ABSTRACT
Avian influenza viruses have been isolated from many bird species; however, little is known about the susceptibility of pet birds to low pathogenic avian influenza (LPAI) viruses. To address this research gap, domestic canaries (Serinus canaria forma domestica) were experimentally infected with H5 and H7 LPAI viruses to determine susceptibility and to evaluate samples for diagnostic purposes. Clinical evidence of infection (e.g. ruffled plumage and apathy) and mortality were noted for the canaries inoculated with chicken-adapted LPAI viruses. Real-time reverse transcription-polymerase chain reaction (RRT-PCR) demonstrated higher viral RNA levels in buccal compared to faecal samples. No clinical signs or mortality were observed in canaries inoculated with LPAI virus originating from wild birds; however, the canaries in this group did have evidence of viral RNA in buccal and faecal samples. Overall, this study showed that domestic canaries are susceptible to LPAI virus infections and that they can shed large amounts of viral RNA, primarily through the respiratory route. Thus, buccal swabs might be better samples than faeces for efficient detection of some LPAI virus infections in these birds. Although canaries have not been identified as a significant reservoir for LPAI viruses, they may be infected by LPAI viruses. Thus, the importance of the control of domestic canaries for detection of LPAI viruses should not be underestimated, especially in the contexts of international commercial exchange and outbreaks.
RESEARCH HIGHLIGHTS
Canaries are susceptible to infection with H5/H7 LPAI viruses.
Canaries inoculated with LPAI viruses excrete large amounts of viral RNA.
Buccal swabs may be appropriate specimens for AI virus detection in canaries.
The control of canaries for LPAI virus detection should not be overlooked.
Introduction
Avian influenza (AI) A viruses have been isolated from many wild bird species. Aquatic birds of the Anseriformes (particularly ducks, geese, and swans) and Charadriiformes (particularly gulls, terns, and waders) families are the primary natural reservoirs of LPAI viruses (Fouchier & Munster, Citation2009). AI viruses are also known for their virulence in poultry (especially chickens), where they cause two distinct disease pathotypes. Highly pathogenic avian influenza results from extremely virulent viruses that cause systemic disease with high morbidity and mortality. Low pathogenic avian influenza (LPAI) is caused by viruses that are associated with mild respiratory disease, reduced egg production, and moderate increases in mortality (Webster et al., Citation1992).
Most current information about AI virus replication and bird susceptibility comes from studies of poultry and wild ducks (Alexander, Citation2000, Citation2007; Pantin-Jackwood & Swayne, Citation2009; Brown, Citation2010). Only limited information is available about domestic bird susceptibility to AI. Although some LPAI viruses have been isolated from birds of the Psittacidae family (parrots or budgerigars) (Kawano et al., Citation1979; Yamamoto et al., Citation1979; Senne et al., Citation1983; Mase et al., Citation2001; Perkins & Swayne, Citation2003; Jiao et al., Citation2012; Jones et al., Citation2015), little is known about AI in domestic canaries (Kaleta & Honicke, Citation2005). Domestic canaries are often involved in international commercial exchanges (Boseret et al., Citation2013). Prior to export, these birds are subjected to health controls designed to identify potential infection with viruses, including notifiable AI of H5 and H7 subtypes (World Organization for Animal Health [OIE]; 2005/94/05). These AI subtypes have been identified as being able to be highly pathogenic in poultry (Pantin-Jackwood & Swayne, Citation2009), and the OIE terrestrial manual for AI diagnosis recommends that small birds such as canaries should be assessed using faecal samples when cloacal swabbing is not possible. However, information about canary susceptibility and route of excretion of AI is lacking; thus, it is unknown whether this sampling method is the most appropriate for AI viruses detection. Moreover, the frequent interaction between humans and domestic canaries makes it even more important that canaries be evaluated for their susceptibility to LPAI viruses and their potential role in the spreading of AI viruses.
In this study, domestic canaries (Serinus canaria forma domestica) were experimentally infected with three different H5 and H7 LPAI viruses that were either poultry-adapted or originating from wild birds to: (1) estimate susceptibility to LPAI viruses; (2) determine the route of viral excretion; and (3) determine the most appropriate samples to collect for AI detection.
Materials and methods
Viruses
All viruses used in this study were amplified in 10-day-old embryonated specific pathogen free (SPF) chicken eggs (Lohmann Vahlo, Cuxhaven, Germany), and the harvested allantoic liquid was used as the viral stock for experimental inoculations.
