ABSTRACT
Pathogenic strains of Enterococcus cecorum cause symmetrical paralysis in broilers due to infection of the free thoracic vertebra. The disease caused by pathogenic E. cecorum, known as enterococcal spondylitis or “kinky-back” continues to be responsible for significant losses to the broiler industry worldwide. In outbreaks of pathogenic E. cecorum, gut colonization and sepsis occur in the first three weeks-of-life. Since maternal antibodies are present during this period, we postulated that vaccination of breeders with a polyvalent killed vaccine would protect chicks from challenge. To test this hypothesis, representative isolates from seven genotype groups of pathogenic E. cecorum circulating in the US were chosen to produce adjuvanted killed vaccines (bacterins) and given to broiler-breeder hens. No single strain produced high titres of antibodies to all other strains; however, the combination of serologic reactivity of pathogenic isolates (designated SA3 and SA7) was sufficient to react with all genotypes. Vaccination of commercial broiler-breeder hens with a bacterin composed of SA3 and SA7 did not have any adverse effects. Vaccinated hens developed E. cecorum specific antibodies; however, no significant difference in survival was observed in infected embryos from hens in vaccine or adjuvant only groups. Chicks from vaccinated hens also failed to resist homologous or heterologous challenge during experimental infection. In a macrophage killing assay, pathogenic E. cecorum were found to evade opsinophagocytosis with elicited antibodies. These data suggest that pathogenic strains of E. cecorum possess virulence mechanisms that confound antibody-mediated opsinophagocytosis, complicating vaccine development for this pathogen of broilers.
Introduction
Pathogenic strains of Enterococcus cecorum cause outbreaks of spinal infection and paralysis in broiler production systems around the world (Devriese et al., Citation2002; Wood et al., Citation2002; Herdt et al., Citation2009; Stalker et al., Citation2010; Makrai et al., Citation2011; Robbins et al., Citation2012; Szeleszczuk et al., Citation2013; Aitchison et al., Citation2014; Albini et al., Citation2014; Jung & Rautenschlein, Citation2014; Talebi et al., Citation2016; Borst et al., Citation2017; Jung et al., Citation2017). The disease caused by pathogenic E. cecorum is called enterococcal spondylitis (ES) or “kinky-back” because infection of the free thoracic vertebra is responsible for clinical signs. Molecular epidemiologic studies and whole genome sequencing revealed that E. cecorum strains isolated from spinal lesions have distinct genotypes and genomic composition compared to their commensal counterparts (Borst et al., Citation2012; Borst et al., Citation2015). Despite much work on the epidemiology and pathogenesis of this disease, effective treatments for disease caused by pathogenic E. cecorum are lacking. Antimicrobial therapy is not effective once birds show clinical signs, and no vaccine for this organism is available.
In recent work detailing the pathogenesis of E. cecorum infection, we noted that virulent strains of E. cecorum colonize the gut and translocate into the bloodstream in the first three weeks-of-life (Borst et al., Citation2017). This is in contrast to commensal E. cecorum, which did not appear in the intestine until week 3 and did not escape the gut niche (Borst et al., Citation2017). As pathogenic E. cecorum enter the circulation during in the first three weeks-of-life, we postulated that passive transfer of maternal antibodies, which are present in this period, could protect chicks from bacteraemia and spinal lesions. In this work, we evaluated the utility of a polyvalent killed vaccine in breeder hens to prevent bacteraemia and disease caused by pathogenic E. cecorum in their chicks. We also assessed the function of elicited antibodies in opsinophagocytosis of pathogenic E. cecorum.
Materials and methods
Strain selection
Through a national surveillance programme funded by the US Department of Agriculture, we have accumulated a collection of over 3000 isolates of pathogenic E. cecorum recovered from outbreaks of ES in different regions of the US for genotyping using pulsed field gel electrophoresis. For this study, seven strains (SA1-7), representing the common genotypes observed circulating in US production systems, were selected for development of a polyvalent killed vaccine. In addition to these virulent strains, non-pathogenic, commensal E. cecorum strain CE1 was selected for use as a control for some studies. Strains SA1-7 were evaluated for virulence in an embryo lethality assay in a prior study (Borst et al., Citation2014) and whole genome seqeunce is available for strains SA1-3 and CE1 (Borst et al., Citation2015).
