord, and autonomic ganglia in the adrenal, lung, thyroid, and heart of the index case and sibling (Cases 1 and 2) were markedly swollen. Nissl substance was often located centrally and surrounded the nucleus. Pale cytoplasmic inclusions filled the peripheral cytoplasm and expanded the cell. Cytoplasmic inclusions, most evident in larger neurons but occasionally also identified in astrocytes, were variably positive with PAS, with and without diastase, and stained weakly with LFB (). Liver, spleen (), and gastrointestinal lamina propria (not shown) contained numerous clusters of large macrophages with finely vacuolated cytoplasm. These cells did not stain with PAS or LFB.
Figure 1. Histopathology of emus with MPS IIIB compared to an age-matched control. (A) Emu 3, brain, normal neurons. This emu was euthanized following fracture of the spine. HE. (B) Emu 2, sibling to index case, MPS IIIB, brain. Swollen neurons, Nissl substance crowded around nuclei, and cytoplasm filled with pale staining inclusions are typical of a lysosomal storage disease. HE. (C) Emu 1, index case, MPS IIIB, brain. Cytoplasmic inclusions are moderately stained with periodic acid – Schiff. (D) Emu 1, index case, MPS IIIB, brain. Cytoplasmic inclusions stain weakly with LFB.
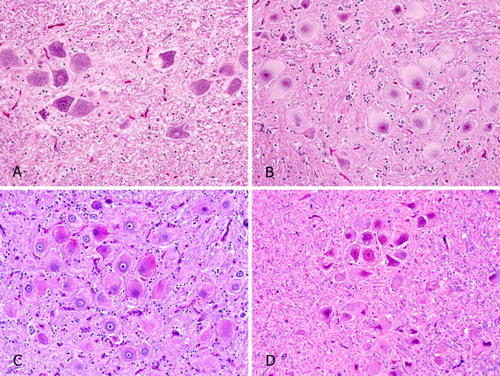
Genotyping for NAGLU mutation
Genomic DNA analysis of the index case and sibling (Cases 1 and 2) showed homozygosity for the two-base deletion in NAGLU (c.1098_1099delGG) by DNA sequencing and PCR–RFLP (), while the emu with trauma from the same flock (Case 3) was homozygous for the normal NAGLU allele.
Figure 3. Polyacrylamide gel electrophoretogram of the PCR- RFLP test to screen for the known MPS IIIB two-base deletion (c.1098_1099delGG) in emus. Amplified DNA band surrounding the mutation is 294 and 292 bp in length in normal and affected emus, respectively. For the normal allele, the product is cleaved with the restriction enzyme MspI at two sites producing three bands (185, 94 and 15 bp). The mutant allele is missing one restriction site, and thus only two bands at 185 and 107 bp are seen. The 15 bp fragment is too small to be detected on the gel. Lanes: M, 100 bp DNA marker; N, normal emu; C, heterozygous carrier; A, affected emu; bp, base pair.
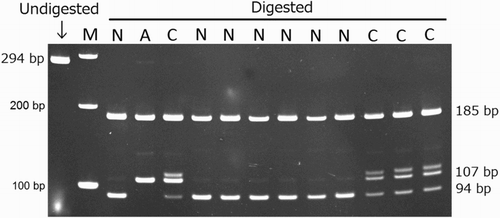
Screening of the 78 clinically healthy adult emu breeders identified 14 emus (nine males, four females, and one unknown gender) as heterozygous for the two-base NAGLU deletion, whereas the others were homozygous for the normal allele by real-time PCR (). Thus, the carrier rate in the flock was 18%, and the mutant allele frequency was 0.09 (or 9%).
Figure 4. Real-time PCR discrimination plots of wild-type and mutant alleles for the two-base deletions (c.1098_1099delGG) in NAGLU. Dots at lower right (red), intermediate (green) and upper left (blue) parts indicate normal, heterozygous carrier, and affected emus, respectively. X indicates heterozygous carriers with an additional missense polymorphism.