The LPAI viruses used in this study were representative of notifiable LPAI viruses (i.e. H5 or H7 subtype) with different origins (poultry-adapted or wild bird) and were already characterized by experimental infections in SPF chickens (Claes et al., Citation2013).
The LPAI virus H5N2 A/Chicken/Belgium/150VB/99 (H5N2/chicken) was isolated from backyard chickens in 1999 in Belgium (Meulemans et al., Citation1999). A second egg passage of this virus was used to inoculate the canaries.
The LPAI virus H7N1 Italy A/ck/It/1067/v99 (H7N1/chicken) was provided by the laboratory of Dr I. Capua. This virus was received as a third passage and its fifth passage was used to inoculate the canaries.
The LPAI virus H5N3 A/Anas Platyrhynchos/Belgium/09-884/08 (H5N3/wild mallard) was isolated from a cloacal swab sampled from a wild mallard in Belgium in 2008 as a part of annual AI wild bird monitoring (Van Borm et al., Citation2011). A second egg passage of this virus was used to inoculate the canaries.
Experimental inoculation of canaries
Adult canaries (approximately 1-year-old), male and female, were purchased by a local provider (Animalis, Eghezée, Belgium) and kept in biosecurity level 3 (BSL 3) isolators throughout the experiment with ad libitum access to food and water and a water bath refilled every day. Birds were infected after an adaptation period of 1 week. They were divided randomly into four groups with 15–16 birds per group (three inoculated groups and one non-inoculated control group). Each animal experiment was conducted under the authorization and supervision of the Biosafety and Bioethics Committees at the Veterinary and Agrochemical Research center (number 120104-01), following national and European regulations.
Canaries from each of the three experimental groups were inoculated in the eyes, using a viral dose of 106 median egg infective dose in 50 µl. Canaries in the non-inoculated control group were not inoculated, but were kept in identical conditions as inoculated canaries (BSL 3 isolators). Each day, inoculated and non-inoculated birds were observed to monitor and describe clinical signs.
Samples
Minitip swabs (Copan, Brescia, Italy) were used to collect buccal samples from inoculated and non-inoculated canaries and then placed immediately in 0.8 ml brain–heart infusion (BHI) broth containing antibiotics (106 U/l penicillin G, 2 g/l streptomycin, 1 g/l gentamycin, and 650 mg/l kanamycin sulphate). They were stored at −80°C while awaiting further analysis.
Fresh faeces were collected by swabbing fresh faecal material from plastic sheets covering a portion of isolator floors. Collected fresh faecal material was immediately placed in 1.5 ml BHI medium containing antibiotics and stored at −80°C. For each sampling day (described later in the manuscript), five fresh faecal samples were collected individually from each group of inoculated canaries.
Dry faeces were collected at the end of each day by taking as much accumulated faecal material as possible from the 7-hour workday. Samples were stored at −80°C without storage media. For analysis, each dry faecal sample was resuspended at 20% weight/volume (w/v) in BHI medium containing antibiotics. For each sampling day (described later in the manuscript), one bulk dry faecal sample was collected from each group of inoculated canaries.
Drinking water and the water baths were sampled by collecting 1.5 ml each and pouring them into separate, 1.5 ml double-concentrated BHI media containing antibiotics. The samples were immediately stored at −80°C. For each sampling day (described later in the manuscript), one sample each of drinking water and water bath were collected for each group of inoculated canaries. To avoid contamination of the drinking water and water bath by the inoculum, the water sources were disposed at the end of the experimental infection day (day 0) and replaced by fresh water.
All samples were collected at 1, 3, 6, 8, 10, and 14 days post-infection (pi) for each of the four groups. On day 21 pi, all remaining canaries from the three inoculated groups and the one non-inoculated control group were euthanized and their blood was sampled. Collected sera were stored at −20°C until they were used for anti-nucleoprotein antibody detection.
Detection of viral genomic matrix RNA by real-time reverse transcription-polymerase chain reaction (RRT-PCR)
Viral RNA was extracted from 50 µl of samples using the Magmax AI/ND 96 viral RNA kit (Ambion Inc., Austin, Texas) adapted for semi-automated extraction using a Kingfisher magnetic particle processor (Thermo Fisher Scientific, Erembodegem, Belgium). The Quantitect Probe reverse transcriptase polymerase chain reaction kit (QiagenGmBH, Hilden, Germany) was used for amplification in a 7500 real-time PCR cycler (Applied Biosystems, Lennik, Belgium).