Bacterin
Each of the seven pathogenic E. cecorum strains was revived from frozen (–80°C) stock on trypticase soy agar containing 5% sheep blood (BA). Plates were incubated at 37°C overnight with 5% CO2 and evaluated for purity. For each strain, a 250 ml flask containing 30 ml of Todd-Hewitt broth with 0.125% dextrose was inoculated from the BA plates. Cultures were grown standing for approximately 4 h at 42°C with 5% CO2 until an OD600 of 0.9–1.0 was reached. Bacteria were killed by adding 0.35% of 37% formaldehyde and gently mixing for 6 h at room temperature. To ensure the culture was completely killed, a 1 ml sample from each flask was collected, centrifuged and plated onto BA. Plates were incubated for 48 h at 37°C with 5% CO2 and assessed for growth. Oil-emulsion adjuvant (Emulsigen-D, MVP Technologies, Omaha, NE, USA) was added at a final concentration of 20% v/v.
Bird experiments
Experimental use of birds was approved by the Animal Care and Use Committee and conducted in compliance with the Guidelines for Care and Use of Laboratory Animals at North Carolina State University.
Eliciting antibodies in breeder hens
Antibodies were raised in 13-week-old breeder hens by vaccinating groups of seven birds with one of the seven selected bacterins or with media/adjuvant only. For vaccination, 0.5 ml adjuvanted bacterin was injected intramuscularly in the breast muscle. Serum was collected by venipuncture of the brachial vein at three time points: immediately prior to vaccination; at booster (four weeks later); and four weeks following booster (eight weeks post initial vaccination). Elicited antibodies were detected using a whole cell enzyme-linked immunosorbent assay which was performed as previously described (Borst et al., Citation2011). Briefly, individual plates were coated with one of seven strains. Serum from birds, n = 7 per bacterin group, were evaluated individually against all seven bacterial strains at each time point in triplicate. For each assay, the absorbance of all wells was standardized by subtracting the negative (no bacteria) control from all wells. Average absorbance (OD450) was compared among strains to identify serologic cross-reactivity (). SA3 exhibited robust antigenicity producing cross-reactive antibodies to most strains; however, strains SA4 and SA7 had numerically decreased cross-reactivity and overall antigenicity compared to other strains. Strain SA7 provided the best response to SA4 and SA7; therefore, SA7 was included with SA3 in a polyvalent vaccine to ensure cross-reactivity to all seven representative strains.
Vaccination of commercial broiler-breeders
A polyvalent bacterin containing strains SA3 and SA7 was created as described above, but in sufficient quantities to vaccinate 10,000 Ross 708 hens. A commercial broiler-breeder flock, housed in two separate houses in North Carolina (NC), was vaccinated with either polyvalent killed vaccine (house 1) or media/adjuvant only sham vaccination (house 2). Vaccination and booster occurred at weeks 11 and 18 according to the standard vaccination schedule of the integrator. Vaccine was delivered intramuscularly in the breast muscle alternating between the right and left sides for first vaccine dose and booster. Birds in both houses were grown under standard conditions, and no health challenges, including pathogenic E. cecorum infection, were reported during the growing period. Serum was collected from 30 birds from both the vaccinated (Vx) and sham (Sham) groups at 11 weeks-of-age, prior to initial vaccination, and again at week 32 as birds entered peak egg production. Elicited antibodies were assessed using whole cell enzyme-linked immunosorbent assay (as above) with SA3 used to coat the plates. Sera from five birds were pooled and the resulting six samples were run in triplicate. Mean absorbances were compared between Vx and Sham birds at both time points using a Student’s t-test of significance. Fertile eggs were also collected from birds at 32 weeks for use in the embryo lethality and broiler challenge experiments.