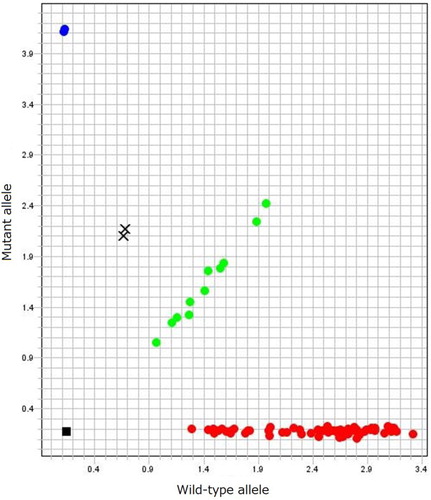
Interestingly, two of the 14 carrier emus showed an unusual amplification pattern by real-time PCR (). Direct DNA sequencing of the region surrounding the two-base deletion in the NAGLU gene showed that both had, in the area where the TaqMan probes hybridize, the same heterozygous single nucleotide polymorphism (SNP; c.1100A > G) at just one base downstream from the known mutation site (). Because only the hybridization of the probe for the wild-type allele was affected, the SNP must exist on the chromosome, which does not have the deletion (wild-type allele). The SNP causes an aspartic acid to glycine substitution (p.Asp367Gly; D367G) but is not predicted to affect the function of the NAGLU enzyme by SIFT analysis (score 0.42 with a range from 1 to 0 and a deleterious score of <0.05) (Ng & Henikoff, Citation2001).
Figure 5. Genomic DNA sequencing chromatograms of a small region surrounding the known two-base deletion in NAGLU gene (c.1098_1099delGG) in a heterozygous bird also having a missense polymorphism (c.1100A > G). A single nucleotide substitution (arrow) adjacent to the known mutation site (dotted line) is predicted to change the amino acid from aspartic acid (Asp, D) to glycine (Gly, G).
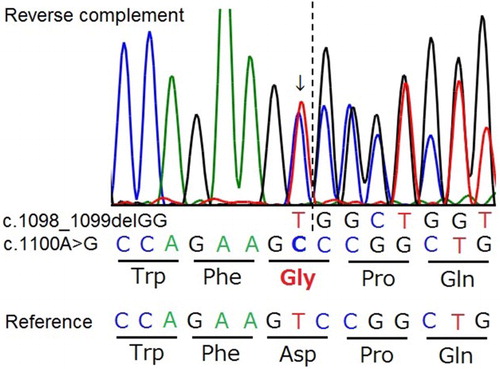
Discussion
The recurring, sporadic, slowly progressing neurologic disease characterized by neurologic signs in emus from this commercial flock was identified as a lysosomal storage disease on histopathology and confirmed by genetic testing to be MPS IIIB. MPS IIIB (Sanfilippo syndrome B) is the first inborn error of metabolism identified in emus and the first to be fully characterised from clinical signs to molecular genetic defect (Aronovich et al., Citation2001). This report describes MPS IIIB in a commercial flock of emus, screening breeding stock for the NAGLU mutation, and proposes a strategy to control the disease through a “test and cull male carriers” program.
A lysosomal storage disease in emus, initially described as a gangliosidosis (Bermudez et al., Citation1995, Citation1997; Freischütz et al. Citation1997), was subsequently found to have massive heparan sulphate storage and a severe deficiency in NAGLU enzyme activity. Identification of a deletion in the NAGLU gene established the disease to be MPS IIIB with secondary ganglioside accumulation. Secondary accumulation of gangliosides in cells occurs with MPS disorders in other animals and people (Walkley & Vanier, Citation2009; Xu et al., Citation2010). However, the precise mechanism for secondary ganglioside accumulation in MPS IIIB remains unknown (Aronovich et al., Citation2001; Haskins & Giger, Citation2008; Palmieri et al., Citation2015).
Several distinct types of MPS disorders are recognized depending on the specific enzyme that is deficient. They form a group of typically autosomal recessive genetic diseases caused by a deficiency of one of the lysosomal enzymes needed to degrade GAGs (Haskins, Citation2007; Haskins & Giger, Citation2008). MPS IIIB specifically results from an inability to degrade heparan sulphate due to missing NAGLU enzyme activity. The resulting accumulation of heparan sulphate in neurons causes severe progressive neurologic dysfunction, although the pathogenesis of why this accumulation leads to neural degradation is unknown (Haskins Citation2007; Walkley & Vanier, Citation2009; Xu et al., Citation2010).
In contrast to other MPS disorders, animals and human patients with MPS IIIB show severe neuropathy without musculoskeletal lesions. Emus may serve as a potential disease model for studying the pathogenesis of this disease in people (Haskins Citation2007).