Matrix (M) RRT-PCR was used to amplify the viral Matrix gene (M gene), to allow detection of all AI virus subtypes (Steensels et al., Citation2007; Van Borm et al., Citation2007). The cut-off value was fixed at 40 threshold cycles (Ct); consequently, all Ct values greater than 40 were considered negative.
A series of 1:10 dilutions of synthetic matrix RNA was run simultaneously in each RRT-PCR run to calculate the number of RNA copies in each sample. Quantification of the viral RNA in each sample was based on this standard curve, and titres were expressed as M RNA copies/ml. The threshold of detection was considered to correspond to 102.7 viral RNA copies/ml sample medium. For buccal swabs, geometric mean values were calculated using a value of 5 × 102 M RNA copies/ml if the viral RNA was not detected in a sample.
Beta-actin gene expression was used as an internal control for the RRT-PCR to assess sample quality and potential degradation or inhibition (Li et al., Citation2005; Van Borm et al., Citation2011).
Virus isolation
To test for the presence of infectious virus in bird organs, prepared organs were inoculated into 9 to 11-day-old SPF chicken embryos following standard methods (OIE, terrestrial manual 2015). Briefly, most of the organs were prepared in 10% (w/v) phosphate buffer saline with antibiotic (106 U/l penicillin G, 10 g/l streptomycin, and 250 mg/l gentamycin). When the quantity of organs was insufficient, organs were prepared in a minimum volume of 3 ml, regardless of organ weight. Suspensions were then inoculated into the allantoic sacs of 9–11-day-old embryonated SPF chicken eggs. After incubation at 37°C for 5 days, allantoic fluid samples were screened for AI viruses by testing haemagglutinating activity using 1% (v/v) chicken blood.
Nucleoprotein enzyme-linked immunosorbent assay
Enzyme-linked immunosorbent assay (ELISA) was performed using a competitive ELISA kit based on detection of antibodies directed against nucleoprotein (NP) (Influenza A antibody competition ELISA, ID-Vet, Montpellier, France). This kit was developed primarily for use with poultry; because there were no instructions for use with canaries, this ELISA kit was used according to the method indicated for chicken sera, with 10 µl of serum diluted in 90 µl of ELISA buffer. Tests were performed and results were calculated as per the manufacturer's instructions.
Results
Clinical signs and virologic examination of dead birds
In canaries inoculated with the H5N2/chicken virus, lethargy with ruffled plumage was observed in five out of 16 birds from day 1 pi until the end of the period of observation (day 21 pi). Diarrhoea was observed on the floor of the BSL3 isolator between days 1 and 9 pi. Two canaries that showed lethargy died at day 17 pi. Virus detection by egg isolation showed no infectious virus in the organs from these two birds.
In the group of canaries inoculated with the H7N1/chicken, three out of 15 birds had lethargy with rapid respiratory rate and difficult breathing from day 1 to day 8 pi, during which time diarrhoea was observed on the BSL 3 isolator floor. Two of the symptomatic birds died (at days 4 and 7 pi) and were necropsied. Live viruses were detected in the trachea, lung, heart, spleen, liver, kidney, and jejunum/ileum from both birds, and additionally from the duodenum in one of the birds (data not shown). After day 9 pi, all birds in this group were asymptomatic.
In the group of canaries inoculated with the H5N3/wild mallard virus, three out of 15 birds had intermittent lethargy with ruffled plumage from days 1 to 8 pi. No deaths occurred during the 21 days of observation.
RRT-PCR detection of M RNA from buccal swabs
The overall excretion profiles for the three viruses were similar from day 1 to 6 pi, and all the inoculated canaries of the three groups had at least one swab tested positive by RRT-PCR. The geometric mean M RNA concentration was highest on day 3 pi with values of 9 × 105 copies/ml, 2 × 106 copies/ml and 1 × 106 copies/ml for the H5N2/chicken, H7N1/chicken and H5N3/wild mallard groups respectively ((A–C)). Most inoculated canaries from the three groups excreted viral RNA until day 6 pi: 48 of 48 (100%) in the H5N2/chicken group ((A)), 33 of 41 (80%) in the H7N1/chicken group ((B)), and 43 of 45 (95.5%) in the H5N3/wild mallard group ((C)). However, differences in duration of viral RNA excretion were observed after that time. Canaries inoculated with the H5N2/chicken virus had positive swabs at day 8 pi (12 of 16), day 10 pi (3 of 16), and day 14 pi (1 of 16). Birds inoculated with the H7N1/chicken virus had the shortest period of excretion, with two of 12 showing excretion on day 8 pi. The H5N3/wild mallard group excreted viral RNA on day 8 pi (8 of 15), day 10 pi (2 of 15), and day 14 pi (1 of 15).