Opsonophagocytosis assay
Isolation of peritoneal macrophages was performed as previously described (Qureshi et al., Citation1994). Broiler chickens (n = 60) were obtained from a commercial hatchery in NC on the day of hatch. Birds were raised in a BSL-2 isolation room in pens with 20 birds per pen. At 14 to 17 days-of-age, birds were injected with 1 ml/100 g body weight of 3% Sephadex G-50 solution in phosphate buffered saline (PBS). Forty hours post injection, birds were euthanized and the peritoneal cavity was flushed with 30–40 ml of cold heparinized saline (0.5 U/ml, saline 0.75%) to collect elicited macrophages. Twenty microlitres of the harvested fluid was cultured overnight on BA at 37°C with 5% CO2 to identify microbial contaminants. Fifty microlitres of the harvested fluid was cytocentrifuged onto a microscopic slide and stained with Diff-Quik to perform a 100 cell differential count to establish the purity of sample. The remainder of the harvested fluid was centrifuged at 250 g for 5 min, resuspended in 2 ml of foetal bovine serum with 10% DMSO, and stored in cryovials at −80°C for 24 h. After 24 h, samples were transferred to liquid nitrogen storage (–140°C).
For opsonophagocytosis assays, cryovials of macrophages were rapidly warmed in a 37°C water bath, resuspended in LM Hahn medium and incubated in T75 flasks at 42°C and 5% CO2 for 4 h (Qureshi et al., Citation1990). After 4 h, the medium was replaced with fresh, pre-warmed LM Hahn medium. After 24 h, cell monolayers were rinsed 2× with PBS and then incubated in 3 ml of 0.05% Trypsin-EDTA solution. After 2 min of incubation, 10 ml of LM Hahn medium was added. The cell suspension was transferred to a 50 ml polypropylene conical tube and centrifuged at 250 g for 5 min. The supernatant was decanted and the cell pellet was resuspended in 5 ml of antibiotic-free LM Hahn medium. A 50 µl aliquot was mixed 1:1 with Trypan Blue solution, incubated for 1 min, and then cell number and viability were determined using a haemocytometer. The remaining cell suspension was diluted to 4 × 105 cells/ml and cells were plated in 24-well plates with 2 × 105 cells/well. Twenty hours after plating in 24-well plates, 50% of the LM Hahn medium was removed and replaced with fresh medium.
E. cecorum strains CE1, SA3, and SA4 were grown for 16 h in Todd-Hewitt Broth with 0.125% dextrose at 37°C and 5% CO2. The bacteria were then sub-cultured in fresh medium, grown to log phase at 42°C, and opsonized in 5% pooled serum from either sham or vaccinated birds (five birds per pool) in PBS for 30 min rotating end-over-end. All serum used for opsonization was previously incubated at 56°C for 1 h to heat inactivate complement components.
Opsonized bacteria (strains CE1, SA3, and SA4) were inoculated onto macrophage monolayers at 100 MOI per well, in triplicate. After 1 h of incubation, cell monolayers were rinsed 2× with PBS and incubated in LM Hahn with 100 µg/ml gentamicin sulphate and 2.5 µg/ml penicillin G for 1 h to kill extracellular bacteria. Monolayers were then rinsed 2× with PBS, lysed using 0.1% Triton X solution, and dilutions of the surviving intracellular bacteria were plated on BA for quantification of phagocytosed bacteria.
Opsonophagocytosis assays were performed with three separate pools of sham and vaccinated sera and were run in triplicate for a total of three biologic and three technical repeats. Average percent phagocytosis was compared among groups using analysis of variance with Tukey HSD post hoc pairwise comparisons (JMP-13, SAS Raleigh, NC). Cut-off for significance was set at P < 0.05.