Affected emus in this flock showed progressive neurologic signs, albeit death before 1 year of age may have resulted from haemorrhage. However, neuronal storage was more severe in brainstem and midbrain nuclei and spinal cord than in cerebellar Purkinje cells. While the storage material in emus studied here was not specifically determined, it was variably PAS positive and weakly LFB positive consistent with GAG and ganglioside accumulation as previously described in emus with MPS IIIB (Palmieri et al., Citation2015).
Bleeding disorders occur in emus and rarely in humans affected with MPS IIIB but the mechanism is unknown. In humans, bleeding disorders are thought to be associated with vasculitis (Aronovich et al., Citation2001; Aydin et al., Citation2006; Palmieri et al., Citation2015). At necropsy, emus showed multisystemic haemorrhage including haemorrhage in the body cavity. Haemorrhage has frequently been reported in juvenile emus with MPS IIIB (Bermudez et al., Citation1995, Citation1997; Aronovich et al., Citation2001; Aydin et al., Citation2006; Palmieri et al., Citation2015). Although the mechanism has yet to be elucidated, a vasculopathy may be involved and heparan sulphate as an anticoagulant may be contributing to the coagulopathy (Aronovich et al., Citation2001; Aydin et al., Citation2006; Palmieri et al., Citation2015). Trauma from incoordination and falling likely further predisposed to the subcutaneous and intramuscular haemorrhages seen in these birds.
Abnormal findings in the bloodwork were not specific but likely reflected secondary changes from bleeding and trauma. Haemorrhage is a frequent finding of MPS IIIB in emus (Palmieri et al., Citation2015). In this bird, the mild anaemia may have resulted from haemorrhage or possibly it occurred secondarily to chronic inflammation. However, the relatively mild anaemia in this emu is not consistent with the massive haemorrhage identified at necropsy. Given its upright and alert posture, the blood sample from this emu could have been drawn prior to the massive and peracute haemorrhagic event. In the face of no discernable toxic change, leukocytosis indicates inflammation, possibly secondary to external trauma or blood extravasation. Hypoalbuminemia supports blood loss as a cause of haematologic changes in this bird. Monocytosis is frequently found in birds with chronic and granulomatous inflammation (Campbell, Citation2015). There was no indication of concurrent infectious disease. The nature of the association between monocytosis and MPS IIIB is unknown. Elevated CK and LDH likely resulted from muscle trauma due to the bird’s incoordination and falling.
Like most lysosomal storage diseases and all Sanfilippo syndromes in other species, MPS IIIB in emus is an autosomal recessive inherited disorder. While the molecular basis of MPS IIIB in emus is defined, DNA screening of emu flocks has not been applied previously. All affected emus that have been tested have had the same DNA mutation indicating a common ancestor. This is not surprising as the majority of emus currently in the commercial breeding pool originated from only a few zoological pairs, and a likely founder emu and inbreeding lead to the spread of the NAGLU mutation (Minnaar & Minnaar, Citation1992). MPS IIIB has not been described from emu populations in other countries or in free-living emus in Australia. There is also no recognized advantage of being heterozygous for the NAGLU mutation, as was suspected for the common CD18 variant in Holstein cattle or RYR1 variant in pigs.
DNA screening of the commercial flock in this study showed a high prevalence of the mutant NAGLU allele (0.09) with nearly 20% of breeders being carriers. The frequency of the MPS IIIB mutation in the emu population is unknown, but MPS IIIB has been described in different parts of the USA. For this farm, a “test and cull male carriers” program was proposed in an attempt to preserve the gene pool and breeders and eliminate the disease from the flock over two or more generations. All heterozygous carrier males were to be culled, while carrier females would be paired with males tested clear for the mutant allele. Removing only the males and keeping the females would maintain the breeding potential of the flock and maintain most of the genetic diversity. It was further recommended to do the same testing of offspring retained as potential breeders in future years and then to select the clear males and females in future breeding, but there is no follow up at this time. Lack of follow up may be related to an unawareness of the power of genetic screening and economic losses by the MPS IIIB disease. Currently, there are no large surveys like the farm described here; however, it seems ethical, prudent, and economical to screen emus for the NAGLU to avoid bird suffering and financial losses. Therefore, genetic screening is recommended for any emu flock with affected birds and/or carriers in North America to assure no affected progeny are produced and young emus are not lost. Combining MPS IIIB testing with screening to determine gender in young emus or even for quality trait loci may be possible in the future.