RRT-PCR viral RNA detection in fresh faeces
Very few fresh faecal samples (1 of 30 on day 6 pi) from the H5N2/chicken group had evidence of viral RNA ((A)) (). The level of detected M RNA was 103 copies/ml ((A)).
In birds inoculated with the H7N1/chicken virus, four of 25 fresh faecal samples were positive for viral RNA: two of five on day 1 pi, one of five on day 3 pi and one of five on day 10 pi ((B)) (). These levels reflect a mean concentration of 103 RNA copies/ml.
In contrast, the H5N3/wild mallard group had 13 of 30 fresh faecal samples tested positive for viral RNA by RRT-PCR: four of five on day 1 pi, four of five on day 3 pi, two of five on day 6 pi, two of five on day 10 pi, and one of five on day 14 pi ((C)) (). The number of M RNA copies/ml ranged from 5 × 102 to 105/ml.
RRT-PCR viral M RNA detection in dry faeces
In the groups of canaries inoculated with chicken-adapted viruses (i.e. H5N2/chicken virus and H7N1/chicken virus), only one of five samples from each group showed evidence of viral M RNA (on days 6 pi and 1 pi, respectively) (). The mean amount of M RNA detected for each group was 103 M RNA copies/ml.
In birds inoculated with the H5N3/wild mallard virus, three of five samples were positive for M RNA by RRT-PCR (days 1, 3, and 6 pi) (). The M RNA copies/ml ranged from 103 to 104.
Figure 1. A, B, C: RT-PCR detection of M RNA from buccal swabs of experimentally inoculated canaries. Buccal swabs were sampled at 1, 3, 6, 8, 10 and 14 days pi and RRT-PCR was used to detect viral M RNA. Results are expressed as M RNA copies/ml from swabs sampled from canaries inoculated with the H5N2/chicken virus ((A)), with the H7N1/chicken virus ((B)) and the H5N3/wild mallard virus ((C)). A dotted line represents the approximate limit of detection; samples where virus was not detected are not shown.
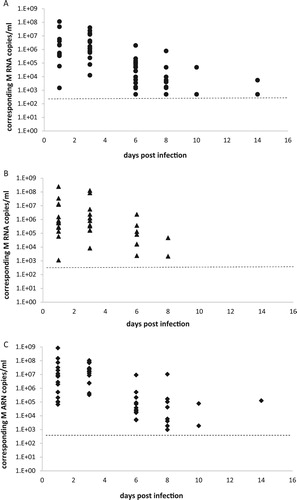
Figure 2. A, B, C: RT-PCR detection of M RNA from fresh and dry faeces. Fresh (cross symbol) and dry (square symbol) faeces were sampled at 1, 3, 6, 8, 10 and 14 days pi and RRT-PCR was used to detect viral M RNA. Results are expressed as M RNA copies/ml from groups of canaries inoculated with the H5N2/chicken virus ((A)), with the H7N1/chicken virus ((B)) and the H5N3/wild mallard virus ((C)). A dotted line represents the approximate limit of detection; samples where virus was not detected were given an arbitrary value of 5.
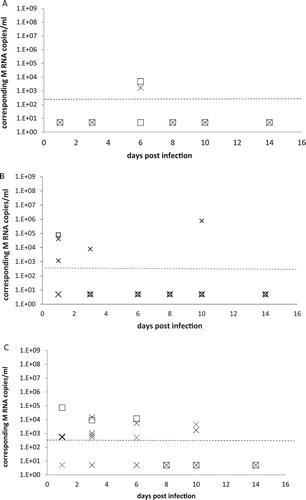
Table 1. Positive samples during the experimental infection of canaries with the H5N2/chicken virus, the H7N1/chicken virus and the H5N3/wild mallard virus.
RRT-PCR viral M RNA detection in water samples. In the H5N2/chicken group, four of six drinking water samples and three of six bath water samples were positive for viral RNA (). The M RNA copies/ml ranged from 5 × 102 to 104 ().
In birds inoculated with the H7N1/chicken virus, three of six drinking water samples and two of six water bath samples showed evidence of viral M RNA (). The associated number of M RNA copies/ml ranged from 5 × 102 to 104 ().
