ABSTRACT
A double recombinant strain of herpes virus of turkeys (HVT) was constructed that contains the fusion (F) gene from Newcastle disease virus (NDV) and the gD plus gI genes from infectious laryngotracheitis virus (ILTV) inserted into a non-essential region of the HVT genome. Expression of the F protein was controlled by a human cytomegalovirus promoter, whereas expression of gD plus gI was driven by an ILTV promoter. The double recombinant vaccine virus (HVT-NDV-ILT) was fully stable genetically and phenotypically following extended passage in cell culture and infection of chickens. Safety of the vaccine virus was confirmed by overdose and backpassage studies in specific-pathogen-free chickens. Chickens vaccinated with a single dose of HVT-NDV-ILT administered by the in ovo route were highly protected from challenge with the velogenic NDV (GB Texas), ILTV (LT 96-3) and Marek’s disease virus (GA 5) strains (97%, 94% and 97%, respectively). Similarly, chickens vaccinated with a single dose by subcutaneous (SC) route at 1 day of age were highly protected from challenge with the same three viruses (100%, 100%, and 88%, respectively). The protection level of a single dose given by in ovo or SC route against challenge with a virulent Marek’s disease virus strain demonstrates that insertion of multiple genes from two different pathogens within the HVT genome had no adverse effect on the capacity of HVT to protect against Marek’s disease. These results demonstrate that HVT-NDV-ILT is a safe and efficacious vaccine for simultaneous control of NDV, ILTV and Marek’s diseases.
Introduction
Immunization of chickens with live, inactivated or recombinant vaccines is extensively used in the poultry industry world-wide and vaccination is considered the cornerstone of infectious disease control in all segments of poultry production (Witter & Hunt, Citation1994; Sharma, Citation1999). With the increasing availability of information on the protective antigens and immune responses against many poultry pathogens (Martinez-Torrecuadrada et al., Citation2003; Kumar et al., Citation2011; Muller et al., Citation2012; Pradhan, et al., Citation2012; Coppo et al., Citation2013a; Kapczynski et al., Citation2013; Kim et al., Citation2013), it has become possible to apply recombinant DNA technology to the development of novel vaccines that can be easily administered through mass application, display improved safety and efficacy profiles and are less costly to produce (Boyle & Coupar, Citation1988; Sondermeijer et al., Citation1993). Currently, there are several recombinant vaccines (Reddy et al., Citation1996; Le Gros et al., Citation2009; Johnson et al., Citation2010; Esaki et al., Citation2013; Kapczynski et al., Citation2015) based on the herpes virus of turkeys (HVT) that are approved for protection against diseases caused by four important avian pathogens (infectious laryngotracheitis virus, ILTV; Newcastle disease virus, NDV; infectious bursal disease virus, IBDV; and avian influenza virus). The global commercial application of these recombinant vaccines demonstrates their important role in protecting poultry flocks and in overcoming problems associated with use of live vaccines such as vaccine reactions in the respiratory tract and potential reversion to virulence (Rodriguez-Avila et al., Citation2007; Bwala et al., Citation2011; Palya et al., Citation2012; Coppo et al., Citation2013b).
The diseases caused by ILTV and NDV result in severe economic losses in the poultry industry due to high mortality, morbidity and/or reduced egg production (Ou & Giambrone, Citation2012; Ashraf & Shah, Citation2014). Concurrent infections with ILTV and NDV can occur early in life, therefore the application of HVT-based recombinant vaccines at the same time in the hatchery is ideal. While first generation recombinant HVT-based vaccines such as HVT-ND and HVT-ILT (Morgan et al., Citation1992; Vagnozzi et al., Citation2012) have proven their utility in the control of diseases and production losses caused by the NDV and ILTV viruses, previous attempts to combine the recombinant HVT-ND and HVT-ILT vaccines for dual protection under field situations indicated that they can interfere with each other (our unpublished data). In addition, a recent study (Slacum et al., Citation2009) showed that interference occurs when recombinant HVT vaccines are simultaneously administrated with a conventional HVT vaccine, and this interference is expressed as a decrease in the immunity against the virus from which the donor genes in the recombinant HVT vaccine are derived. Slacum et al. (Citation2009) did not investigate the mechanism for the interference observed in this study. Since the vaccines cannot be administered together, a choice is made between the HVT recombinants used under commercial rearing situations contingent on which disease problems are being encountered in the local region. Therefore, in order to provide simultaneous protection against NDV and ILTV from one viral vector, and maintain protection against Marek’s disease virus (MDV) from an HVT virus backbone, we constructed a double recombinant HVT-NDV-ILT vaccine strain that concurrently expresses the F protein from NDV and the gD plus gI proteins from ILTV.