While blood samples from the breeders and tissues from necropsied birds were used as DNA sources for screening emus for the NAGLU mutation in this study, immature feathers containing pulp or dried blood spots might be simpler to collect and yet serve as well. Dried blood spots have been used for economical diagnostic screening of people in developing countries to identify a variety of lysosomal diseases including MPS IIIB (Verma et al., Citation2017). Cheek swabs or cytobrushes and dried blood spot samples are used routinely for genetic screening of dogs, cats, and people and could work in emus.
Screening samples from this flock for the MPS IIIB NAGLU mutation using a simple real-time PCR assay developed in this study identified two carrier emus with an abnormal TaqMan pattern for the wild-type/normal allele. DNA sequencing revealed the presence of a SNP very close to the position of the two-base deletion affecting the PCR product. Indeed, while real-time PCR is a quick and accurate test, it is not rare to have additional DNA changes in the amplified DNA as described in the instructions for Applied Biosytem TaqMan® SNP Genotyping Assays. Finding the SNP is of interest but did not affect the genotyping. Its biological importance is presently unknown but predicted to be insignificant based on in vitro model analysis of the NAGLU variant.
In conclusion, a method of genetic testing to accurately and quickly test many birds and identify carriers of the NAGLU mutation that causes MPS IIIB was developed in response to the disease in this emu flock. DNA screening should be considered for all commercial emu flocks in North America, especially if they have juvenile emus that die following clinical signs of progressive neurologic disease or evidence of substantial haemorrhage. By instituting a DNA screening and “test and cull male carriers” breeding program, it could be possible to eliminate MPS IIIB from any captive emu population.
Acknowledgements
The authors thank Dr Luke Borst for providing photomicrographs of PAS and LFB stained brain from the index case.
Disclosure statement
No potential conflict of interest was reported by the authors. The PennGen lab, under the direction of Dr Urs Giger is a not-for-profit organization that offers limited screening of emus for MPS IIIB using a DNA test that identifies the specific mutation that causes MPS IIIB. It is important to test emu breeders and remove carriers to eliminate the disease.
Additional information
Funding
References
- Aronovich, E.L., Johnston, J.M., Wang, P., Giger, U. & Whitley, C.B. (2001). Molecular basis of mucopolysaccharidosis type IIIB in emu (Dromaius novaehollandiae): an avian model of Sanfilippo syndrome type B. Genomics, 74, 299–305. doi: 10.1006/geno.2001.6552
- Aydin, M., Akarsu, S., Kabakus, N. & Akpolat, N. (2006). Mucopolysaccharidosis IIIB, cerebral vasculopathy and recurrent subdural hematoma. Indian Pediatrics, 43, 437–440.
- Bermudez, A.J., Freischütz, B., Yu, R.K., Nonneman, D., Johnson, G.S., Boon, G.D., Stogsdill, P.L. & Ledoux, D.R. (1997). Heritability and biochemistry of gangliosidosis in emus (Dromaius novaehollandiae). Avian Diseases, 41, 838–849. doi: 10.2307/1592337
- Bermudez, A.J., Johnson, G.C., Vanier, M.T., Schröder, M., Suzuki, K., Stogsdill, P.L., Johnson, G.S., O’Brien, D., Moore, C.P. & Fry, W.W. (1995). Gangliosidosis in emus (Dromaius novaehollandiae). Avian Diseases, 39, 292–303. doi: 10.2307/1591870
- Campbell, T. (2015). Exotic Animal Hematology and Cytology 4th edn (pp. 56–57). Ames, IA: Wiley Blackwell.
- Costa, N.D., McDonald, D.E. & Swan, R.A. (1993). Age-related changes in plasma biochemical values of farmed emus (Dromaius novaehollandiae). Australian Veterinary Journal, 70, 341–344. doi: 10.1111/j.1751-0813.1993.tb00880.x
- Freischütz, B., Tokuda, A., Ariga, T., Bermudez, A.J. & Yu, R.K. (1997). Unusual gangliosidosis in emu (Dromaius novaehollandiae). Journal of Neurochemistry, 68, 2070–2078. doi: 10.1046/j.1471-4159.1997.68052070.x
- Fudge, A. M. (1996). Clinical hematology and chemistry of ratites. In T.N. Tully, Jr. & S.M. Shane (Eds.), Ratite Management, Medicine, and Surgery (pp. 105–114). Malabar, FL: Krieger Publishing Company.