Figure 3. A, B, C: RT-PCR detection of M RNA in drinking water and water bath samples. Water samples from drinking water (light bars) and bath water (dotted bars) were collected at 1, 3, 6, 8, 10 and 14 days pi and RRT-PCR was used to detect viral M RNA. Results are expressed as M RNA copies/ml from groups of canaries inoculated with the H5N2/chicken virus ((A)), with the H7N1/chicken virus ((B)) and the H5N3/wild mallard virus ((C)). A dotted line represents the approximate limit of detection; samples where virus was not detected were given an arbitrary value of 5.
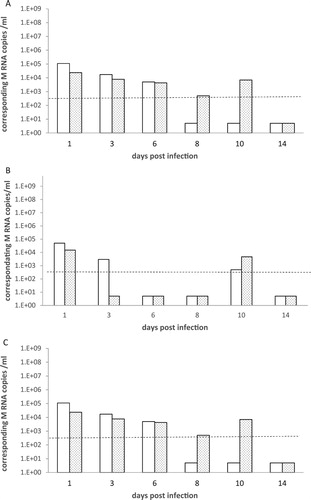
In the group of canaries inoculated with the H5N3/wild mallard, three of six drinking water samples and five of six water bath samples were positive for viral M RNA () at a concentration of 5 × 102 to 105 M RNA copies/ml ().
Figure 4. Detection of anti-NP antibodies by competitive NP ELISA. Detection of anti-NP antibodies was performed using a competitive NP ELISA and results are expressed as % of competition. Group 1 is the non-inoculated control group with non-inoculated canaries; group 2: the canaries inoculated with the H5N2/chicken virus; group 3: the canaries inoculated with the H7N1/chicken virus and the group 4: the canaries inoculated with the H5N3/wild mallard virus.
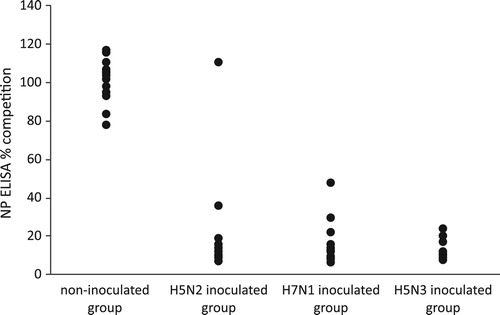
Use of beta-actin gene RNA to confirm sample quality for RRT-PCR tests
To control the sample quality and the presence of inhibitors in RRT-PCR tests, beta-actin gene expression was tested in some buccal swabs and faecal samples from inoculated canaries. During these experimental infections, 97% (226 of 232) of buccal swabs, 92% (83 of 90) of fresh faecal samples, and 81% (13 of 16) of dry faecal samples were positive for beta-actin RNA by RRT-PCR.
Serology results
Sera were tested at 21 days pi using commercial competition NP ELISA detecting anti-NP antibodies. When the profiles of the inoculated birds were compared to those of the uninoculated birds, all but one canary (from H5N2/chicken group) could be considered positive by NP ELISA ().
Discussion
Understanding which hosts allow replication and transmission of AI viruses is important for understanding AI virus epidemiology, risk management, and control. Even if the main AI reservoirs have been well-described, some bird species may serve as occasional or accidental hosts (Achenbach & Bowen, Citation2011; Jones et al., Citation2015; Lenny et al., Citation2015; Yuan et al., Citation2015). Their identification may be important to better understanding AI virus epidemiology, especially with respect to identifying hosts, such as pet birds, that may pose a risk for zoonotic transmission.
Overall, this study provides information about clinical signs, viral excretion in buccal and faecal specimens, viral detection in organs of dead birds, and anti-NP response in canary hosts of LPAI viruses. Virulence and route of excretion observed for the tested viruses were slightly different, which may reflect differences in viral origins. More severe clinical signs and, to some extent, higher number of deaths, were observed in the two groups inoculated with viral strains originating from chickens. The causes of death may be different for these two groups, as canaries inoculated with H5N2/chicken virus died later, and the live virus could not be isolated from their organs. In canaries inoculated with the H7N1/chicken virus, the presence of live virus in several organs suggests systemic infection. Presence of LPAI virus in organs is an unusual observation, yet reports of detection of LPAI viruses in internal organs have already been made in chickens with different LPAI viruses (including the H7N1/chicken strain used in this study) (Post et al., Citation2012; Post et al., Citation2013) and in turkeys with a H7N8 LPAI virus (Pantin-Jackwood et al., Citation2017).