The present report describes the characterization of this vaccine in terms of its in vivo stability, tropism and safety profile. The efficacy of this vaccine is demonstrated by the results showing protection of susceptible chickens against challenge with virulent NDV, ILTV and MDV. In addition, to expand the range of protection to other important pathogens, the double recombinant vaccine virus was also tested for compatibility with live attenuated IBDV and MDV vaccines.
Materials and methods
Double recombinant vaccine virus
A double recombinant HVT vector vaccine, designated HVT-NDV-ILT, which contains both the ILTV gD plus gI genes and the NDV-F gene, was constructed using the strategy shown in . First, an insertion plasmid was constructed. This plasmid contains a DNA fragment from the ILTV short region corresponding to the gD plus gI ILTV expression cassette, and a DNA fragment corresponding to the NDV fusion (F) gene expression cassette. The gD plus gI and F expression cassettes are inserted into the HVT US2 region. Chicken embryo fibroblasts (CEF) cells were transfected with a set of overlapping cosmid/plasmid-derived DNA fragments from the HVT vaccine strain FC126, plus the insertion plasmid, and the cells allowed to recombine the fragments at the overlap regions. The viral stock obtained following transfection of CEF was plaque purified on CEF cells to assure purity.
Fertile eggs and chickens
Specific-pathogen-free (SPF) white leghorn chickens used in the studies described in this report were obtained from Charles River SPAFAS, Storrs, Connecticut, or from Valo Biomedica GmbH, Germany. Chickens were housed in biosecurity level 2 isolators or in isolation rooms with floor pens. Food and water were provided ad libitum. All experimental procedures were performed in compliance with institutional animal use guidelines.
Vaccination
Eggs with viable embryos, at 18 days of embryonation, were inoculated by the in ovo route with 0.1 ml of the respective vaccine or diluent placebo. The inoculum was administered into the amniotic sac using a 1 ml syringe fitted with a 22G × 1¼″ needle. One-day-old chicks were vaccinated by the subcutaneous route with 0.2 ml in the back of the neck using a 22G × 1″ needle to administer the respective vaccine or diluent placebo.
Vaccine titration
The double recombinant vaccine was titrated in six-well plates on confluent monolayers of CEF cells. Serial dilutions of the vaccine sample were prepared and plates inoculated. Plates were incubated at 38.0 ± 1°C with 4–5% CO2 for 5 days with a 3 ml maintenance medium re-feed on day 3. On day 5, the medium was decanted and the CEF monolayers were fixed with 100% methanol (2 ml per well) for 5–10 min at room temperature. Two wells were stained at each dilution using specific antibodies against HVT gB, ILTV gD, ILTV gI or NDV F proteins. Plates were observed under a fluorescent microscope and titres were calculated by multiplying the average number of plaques by the dilution factor. Titres are reported as plaque forming units (PFU) per ml. Titration of the IBDV vaccine was conducted using CEF cells. Briefly, serial dilutions of the vaccine were prepared in CEF (7 × 105 cells per ml) and 200 μl of each dilution dispensed per well (10 wells per dilution) in 96-well plates. The vaccine titration plates were incubated at 38.0 ± 1°C with 4–5% CO2 and read for cytopathic effects (CPE) on day 6 post-titration. Vaccine titre was calculated by the Reed and Muench method (Reed and Muench, Citation1938) using LISA 1.60 Intervet Statistical Application Software, Boxmeer, the Netherlands.