Embryo lethality assay
An embryo lethality assay has been developed to measure the virulence of E. cecorum strains (Borst et al., Citation2014). Fertile eggs collected from Vx and Sham groups during peak egg production (32 weeks) were incubated for 12 days. Embryos were challenged with strains SA3 and SA7 (homologous challenge), SA1 (heterologous challenge) and commensal E. cecorum strain CE1. Embryos were incubated for 96 h and candled every 24 h to assess viability. Results were plotted as Kaplan–Meier survival curves and statistically analysed by log rank test of significance using Statistica (TIBCO Software, Palo Alto, CA USA). Cut-off for significance was set at P < 0.05.
Broiler challenge
Broiler chicks from both Vx and Sham groups (n = 288 per group) were individually tagged and randomly placed in three separate isolation rooms: two rooms for bacterial challenge and one infection control room. The two isolation rooms for bacterial challenge had six pens with 20 broiler chicks from vaccinated hens (n = 120 Vx group) and 6 pens with 20 chicks from hens receiving sham vaccine (n = 120 Sham group). Oral challenge of day-old broilers was performed as previously described (Borst et al., Citation2017). Birds in the first isolation room were challenged with strain SA3 (homologous challenge) and birds in the second isolation room were challenged with strain SA4 (heterologous challenge). The third isolation room housed infection control birds that received PBS. This room contained 48 chicks in the Vx group and 48 chicks in the Sham group housed in 3 pens of 16 birds each.
All birds were grown under standard conditions for 35 days and sampled as follows. Serum was collected from birds for serology at days 0, 14, and 35. For day 0, blood was collected via jugular venipuncture from eight birds per pen and pooled by pen (three pooled samples from Vx birds and three pooled samples from Sham birds). At day 14, blood samples from eight birds from five Vx pens and four Sham pens in the SA3 and SA4 challenge rooms and from three Vx and three Sham pens in the infection control room were collected via brachial vein venipuncture and pooled by pen for analysis. Finally, at necropsy on day 35, blood was collected from all birds and pooled by pen. Spleens were sterilely collected at necropsy and cultured for E. cecorum as previously described (Borst et al., Citation2017). The spinal column surrounding the free thoracic vertebra was collected into formalin for histologic evaluation of ES lesions.
Serologic responses were compared for each time point using the Student’s t-test of significance or analysis of variance with post hoc Tukey HSD pairwise of tests of significance as appropriate (JMP-13, SAS Raleigh, NC). Within each challenge, the proportion of positive spleen culture results and proportion of histologic lesions of ES were compared using the Fisher’s Exact test of significance (Statistica™, TIBCO Software, Palo Alto, CA USA). Cut-off for significance was set at P < 0.05.
Results
Eliciting antibodies in breeder hens
Vaccination and booster of broiler-breeder hens with all seven bacterins elicited antibodies compared to sham vaccination with media/adjuvant only (). The seven representative strains exhibited variable antigenicity with strains SA3 and SA7 producing the most antibodies to all tested strains. These two strains were incorporated into a polyvalent vaccine for testing in a commercial farm.
Vaccination of commercial broiler-breeders
A commercial broiler-breeder flock, housed in two separate houses in NC, was vaccinated with either polyvalent killed vaccine (house 1) or adjuvant-only sham vaccination (house 2). Vaccination and booster occurred at weeks 11 and 18 according to the standard vaccination schedule of the integrator with no adverse reactions reported. Pre-vaccination, low levels of antibodies to pathogenic E. cecorum strain SA3 were detected and likely represent cross-reactivity with cellular antigens shared with commensal E. cecorum strains; however, low-level infection of the breeder flock with pathogenic E. cecorum cannot be ruled out (). No significant differences between Vx or Sham groups were detected pre-vaccination; however, at week 32, the mean post-booster serologic response was significantly higher in Vx birds compared to the Sham group (P = 0.121) (). Interestingly, elevated serologic response to E. cecorum was detected in the Sham group suggesting that a non-specific increase in E. cecorum reactive antibodies occurs over the growing period.