The route of excretion also differed slightly by viral type, with canaries inoculated with virus isolated from wild duck showing higher cloacal shedding compared to those inoculated with virus isolated from chicken. This difference was particularly apparent when comparing fresh faeces, but the lack of difference between groups for dry faeces may be due to the small number of samples collected. However, differences in virulence and route of excretion observed in this study might have been influenced by the experimental inoculation or be specific to the isolates used and need to be confirmed with more data.
It should be noted that H5N3/wild mallard virus appears to replicate better in canaries than in SPF chickens (Claes et al., Citation2013). This result might indicate that, distinct from SPF chickens, no adaptation seemed necessary for a high replication of LPAI viruses originated from wild birds in canaries.
In this study, several types of samples were collected to determine the most appropriate samples for diagnostic purposes and the usefulness of beta-actin gene RNA as a quality control for RRT-PCR to evaluate degradation or inhibition (Li et al., Citation2005; Van Borm et al., Citation2011). Use of internal controls is even more important for canaries, as the handling of these small birds must be done with great care to minimize stress, which could diminish sampling quality, especially for buccal swabs. Based on our results, it does not seem that faecal samples (recommended by OIE for small birds when cloacal swabbing is not possible) are ideal for detecting all LPAI infection in canaries. The number of positive buccal swabs was higher than the number of positive faecal samples (individual or dry faeces) from birds infected with the H5N2/chicken and H7N1/chicken viruses. Moreover, the finding that there were a smaller number of positive results for beta-actin RNA with dry faecal samples (81%) and fresh faecal samples (92%) compared to buccal swabs (97%) suggests that degradation or inhibition may occur in faecal samples. Therefore, buccal swabs might also be considered as appropriate specimens for AI virus detection in canaries and complementary to faecal samples.
Water samples have previously been described as good indicators of the presence of LPAI virus in live poultry markets (Leung et al., Citation2007). However, in the present study, viral RNA detection was sporadic and irregular in drinking and bath water samples, with no clear correlation between viral detection in the two types of water (which are considered by birds as rather equivalent water supply). Lack of correlation between viral detection in water samples and viral shedding was also observed. Altogether, these observations may indicate either presence of RT–PCR inhibition, or degradation of viral RNA in these samples. Moreover, beta-actin gene expression cannot be used to measure quality in this type of sample, as the presence of beta-actin RNA may be not associated with infected bird cells in water samples. Consequently, water samples should be used with caution and only as an alternative or confirmatory sample to determine the absence or presence of LPAI virus infection.
To date, isolation of LPAI viruses from canaries has been infrequent (Kaleta & Honicke, Citation2005) suggesting that canaries are not a significant natural reservoir of LPAI viruses. Our study results suggest, however, that these previous findings may reflect rearing conditions rather than natural resistance. Some authors have suggested the possibility that canaries and other caged birds can become infected through contact with wild birds when they are left outside in the summer for sunbathing (Boseret et al., Citation2013) or during outbreaks on farms (Ssematimba et al., Citation2013). Therefore, control of canaries for detecting LPAI viruses should not be neglected during outbreaks, routine control, or international trading. Moreover, the large amount of viral RNA excreted by infected canaries and the presence of live virus in water (data not shown) indicated that canaries may serve as vectors that spread AI viruses to other species such as humans, as pet birds are known to transmit other zoonotic infections (Boseret et al., Citation2013). Thus, our results raise the question of whether pet birds such as canaries could be potential vectors of emerging LPAI viruses with zoonotic potential, such as H7N9, H10N8, or H9N2 viruses.
Acknowledgements
We thank A. Nadi for his excellent technical assistance and C. Delgrange and M. Vandenbroeck for their excellent animal assistance.
Disclosure statement
No potential conflict of interest was reported by the authors.
Additional information
Funding
Notes
* The work was carried out at Avian Virology & Immunology, Sciensano, rue Juliette Wytsmanstraat 14, 1050 Brussels, Belgium.