Challenge procedures and viruses
All challenge viruses were titrated in chickens to calculate the virus dilution that causes disease in at least 80% (MDV GA 5 and RB1B strains and USDA strain ILTV 96-3) or at least 90% (NDV Texas GB strain, classical IBDV STC strain and Variant E IBDV strain) of non-vaccinated chickens. ILTV challenge was conducted using the USDA challenge strain by the intratracheal route using 104.5 50% egg infective dose (EID50) in 0.25 ml. NDV challenge was conducted using the velogenic Texas GB strain by the intramuscular (IM) route with 104 embryo lethal dose (ELD50) in 0.2 ml. MDV challenge was carried out using the GA 5 strain by the intraperitoneal (IP) route (0.2 ml/bird of stock virus diluted 1:8 in Marek’s vaccine diluent) or with the RB1B strain by the intramuscular route into the leg (0.2 ml/bird of stock virus diluted 1:200). IBDV challenge was conducted by the intraocular route with classical IBDV STC strain using 102.5 EID50 per dose or with variant E IBDV strain using102.2 EID50 per dose. Titration of the variant E IBDV was conducted by inoculating ten-fold dilutions of the virus into embryonated chicken eggs and scoring daily for 7 days. Positive egg embryos exhibit haemorrhage, mottled necrosis of the liver and/or kidneys and death. HVT FC126 strain is a commercially available HVT vaccine (Merial Select, Inc., Duluth, GA).
Tissue and sample collection
Six types of samples were collected and processed from each treatment group at each time point and plated onto confluent CEF monolayers for virus isolation. These samples included peripheral blood leukocytes, spleen, bursa, liver, tracheal washings and feather follicle epithelium (FFE). For peripheral blood leukocytes, and at each collection time point, samples from birds in the same treatment group were pooled (one pool per group). Leukocytes from each pooled sample were isolated using the SepMate™-50 density gradient centrifugation procedure. Briefly, each blood sample, diluted with an equal volume of PBS containing 2% FBS, was added to the SepMate™ 50 tube, Cambridge, MA. Following centrifugation, at 1200×g for 10 min at room temperature (15–25°C), the cell pellet was resuspended to a final concentration of 1.6 × 107 cells/ml in EMEM maintenance medium. For spleen, bursa and lung tissues at each time point, samples from the same treatment group were collected and pooled (one pool/group) in EMEM maintenance medium. Pooled organs and the collection medium were poured through a 70 µm cell strainer and placed in a 50 ml conical tube. The tissue was pressed through the strainer with the plunger of a 3 cc syringe to release the cells. A cell count was performed, and the cell suspension was adjusted to 1.6 × 107 cells/ml using EMEM maintenance medium. For FFE, 5–10 g of FFE samples were collected by pulling feathers from the breast or wing of each bird and cutting the proximal 3–5 mm of the feather shaft into a tube containing 25 ml of EMEM maintenance medium with antibiotics and antimycotics. The collected feather follicles were sonicated for 2 min (4 bursts of 30 s each) with an ultrasonic oscillator with the needle probe set at an intensity of 70. The suspension was centrifuged at 1000 rpm for 15 min using a Beckman GS-6R centrifuge. The supernatant was filtered through a 0.45 µm filter. No cell count was performed. Tracheal washing samples from each treatment group were pooled by group (one pool/group). No cell count was performed.
Virus isolation
Cells from processed tissues were adjusted to a standard cell density (1.6 × 107/ml) and serial dilutions were prepared. No cell count was conducted for processed FFE and tracheal washings, instead a standard undiluted volume (0.1 ml per well of a 96-well dish) was used. At least two aliquots of each sample were added to separate wells on confluent monolayers of CEF. Medium only was used to inoculate wells used for negative control. Plates were incubated for 3 days in a 38.5 ± 1°C, 4.0–6.0% CO2 incubator then the maintenance medium was replaced and plates incubated for an additional 2 days.
Immunofluorescence antibody assay
After 5 days incubation, the medium was decanted and the CEF monolayer was fixed with 100% methanol for 5–10 min at room temperature. Methanol was decanted and monolayers allowed to air dry followed by staining with one of four primary antibodies: polyclonal Rabbit anti-ILT-gI sera (B04-120-01), monoclonal Mouse anti-ILT-gD (Mab #6), Mouse anti-NDV-F (Mab #57), or Mouse anti-HVT-gB (Mab #L78.2). The antibody was diluted and added at 2.0 ml per well then incubated at room temperature for 3 h on a rocking platform. After antibody incubation, plates were washed three times with 1X PBS. Fluorescein isothiocyanate-conjugated secondary antibodies, anti-Rabbit IgG – FITC (Sigma, St. Louis, MO, catalog no. F9887, diluted 1:50) and anti-Mouse IgG – FITC (Sigma, catalog no. F9137, diluted 1:100) were prepared and 2 ml was added to each well. Plates were incubated for 1 h at room temperature. Following incubation, plates were washed three times with 1X PBS. Following staining, HVT plaques were counted under bright light and under fluorescent light. Virus titre was determined using plaque counts obtained from fluorescent light.