Opsonophagocytosis assay
To compare the functionality of antibodies elicited by the polyvalent killed or sham vaccine, an in vitro antibody-mediated opsonophagocytosis assay was performed (). Pooled sera from 32 week-old Vx and Sham hens were incubated with pathogenic E. cecorum strains SA3 (homologous challenge), SA4 (heterologous challenge) or the commensal strain CE1. Serum from both Vx and Sham inoculated hens significantly increased opsonophagocytosis of the commensal E. cecorum strain CE1 compared to no-serum (PBS) control (P = 0.0013 and P = 0.0028, respectively). However, no increased opsonophagocytosis of pathogenic E. cecorum strains SA3 and SA4 was observed with either Vx or Sham group sera. These data indicate that pathogenic strains of E. cecorum may have intrinsic factors that allow for evasion of opsonophagocytosis.
Figure 3. Compared to no-serum (PBS) control, significantly increased antibody-mediated opsonophagocytosis (a) was observed when commensal strain CE1 was incubated with serum from hens in either Vx (P = 0.0013) or Sham (P = 0.0028) groups. However, no significant differences were observed in opsonophagocytosis when pathogenic strains SA3 or SA4 were incubated with serum from hens in either Vx or Sham groups.
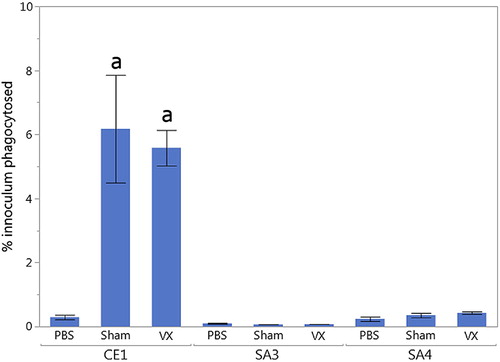
Embryo lethality assay
To further assess the function of antibodies elicited by vaccination, fertile eggs from Vx and Sham group hens were collected for use in an embryo lethality assay. For this assay, eggs from both groups were inoculated with the commensal E. cecorum strain CE1; pathogenic E. cecorum strains SA3 or SA7 (homologous challenge), or pathogenic E. cecorum strain SA1 (heterologous challenge). Survival curves for each challenge isolate are presented in . No significant difference in survival was observed for embryos inoculated with any of the pathogenic E. cecorum strains. However, there was significantly increased survival of embryos from vaccinated birds inoculated with commensal E. cecorum strain CE1 (P = 0.0024).
Broiler challenge
Broiler chicks from Vx and Sham hens were hatched in the integrator’s commercial hatchery and transferred to isolation rooms for oral inoculation with pathogenic strain SA3 (homologous challenge) or SA4 (heterologous challenge). Chicks from both Vx and Sham groups were also raised without challenge (PBS only control). Enzyme-linked immunosorbent assays for antibodies to E. cecorum were performed at days 0, 14, and 35 (weeks 0, 2, and 5) for control and challenge groups (). In uninfected control birds on the day of hatch, maternal transfer of anti-E. cecorum antibodies was observed with significantly increased antibodies detected in chicks from Vx hens compared to chicks from Sham hens (P < 0.001).
Figure 5. Passive transfer of maternal anti-E. cecorum antibodies was detected at week 0 as evidenced by significantly increased serologic response (a) in Vx (right side bars) compared to Sham (left bars) chicks (P < 0.001). However, these antibodies were no longer detectable at week 2 in non-challenged control birds. Broilers from Vx hens developed antibodies to SA3 challenge (a) more quickly than broilers from Sham group hens as evidenced by significantly higher serologic response compared to all other groups (P < 0.001). At week 5, no significant differences were observed between serologic response of broilers from Sham or Vx group hens; however, the trend of increased serologic response in SA3 challenged birds (a, P < 0.005) compared to SA4 challenged birds (b) continued. Both challenged groups had significantly higher antibody response compared to unchallenged control birds (c, P < 0.001).