Abbreviations: AI: avian influenza; BHI: brain–heart infusion; BSL 3: biosecurity level 3; Ct: threshold cycle; ELISA: enzyme-linked immunosorbent assay; LPAI: low pathogenic avian influenza; M: matrix; NP: nucleoprotein; OIE: World Organization for Animal Health; pi: post-infection; RRT-PCR: real-time reverse transcription-polymerase chain reaction; SPF: specific pathogen free
References
- Achenbach, J.E. & Bowen, R.A. (2011). Transmission of avian influenza A viruses among species in an artificial barnyard. PLoS One, 6, e17643. doi: 10.1371/journal.pone.0017643
- Alexander, D.J. (2000). A review of avian influenza in different bird species. Veterinary Microbiology, 74, 3–13. doi: 10.1016/S0378-1135(00)00160-7
- Alexander, D.J. (2007). Summary of avian influenza activity in Europe, Asia, Africa, and Australasia, 2002-2006. Avian Diseases, 51, 161–166. doi: 10.1637/7602-041306R.1
- Boseret, G., Losson, B., Mainil, J.G., Thiry, E. & Saegerman, C. (2013). Zoonoses in pet birds: review and perspectives. Veterinary Research, 44, 36. doi: 10.1186/1297-9716-44-36
- Brown, I.H. (2010). Summary of avian influenza activity in Europe, Asia, and Africa, 2006-2009. Avian Diseases, 54, 187–193. doi: 10.1637/8949-053109-Reg.1
- Claes, G., Welby, S., van den Berg, T., Van der Stede, Y., Dewulf, J., Lambrecht, B. & Marché, S. (2013). The impact of viral tropism and housing conditions on the transmission of three H5/H7 low pathogenic avian influenza viruses in chickens. Epidemiology and Infection, 141, 2428–2443. doi: 10.1017/S0950268813000125
- Fouchier, R.A. & Munster, V.J. (2009). Epidemiology of low pathogenic avian influenza viruses in wild birds. Revue Scientifique et Technique, 28, 49–58. doi: 10.20506/rst.28.1.1863
- Jiao, P., Song, Y., Yuan, R., Wei, L., Cao, L., Luo, K. & Liao, M. (2012). Complete genomic sequence of an H5N1 influenza virus from a parrot in southern China. Journal of Virology, 86, 8894–8895. doi: 10.1128/JVI.01243-12
- Jones, J.C., Sonnberg, S., Webby, R.J. & Webster, R.G. (2015). Influenza A(H7N9) virus transmission between finches and poultry. Emerging Infectious Diseases, 21, 619–628. doi: 10.3201/eid2104.141703
- Kaleta, E.F. & Honicke, A. (2005). A retrospective description of a highly pathogenic avian influenza A virus (H7N1/Carduelis/Germany/72) in a free-living siskin (Carduelis spinus Linnaeus, 1758) and its accidental transmission to yellow canaries (Serinus canaria Linnaeus, 1758). Deutsch Tierarztliche Wochenschrift, 112, 17–19.
- Kawano, J., Yanagawa, R. & Kida, H. (1979). Site of replication of influenza virus A/budgerigar/Hokkaido/1/77 (Hav 4 Nav 1) in budgerigars. Zentralblatt für Bakteriologie Originale Reihe A, 245, 1–7.
- Lenny, B.J., Shanmuganatham, K., Sonnberg, S., Feeroz, M.M., Alam, S.M., Hasan, M.K., Jones-Engel, L., McKenzie, P., Krauss, S., Webster, R.G. & Jones, J.C. (2015). Replication capacity of avian influenza A(H9N2) virus in pet birds and mammals, Bangladesh. Emerging Infectious Diseases, 21, 2174–2177. doi: 10.3201/eid2112.151152
- Leung, Y.H., Zhang, L.J., Chow, C.K., Tsang, C.L., Ng, C.F., Wong, C.K., Guan, Y. & Peiris, J.S.M. (2007). Poultry drinking water used for avian influenza surveillance. Emerging Infectious Diseases, 13, 1380–1382. doi: 10.3201/eid1309.070517
- Li, Y.P., Bang, D.D., Handberg, K.J., Jorgensen, P.H. & Zhang, M.F. (2005). Evaluation of the suitability of six host genes as internal control in real-time RT-PCR assays in chicken embryo cell cultures infected with infectious bursal disease virus. Veterinary Microbiology, 110, 155–165. doi: 10.1016/j.vetmic.2005.06.014
- Mase, M., Imada, T., Sanada, Y., Etoh, M., Sanada, N., Tsukamoto, K., Kawaoka, Y. & Yamaguchi, S. (2001). Imported parakeets harbor H9N2 influenza A viruses that are genetically closely related to those transmitted to humans in Hong Kong. Journal of Virology, 75, 3490–3494. doi: 10.1128/JVI.75.7.3490-3494.2001
- Meulemans, G., Boschmans, M., Deccaesstecker, M. & van den Berg, T. (1999). Isolation of a H5N2 non-pathogenic avian influenza virus in Belgium in 1999. In Proceedings of the joint sixth annual meetings of the national newcastle disease and avian influenza laboratories of countries of the European Union. Brussels.