DNA extraction
Frozen tissue samples were thawed and 5 × 106 cells were pelleted at 12,000 rpm for 3 min. DNA was extracted and purified using a KingFisher Cell and Tissue DNA Kit (Thermo Scientific, Vernon Hills, IL, catalog no. 97030196). Briefly, pelleted cells were resuspended in 200 µl of lysis buffer, then 25 µl of Proteinase K solution was added to each sample, and lysed at 56°C overnight. Lysed samples were centrifuged for 5 min at 600×g to remove debris and supernatant was collected for DNA purification. DNA was purified from each sample on a 96-well Thermo Scientific KingFisher Flex machine according to the manufacturer’s instructions. DNA was extracted from 1 ml tracheal wash or FFE wash samples using a KingFisher Viral NA Extraction Kit (Thermo Scientific, catalog no. 97040196) after concentrating fluids to a final volume of 200 μl, using a MicrosepTM 100 K Omega Micro concentrator (Pall Life Sciences, Port Washington, NY, Catalog no. OD100C46).
Primers and polymerase chain reaction (PCR)
The primers used for this study are listed in . Two primer sets were designed to anneal to the two junctions of the inserted cassettes within the recombinant virus and amplify a 542 bp product and a 1886 bp product. Two additional primer sets were designed to anneal within the native HVT gB gene or the native HVT US2 gene. A 904 bp product should be amplified from any HVT virus carrying the native gB gene (including the double recombinant), and a 187 bp product from wild-type HVT (lacking an insert in the US2 region). PCR assays were set up using HotStarTaq Master Mix (Qiagen Germantown, MD, Catalog No. 203445), which contained HotStarTaq DNA Polymerase, PCR buffer (with 3 mM MgCl2), and 400 μM each dNTP. Fifty microliter reactions contained 2 μl of the purified DNA, 1× Enzyme Mix and 1 μM of the forward and reverse primers. To improve sensitivity, reactions using the US2/gD primer set were run in a final volume of 100 μl, and contained 5 μl DNA. The thermal cycler conditions consisted of: one cycle of 95°C for 14–15 min; 35–37 cycles of 94°C for 30 s (denaturing), 60°C for 30 s (annealing), 72°C for 1–2 min (extension); one cycle of 72°C for 6–7 min. A volume of 10 μl of each reaction was electrophoresed on either a 1.2% or a 2% agarose E-gel to visualize the product.
Table 1. List of primers used for PCR.
Experimental design
Overdose safety studies in chickens
The safety of the double recombinant vaccine virus was evaluated following vaccination by in ovo and subcutaneous (SC) routes using a vaccine dose representing at least 10 times as much viable virus as could be contained in one dose of vaccine at the time of serial release. At 18-days of embryonation, 75 eggs were vaccinated with 266,400 PFU of HVT-NDV-ILT master seed virus (MSV) per dose in 0.1 ml and 75 eggs were inoculated with 0.1 ml of a Marek’s diluent as placebo. Fifty one-day-old chicks were vaccinated with 336,000 PFU of HVT-NDV-ILT MSV per dose in 0.2 ml by the subcutaneous route. At one day of age, chicks were housed on floors in a BSL2 facility. At 5 days of age, 49 non-vaccinated chicks were challenged with the very virulent Marek’s disease virus RB1B to determine if the birds used in the study were susceptible to Marek’s disease. The chickens challenged with RB1B virus were monitored for 7 weeks and any surviving chickens were necropsied at 7 weeks of age and observed for gross lesions indicative of Marek’s disease. Chickens in the other groups were kept and monitored for adverse events or clinical signs of MDV, NDV, or ILTV. At 120 days of age these chickens were necropsied and evaluated for any observable lesions.