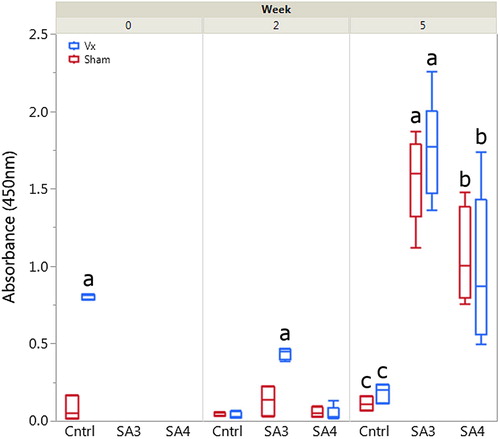
These antibodies were short-lived, with anti-E. cecorum antibodies from non-infected control birds found to be significantly decreased compared to day 0 and no longer significantly different in birds from Sham hens at day 14. At day 14, significantly increased anti-E. cecorum antibodies were detected in SA3 challenged birds from Vx birds (P < 0.001); however, no significant increase was observed in birds receiving the SA4 heterologous challenge. At day 35, no significant differences were observed between Vx and Sham group birds in any challenge group. However, birds challenged with SA3, regardless of vaccine status, had significantly increased antibodies detected compared to SA4 challenged birds or control birds (P < 0.005 for all pairwise comparisons). This finding recapitulates the increased antigenicity of SA3 compared to SA4 observed in . As expected, both SA3 and SA4 challenged birds had significantly greater serologic response than control birds (P < 0.001 for all pairwise comparisons).
Challenged broilers were assessed at day 35 for disease manifestations of E. cecorum infection. Bacteraemia was measured by culturing the spleens of all birds, and the presence of the characteristic spinal lesion of E. cecorum was measured using histopathology of the free thoracic vertebra of all birds. While a general trend was observed towards decreased bacteraemia and decreased histologic evidence of spinal infection in birds from Vx hens, no statistically significant differences were observed ().
Table 1. Spleen culture and free thoracic vertebral histopathology.
Discussion
Few options exist for the treatment or control of pathogenic E. cecorum. Once clinical signs of enterococcal spondylitis occur, antimicrobial therapy is not effective. Antimicrobial therapy (e.g. penicillin) in the first two weeks-of-life has been used anecdotally to prevent disease; however, controlled studies to determine the effectiveness of this therapy have not been performed. As there is no guarantee subsequent flocks will break with pathogenic E. cecorum, preventative therapy may not be needed or desired, especially in production systems that limit exposure to antibiotics. As such, vaccination would be a preferred method for control of this emerging pathogen.
Vaccines made with whole killed bacteria applied to hens are routinely deployed to prevent disease in chicks due to passive transfer of immunoglobulins (IgA and IgY) in the egg. These vaccines have the advantage of being safe and relatively inexpensive to produce: however, killed vaccines have some drawbacks. They are somewhat labour-intensive for delivery as birds must be individually vaccinated, typically via subcutaneous or intramuscular injection, to elicit an immune response and occasionally vaccine-site inflammatory lesions develop. Likewise, protection from heterologous challenge can be limited using killed vaccines as pathogenic bacteria from different serogroups or with variable surface antigens can avoid detection by antibodies developed to the vaccine strain (Glisson, Citation1998; Ghunaim et al., Citation2014).
In this work, we developed and assessed a killed polyvalent vaccine that elicited antibodies to seven known genotypes of pathogenic E. cecorum. The inclusion of two strains SA3 and SA7 was required to provide serologic cross-reactivity to all strains. Antibodies produced by vaccinated hens were shown to transfer to chicks and were present in blood in the early life period. However, these antibodies were ineffective in preventing disease caused by pathogenic E. cecorum.