- Haskins, M. (2007). Animal models for mucopolysaccharidoses disorders and their clinical relevance. Acta Paediatrica, 96, 56–62. doi: 10.1111/j.1651-2227.2007.00211.x
- Haskins, M. & Giger, U. (2008). Lysosomal storage diseases. In J.J. Kaneko, J.W. Harvey & M.L. Bruss (Eds.), Clinical Biochemistry of Domestic Animals 6th edn (pp. 731–742). San Diego, CA: Academic Press.
- Kim, D.Y., Cho, D.Y. & Taylor, H.W. (1996). Lysosomal storage disease in an emu (Dromaius novaehollandiae). Veterinary Pathology, 33, 365–366. doi: 10.1177/030098589603300416
- Kummrow, M.S. (2015). Ratites or Struthioniformes: Struthiones, Rheae, Cassuarii, Apteryges (ostriches, rheas, emus, cassowaries, and kiwis), and Tinamiformes (tinamous). In R.E. Miller & M.E. Fowler (Eds.), Zoo and Wild Animal Medicine 8th edn (pp. 75–81). St. Louis, MO: Elsevier.
- Maehashi, K., Matano, M., Irisawa, T., Uchino, M., Itagaki, Y., Takano, K., Kashiwagi, Y. & Watanabe, T. (2010). Primary structure of potential allergenic proteins in emu (Dromaius novaehollandiae) egg white. Journal of Agricultural and Food Chemistry, 58, 12530–12536. doi: 10.1021/jf103239v
- Minnaar, P. & Minnaar, M. (1992). The Emu Farmer’s Handbook. Groveton, TX: Induna Co.
- Ng, P.C. & Henikoff, S. (2001). Predicting deleterious amino acid substitutions. Genome Research, 11, 863–874. doi: 10.1101/gr.176601
- Palmieri, C., Giger, U., Wang, P., Pizarro, M. & Shivaprasad, H.L. (2015). Pathological and biochemical studies of mucopolysaccharidosis type IIIB (Sanfilippo syndrome type B) in juvenile emus (Dromaius novaehollandiae). Veterinary Pathology, 52, 160–169. doi: 10.1177/0300985814529314
- Sales, J. (2007). The emu (Dromaius novaehollandiae): a review of its biology and commercial products. Avian and Poultry Biology Reviews, 18, 1–20. doi: 10.3184/147020607X245048
- Shane, S.M. (1998). Infectious diseases and parasites of ratites. Veterinary Clinics of North America, Food Animal Practice, 14, 455–483. doi: 10.1016/S0749-0720(15)30231-0
- Tully, T.N. & Shane, S.M. (1996). Husbandry practices as related to infectious and parasitic diseases of farmed ratites. Revue Scientifique et Technique, 15, 73–89. doi: 10.20506/rst.15.1.916
- Verma, J., Thomas, D.C., Kasper, D.C., Sharma, S., Puri, R.D., Bijarnia-Mahay, S., Mistry, P.K. & Verma, I.C. (2017). Inherited metabolic disorders: efficacy of enzyme assays on dried blood spots for the diagnosis of lysosomal storage disorders. Journal of Inherited Metabolic Disease Reports, 31, 15–27.
- Walkley, S.U. & Vanier, M.T. (2009). Secondary lipid accumulation in lysosomal disease. Biochimica et Biophysica Acta, 1793, 726–736. doi: 10.1016/j.bbamcr.2008.11.014
- Whitehouse, M.W., Turner, A.G., Davis, C.K. & Roberts, M.S. (1998). Emu oil(s): a source of non-toxic transdermal anti-inflammatory agents in aboriginal medicine. Inflammopharmacology, 6, 1–8. doi: 10.1007/s10787-998-0001-9
- Xu, Y.H., Barnes, S., Sun, Y. & Grabowski, G.A. (2010). Multi-system disorders of glycosphingolipid and ganglioside metabolism. Journal of Lipid Research, 51, 1643–1675. doi: 10.1194/jlr.R003996