Backpassage studies
Twenty-five one-day-old leghorn chicks hatched from SPF eggs were inoculated via the IP route with 32,000 PFU of the HVT-NDV-ILT vaccine. Seven days post inoculation, spleens were harvested and pooled and then cells were dissociated and counted. Processed spleen cells were then inoculated IP into a second group of day-old chicks using 1.6 × 107 cells/bird. Seven days post-inoculation the process was repeated and harvested spleen cells from the second group were used to inoculate a third group of day-old chicks. This process was repeated two more times, for a total of five backpassages through chicks. After the fifth backpassage, chickens were observed for clinical signs of MDV, NDV, and ILTV for 7 weeks. After 7 weeks, these birds were necropsied and evaluated for any observable lesions. Also, at the fifth passage, spleen cells were harvested from a group of birds and plated on CEF monolayers to isolate virus for further analysis.
Dissemination in chickens
The tropism of the double recombinant vaccine was evaluated and compared to that of parental FC 126 HVT strain in SPF chickens. Two groups of 35 one-day-old chickens per group were vaccinated subcutaneously with either the double recombinant vaccine HVT-NDV-ILT (5100 PFU/bird) or a commercially available vaccine HVT FC126 (2497 PFU/bird). Peripheral blood leukocytes, spleen, bursa, lung, trachea and FFE samples were collected from the chickens in each group at weekly intervals through five weeks of age. The samples were processed and used to prepare viral DNA for PCR and for virus isolations in CEF.
Efficacy against ND
Protection against challenge with NDV was evaluated in accordance with standard procedures defined by Title 9, Code of Federal Regulations using the velogenic NDV strain Texas GB. Challenge with NDV was carried out 4 weeks after vaccination. For assessment of protection, two groups of eggs at 18 days of embryonation were inoculated in ovo with 1825 or 2730 PFU/dose of the double recombinant vaccine (groups 1 and 2, respectively) and one group of eggs was inoculated with a diluent placebo as a challenge control. In addition, two groups of day-old chicks were inoculated SC with 2326 or 1641 PFU/dose of the vaccine (groups 1 and 2, respectively), and one group was inoculated also SC with a diluent placebo and served as a challenge control. Protection from challenge was evaluated over a 14-day period and birds were scored positive if any of the typical clinical signs of ND were observed. Typical signs of NDV infection include lack of coordination, paralysis, and/or death. An unprotected bird represents a bird demonstrating any of the clinical signs of ND on any day during the post-challenge observation period.
Efficacy against ILT
Protection against challenge with ILTV was evaluated in accordance with standard procedures defined by 9 CFR using the USDA ILTV challenge strain LT-96-3. For assessment of protection, three groups of eggs at 18 days of embryonation were inoculated in ovo with 1409, 1408, or 1738 PFU/dose of the double recombinant vaccine (groups 1–3; respectively) and one group of eggs was inoculated with a diluent placebo as a challenge control. In addition, two groups of day-old chicks were inoculated SC with 2009 or 2376 PFU/dose of the vaccine (groups 1 and 2; respectively) and one group inoculated SC with a diluent placebo which served as a challenge control. Birds were challenged at 28 days of age and protection was evaluated over a 10-day-period following challenge. Birds were observed for typical clinical signs of ILT such as ocular involvement (swelling, discharge and/or redness), expectoration of blood, gasping, laboured breathing and/or death. A chicken was scored positive for ILT clinical signs if mortality, expectoration of blood, more than one day of gasping or acute laboured breathing, or ocular involvement were observed.
Efficacy against MDV GA 5 strain
Challenge with MDV was carried out 5 days after vaccination using virulent MDV GA 5 strain as per 9CFR requirements. For assessment of protection, two groups of eggs at 18 days of embryonation were inoculated in ovo with 1825 or 2730 PFU/dose of the double recombinant vaccine (groups 1 and 2, respectively) and one group of eggs was inoculated with a diluent placebo as a challenge control. In addition, one group of day-old chicks were inoculated SC with 2230 PFU/dose of the double recombinant vaccine and one group inoculated SC with a diluent placebo which served as a challenge control. Protection was evaluated over a 49-day-period following challenge and chickens were observed for clinical signs such as lack of coordination, paralysis, depression, ruffled feathers and/or death, or gross lesions of Marek’s disease such as gross enlargement of peripheral nerves and visceral tumors involving the liver, heart, spleen, gonad, kidney proventriculus and other organs and tissues. An unprotected bird will demonstrate clinical signs or grossly observable lesions of Marek’s Disease.