An underlying mechanism for vaccine failure was investigated. Our data indicate that pathogenic E. cecorum strains were able to evade opsonophagocytosis while a commensal E. cecorum strain could not. Interestingly, a consistent feature of pathogenic E. cecorum genomes is the presence of a novel polysaccharide capsule locus that has homology to the antiphagocytic capsule of Streptococcus pneumoniae (Borst et al., Citation2015). Although additional studies are needed to determine if this capsule locus does play a role in virulence, it may be a target for the future development of a modified live vaccine. A modified live vaccine for pathogenic E. cecorum would be highly desirable as live vaccines can be distributed in the water, spray-administered at hatch, or, if sufficiently attenuated, given in ovo. Currently, the major roadblock to the production of rationally designed modified live vaccine is the fact that pathogenic E. cecorum remains genetically intractable to date, preventing the creation of targeted gene deletion mutants.
In conclusion, vaccination of breeder hens with a polyvalent killed vaccine for pathogenic E. cecorum was not effective in preventing disease in chicks. Vaccine failure was likely due to evasion of humoral immunity through specific virulence determinants carried by pathogenic E. cecorum. As such, the outlook for killed vaccines as an efficient and effective therapeutic intervention for pathogenic E. cecorum is questionable.
Disclosure statement
No potential conflict of interest was reported by the authors.
ORCID
Luke B. Borst http://orcid.org/0000-0002-2736-1960
Additional information
Funding
References
- Aitchison, H., Poolman, P., Coetzer, M., Griffiths, C., Jacobs, J., Meyer, M. & Bisschop, S. (2014). Enterococcal-related vertebral osteoarthritis in South African broiler breeders: a case report. Journal of the South African Veterinary Association, 85, 1077. doi: 10.4102/jsava.v85i1.1077
- Albini, S., Faye, I., Lobsiger, C., Stadler-Thommen, I., Renggli, F. & Hoop, R.K. (2014). Infektionen mit dem kapnophilen Bakterium Enterococcus cecorum bei Mastbroilern. Schweizer Archiv für Tierheilkunde, 156, 295–298. doi: 10.1024/0036-7281/a000594
- Borst, L.B., Patterson, S.K., Lanka, S., Barger, A.M., Fredrickson, R.L. & Maddox, C.W. (2011). Evaluation of a commercially available modified-live Streptococcus equi subsp equi vaccine in ponies. American Journal of Veterinary Research, 72, 1130–1138. doi: 10.2460/ajvr.72.8.1130
- Borst, L.B., Suyemoto, M.M., Keelara, S., Dunningan, S.E., Guy, J.S. & Barnes, H.J. (2014). A chicken embryo lethality assay for pathogenic Enterococcus cecorum. Avian Diseases, 58, 244–248. doi: 10.1637/10687-101113-Reg.1
- Borst, L.B., Suyemoto, M.M., Robbins, K.M., Lyman, R.L., Martin, M.P. & Barnes, H.J. (2012). Molecular epidemiology of Enterococcus cecorum isolates recovered from Enterococcal spondylitis outbreaks in the southeastern United States. Avian Pathology, 41, 479–485. doi: 10.1080/03079457.2012.718070
- Borst, L.B., Suyemoto, M.M., Sarsour, A.H., Harris, M.C., Martin, M.P., Strickland, J.D., Oviedo, E.O. & Barnes, H.J. (2017). Pathogenesis of Enterococcal spondylitis caused by Enterococcus cecorum in broiler chickens. Veterinary Pathology, 54, 61–73. doi: 10.1177/0300985816658098
- Borst, L.B., Suyemoto, M.M., Scholl, E.H., Fuller, F.J. & Barnes, H.J. (2015). Comparative genomic analysis identifies divergent genomic features of pathogenic Enterococcus cecorum including a type IC CRISPR-CAS system, a capsule locus, an epa-like locus, and putative host tissue binding proteins. PLoS One, 10, e0121294. doi: 10.1371/journal.pone.0121294
- Devriese, L.A., Cauwerts, K., Hermans, K. & Wood, A.M. (2002). Enterococcus cecorum septicemia as a cause of bone and joint lesions resulting in lameness in broiler chickens. Vlaams Diergeneeskundig Tijdschrift, 71, 219–221.