- Pantin-Jackwood, M.J., Stephens, C.B., Bertran, K., Swayne, D.E., Spackman, E. & Davis, C.T. (2017). The pathogenesis of H7N8 low and highly pathogenic avian influenza viruses from the United States 2016 outbreak in chickens, turkeys and mallards. PLoS One, 12, e0177265. doi: 10.1371/journal.pone.0177265
- Pantin-Jackwood, M.J. & Swayne, D.E. (2009). Pathogenesis and pathobiology of avian influenza virus infection in birds. Revue Scientifique et Technique, 28, 113–136. doi: 10.20506/rst.28.1.1869
- Perkins, L.E. & Swayne, D.E. (2003). Varied pathogenicity of a Hong Kong-origin H5N1 avian influenza virus in four passerine species and budgerigars. Veterinary Pathology, 40, 14–24. doi: 10.1354/vp.40-1-14
- Post, J., Burt, D.W., Cornelissen, J.B., Broks, V., van Zoelen, D., Peeters, B. & Rebel, J.M.J. (2012). Systemic virus distribution and host responses in brain and intestine of chickens infected with low pathogenic or high pathogenic avian influenza virus. Virology Journal, 9, 61. doi: 10.1186/1743-422X-9-61
- Post, J., de Geus, E.D., Vervelde, L., Cornelissen, J.B. & Rebel, J.M. (2013). Systemic distribution of different low pathogenic avian influenza (LPAI) viruses in chicken. Virology Journal, 10, 23. doi: 10.1186/1743-422X-10-23
- Senne, D.A., Pearson, J.E., Miller, L.D. & Gustafson, G.A. (1983). Virus isolations from pet birds submitted for importation into the United States. Avian Diseases, 27, 731–744. doi: 10.2307/1590316
- Ssematimba, A., Hagenaars, T.J., de Wit, J.J., Ruiterkamp, F., Fabri, T.H., Stegeman, J.A. & de Jong, M.C.M. (2013). Avian influenza transmission risks: analysis of biosecurity measures and contact structure in Dutch poultry farming. Preventive Veterinary Medicine, 109, 106–115. doi: 10.1016/j.prevetmed.2012.09.001
- Steensels, M., Van Borm, S., Lambrecht, B., De Vriese, J., Le Gros, F.X., Bublot, M. & van den Berg, T. (2007). Efficacy of an inactivated and a fowlpox-vectored vaccine in Muscovy ducks against an Asian H5N1 highly pathogenic avian influenza viral challenge. Avian Diseases, 51, 325–331. doi: 10.1637/7628-042806R.1
- Van Borm, S., Steensels, M., Ferreira, H.L., Boschmans, M., De Vriese, J., Lambrecht, B. & van den Berg, T. (2007). A universal avian endogenous real-time reverse transcriptase-polymerase chain reaction control and its application to avian influenza diagnosis and quantification. Avian Diseases, 51, 213–220. doi: 10.1637/7552-033106R.1
- Van Borm, S., Vangeluwe, D., Steensels, M., Poncin, O., van den Berg, T. & Lambrecht, B. (2011). Genetic characterization of low pathogenic H5N1 and co-circulating avian influenza viruses in wild mallards (Anas platyrhynchos) in Belgium, 2008. Avian Pathology, 40, 613–628. doi: 10.1080/03079457.2011.621410
- Webster, R.G., Bean, W.J., Gorman, O.T., Chambers, T.M. & Kawaoka, Y. (1992). Evolution and ecology of influenza A viruses. Microbiological Reviews, 56, 152–179.
- Yamamoto, H., Otsuki, K. & Tsubokura, M. (1979). Isolation of influenza A virus from budgerigars and its serological characterization. Microbiology and Immunology, 23, 637–642. doi: 10.1111/j.1348-0421.1979.tb00504.x
- Yuan, J., Xu, L., Bao, L., Yao, Y., Deng, W., Li, F., Lv, Q., Gu, S., Wei, Q. & Qin, C. (2015). Characterization of an H9N2 avian influenza virus from a Fringilla montifringilla brambling in northern China. Virology, 476, 289–297. doi: 10.1016/j.virol.2014.12.021