Efficacy against MDV RB1B strain following vaccination with HVT-NDV-ILT plus serotype 1, strain CVI-988 (Rismavac®)
Challenge with MDV was carried out 5 days after vaccination using the very virulent RB1B MDV strain. For assessment of protection, one group of day-old chicks was inoculated SC with one dose (1000 PFU) of serotype 1, strain CVI-988 mixed in the same diluent together with 2000 PFU/dose of the HVT-NDV-ILT vaccine. A second group of day-old chicks was inoculated SC with only the Marek’s vaccine diluent and served as the challenge control. Protection was evaluated over a 70-day period following challenge and chickens were observed for clinical signs such as lack of coordination, paralysis, depression, ruffled feathers and/or death, or gross lesions of Marek’s disease such as gross enlargement of peripheral nerves and visceral tumours involving the liver, heart, spleen, gonad, kidney, proventriculus and other organs and tissues. In addition, birds that died or were culled during the lytic phase of the infection (approximately 10–21 days after infection) were necropsied and the thymus and bursa examined for signs of depletion which occurs during this phase of the infection.
Efficacy against classical and variant IBDV following vaccination with HVT-NDV-ILT plus live 89/03 IBDV vaccine
Challenge with classical IBDV was carried out 4 weeks after vaccination using USDA IBDV STC challenge strain. For assessment of protection, two groups of eggs at 18 days of embryonation were inoculated in ovo with two different doses of live 89/03 IBDV vaccine (3.2 and 3.3 log10 TCID50 per dose) combined in the same ampoule with 8341 or 8892 PFU/dose of the recombinant HVT-NDV-ILT vaccine formulation (groups 1 and 2, respectively), and one group of eggs was inoculated with only the HVT-NDV-ILT vaccine formulation (1875 PFU/dose) and served as a challenge control. In addition, two groups of day-old chicks were inoculated SC with two different doses of live 89/03 IBDV (3.2 and 3.4 log10 TCID50 per dose) in combination with 8428 or 8871 PFU/dose of the HVT-NDV-ILT vaccine (groups 1 and 2, respectively), and one group was inoculated also SC with only the HVT-NDV-ILT (2714 PFU/dose) vaccine and served as the challenge control. Four days following challenge, birds were necropsied and examined for typical gross lesions of IBDV which include peri-bursal oedema and/or oedema and haemorrhage in the bursal tissue.
Challenge with variant IBDV was carried out four weeks after vaccination using variant E challenge strain. For assessment of protection, a group of eggs at 18 days of embryonation was inoculated in ovo with live 89/03 IBDV vaccine (4.29 log10 TCID50 per dose) combined in the same ampoule with 19,300 PFU/dose of the recombinant HVT-NDV-ILT vaccine, and one group of eggs was inoculated with only the double recombinant vaccine (9385 PFU/dose) and served as a challenge control. In addition, two groups of day-old chicks were inoculated SC with live 89/03 IBDV (3.72 or 3.94 log10 TCID50 per dose) in combination with 7933 or 8833 PFU/dose of the HVT-NDV-ILT vaccine (groups 1 and 2, respectively) and one group inoculated also SC with only the HVT-NDV-ILT (2650 PFU/dose) vaccine which served as the challenge control. Ten days following challenge, birds were necropsied and protection evaluated based on the calculation of bursa/body weight ratio.
Results
Vaccine construction and verification
The assembly of the double recombinant vaccine virus (as shown in ) as well as verification of the sequences of inserted F and gD plus gI genes were confirmed following sequencing of the DNA of the inserted genes, which were obtained following PCR amplification from the double recombinant viral DNA (data not shown). In addition, strong expression of the proteins encoded by inserted genes was confirmed by immunofluorescence ().
Figure 2. Photographs of HVT-NDV-ILT plaques stained with antibodies specific to the three inserted gene products. (A) HVT-NDV-ILT plaque stained with anti-ILT gD monoclonal antibody, MAB 6, followed by a FITC labelled goat anti-mouse IgG. (B) HVT-NDV-ILT plaque stained with a rabbit anti-ILT gI polyclonal antibody followed by a FITC labelled goat anti-rabbit IgG. (C) HVT-NDV-ILT plaque stained with an anti-NDV-F monoclonal antibody, 57NDV, followed by a FITC labelled goat anti-mouse IgG.