- Ghunaim, H., Abu-Madi, M.A. & Kariyawasam, S. (2014). Advances in vaccination against avian pathogenic Escherichia coli respiratory disease: potentials and limitations. Veterinary Microbiology, 172, 13–22. doi: 10.1016/j.vetmic.2014.04.019
- Glisson, J.R. (1998). Bacterial respiratory disease of poultry. Poultry Science, 77, 1139–1142. doi: 10.1093/ps/77.8.1139
- Herdt, P.d., Defoort, P., Steelant, J.v., Swam, H., Tanghe, L., Goethem, S.v. & Vanrobaeys, M. (2009). Enterococcus cecorum osteomyelitis and arthritis in broiler chickens. Vlaams Diergeneeskundig Tijdschrift, 78, 44–48.
- Jung, A., Metzner, M. & Ryll, M. (2017). Comparison of pathogenic and non-pathogenic Enterococcus cecorum strains from different animal species. BMC Microbiology, 17, 33. doi: 10.1186/s12866-017-0949-y
- Jung, A. & Rautenschlein, S. (2014). Comprehensive report of an Enterococcus cecorum infection in a broiler flock in Northern Germany. BMC Veterinary Research, 10, 311. doi: 10.1186/s12917-014-0311-7
- Makrai, L., Nemes, C., Simon, A., Ivanics, E., Dudas, Z., Fodor, L. & Glavits, R. (2011). Association of Enterococcus cecorum with vertebral osteomyelitis and spondylolisthesis in broiler parent chicks. Acta Veterinaria Hungarica, 59, 11–21. doi: 10.1556/AVet.59.2011.1.2
- Qureshi, M.A., Marsh, J.A., Dietert, R.R., Sung, Y.J., Nicolas-Bolnet, C. & Petitte, J.N. (1994). Profiles of chicken macrophage effector functions. Poultry Science, 73, 1027–1034. doi: 10.3382/ps.0731027
- Qureshi, M.A., Miller, L., Lillehoj, H.S. & Ficken, M.D. (1990). Establishment and characterization of a chicken mononuclear cell line. Veterinary Immunology and Immunopathology, 26, 237–250. doi: 10.1016/0165-2427(90)90094-9
- Robbins, K.M., Suyemoto, M.M., Lyman, R.L., Martin, M.P., Barnes, H.J. & Borst, L.B. (2012). An outbreak and source investigation of Enterococcal spondylitis in broilers caused by Enterococcus cecorum. Avian Diseases, 56, 768–773. doi: 10.1637/10253-052412-Case.1
- Stalker, M.J., Brash, M.L., Weisz, A., Ouckama, R.M. & Slavic, D. (2010). Arthritis and osteomyelitis associated with Enterococcus cecorum infection in broiler and broiler breeder chickens in Ontario, Canada. Journal of Veterinary Diagnostic Investigation, 22, 643–645. doi: 10.1177/104063871002200426
- Szeleszczuk, P., Dolka, B., Żbikowski, A., Dolka, I. & Peryga, M. (2013). First case of Enterococcal spondylitis in broiler chickens in Poland. Medycyna Weterynaryjna, 69, 298–303.
- Talebi, A., Taifebagherlu, J., Sharifi, A. & Delkhosh-Kasmaie, F. (2016). Spondylitis in broiler breeder farms in west-Azerbaijan province, Iran: clinical report. Veterinary Research Forum, 7, 353–355.
- Wood, A.M., MacKenzie, G., McGiliveray, N.C., Brown, L., Devriese, L.A. & Baele, M. (2002). Isolation of Enterococcus cecorum from bone lesions in broiler chickens. The Veterinary Record, 150, 27.