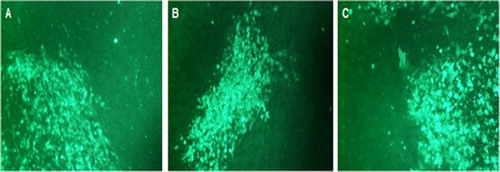
Overdose safety
The safety of the double recombinant vaccine in terms of its effects on hatchability and potential to cause disease or changes in body weights in susceptible chickens was evaluated using SPF birds injected in ovo or SC with more than 10 times as much viable virus as will be contained in one dose of vaccine. The hatchability of eggs vaccinated with the HVT-NDV-ILT was 87% while the hatchability of eggs inoculated with a Marek’s vaccine diluent was 83% (). Based on these results, vaccination with the recombinant vaccine had no adverse effect on hatchability. All birds in the HVT-NDV-ILT vaccinated groups and the Marek’s vaccine diluent vaccinated group were free of clinical signs of Marek’s disease and free of Marek’s disease lesions throughout the study. Eighty-eight per cent of birds challenged with very virulent MDV RB1B strain showed clinical signs and/or lesions typical of Marek’s disease confirming that the birds used in this study were susceptible to MDV. At the time of necropsy (120 days of age), there was no statistically significant difference in the mean bird body weight between the vaccinated groups and the Marek’s vaccine diluent vaccinated group (). These results demonstrate that the HVT-NDV-ILT vaccine is safe for use in chickens.
Figure 3. The safety of the double recombinant vaccine HVT-NDV-ILT, in terms of its potential to cause changes in body weights in chickens, was evaluated in an overdose study using SPF birds as described under materials and methods. At the time of necropsy (120 days of age), the mean body weight for each of the treatment groups was computed with the GLM procedure in SAS® 9.1.3. There was no statistically significant difference in the mean bird body weight between the vaccinated groups and the Marek’s vaccine diluent inoculated group. Left hand bars for each treatment group represent males, right females.
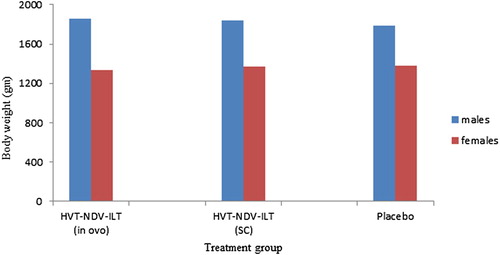
Table 2. Hatchability and Marek’s disease lesions following administration of an overdose of HVT-NDV-ILT vaccine or challenge of birds with RB1B MDV strain.
Backpassage and in vivo stability
In vivo stability and safety of the double recombinant virus was further evaluated by backpassage studies. For this purpose, 25 one-day-old chicks were each vaccinated with 32,000 PFU virus by the intraperitoneal (IP) route. The viral plaques recovered from the final backpassage (BP5) and plaques obtained from virus stock used for initial inoculation were analysed by IFA. All plaques isolated at P0 (initial virus stock) and at BP5 demonstrated strong expression of the proteins encoded by the inserted genes. These results confirm the stability of viral protein expression following in vivo passage. In addition, a group of 20 birds was monitored for 7 weeks after the fifth backpassage. No adverse reactions or clinical signs associated with MDV, NDV, or ILTV were observed and these birds were also free of any Marek’s disease or other lesions upon necropsy. These results confirm that the insertion of multiple genes from two different pathogens did not alter the safety profile of HVT backbone vector.
Dissemination of HVT-NDV-ILT in chickens
The tropism of the recombinant vaccine was evaluated and compared to that of parental FC126 HVT strain in SPF chickens. Various tissues and organs were collected after vaccination with HVT-NDV-ILT or HVT FC126. Tissue samples were processed and inoculated on CEF monolayers for virus detection by IFA using specific antibodies to HVT gB protein, or used for DNA extraction followed by PCR amplification and detection of bands corresponding to gD of ILTV or gB of HVT (data not shown). As shown in , the tissue distribution of HVT-NDV-ILT is not different from HVT FC126 as shown by PCR and, to a lesser extent, IFA. These results confirm that the insertion of multiple genes from two other viruses did not change the tropism of the double recombinant HVT-NDV-ILT vaccine virus.
Table 3. Dissemination of HVT-NDV-ILT vaccine virus in chickens.
Evaluation of protective immunity to NDV
The efficacy of the double recombinant vaccine in protection against challenge with the velogenic NDV strain Texas GB was evaluated by calculation of the per cent of animals with clinical signs of NDV infection. As indicated in , all non-vaccinated birds died following NDV challenge. On the other hand, 95% and 97% of birds vaccinated in ovo had no signs of NDV disease. In addition, 97% and 100% of birds vaccinated by SC were also free from clinical signs of NDV. These results demonstrate that the HVT-NDV-ILT vaccine described in this study is highly efficacious in protection against NDV.
Table 4. Protection against NDV.
Evaluation of protective immunity to ILTV
The efficacy of the double recombinant vaccine in protection against challenge with a virulent ILTV strain (USDA standard challenge strain) was evaluated in SPF chickens which were vaccinated by the in ovo or SC routes. Two groups of chickens were inoculated either in ovo or SC with a diluent and served as the respective challenge control. In the groups vaccinated by the in ovo route, 85%, 86%, and 94% of chickens had no signs of ILTV disease (). By contrast, 93% of chicken vaccinated in ovo with a placebo diluent displayed typical signs of ILTV disease such as expectoration of blood, nasal exudate, conjunctivitis and ocular swelling/discharge. In the two groups vaccinated by the SC route, 100% of chickens had no clinical signs of ILTV disease, whereas 80% of chickens vaccinated SC with a placebo diluent displayed typical signs of ILTV disease (). These results show that the double recombinant HVT-NDV-ILT vaccine is highly efficacious against challenge with a virulent ILTV.
Table 5. Protection against ILTV.
Evaluation of protective immunity to MDV GA5 and RB1B strains
The protection afforded by the HVT-NDV-ILT vaccine was assessed following challenge with a virulent MDV (GA 5). For assessment of protection, two groups of eggs at 18 days of embryonation were inoculated in ovo with two different dose formulations of the recombinant vaccine and one group of eggs was inoculated with a diluent placebo as a challenge control. In the groups vaccinated with HVT-NDV-ILT, 80% and 97% of vaccinated birds had no signs of Marek’s disease, whereas 89% of placebo-vaccinated birds showed clinical signs and lesions typical of Marek’s disease (). Protection was also assessed when birds were vaccinated by the SC route. One group of day-old chicks was inoculated SC with the recombinant vaccine and one group was also inoculated SC with a diluent placebo and served as a challenge control. In the vaccinated group, 88% of birds were free of clinical signs or lesions typical of Marek’s disease, whereas 97% of placebo-vaccinated birds had clinical signs and lesions typical of Marek’s disease (). These results demonstrate that the insertion of multiple genes from two different pathogens did not alter the ability of the HVT backbone to provide solid protection against challenge with a virulent MDV.
Table 6. Protection against MDV.
In addition, protection against challenge with the very virulent RB1B virus isolate was evaluated in birds vaccinated with HVT-NDV-ILT vaccine plus serotype 1 strain CVI-988. One group of day-old chicks were inoculated SC with the HVT-NDV-ILT vaccine plus serotype 1 strain CVI-988 mixed in the same diluent, and one group inoculated also SC with the diluent alone and served as the challenge control. In the vaccinated group, 98% of vaccinated birds were free of clinical signs or lesions typical of Marek’s disease, whereas 80% of placebo-vaccinated birds had clinical signs or lesions typical of Marek’s disease (). These results show that the addition of the double recombinant vaccine virus to serotype 1 strain CVI-988 had no adverse effect on protection against a very virulent MDV.
Evaluation of protective immunity to classical and variant IBDV
In order to determine if concurrent vaccination with the double recombinant vaccine and a live IBDV vaccine interferes with the protection against IBDV, groups of chickens were vaccinated with the live IBDV 89/03 vaccine strain mixed in the same ampoules with an HVT-NDV-ILT vaccine formulation. For assessment of protection, two groups of eggs at 18 days of embryonation were inoculated in ovo with two different doses of IBDV 89/03 strain plus the HVT-NDV-ILT vaccine. One group of eggs was inoculated with a diluent placebo (HVT-NDV-ILT vaccine only) as a challenge control. In the groups vaccinated with 89/03 IBDV plus HVT-NDV-ILT vaccines, 97% and 100% of birds were protected from challenge with classical STC IBDV strain. On the other hand, 97% of placebo-vaccinated birds showed lesions typical of IBDV such as bursal oedema and haemorrhage (). In addition, protection against STC challenge was also assessed when birds were vaccinated by SC route. For this purpose, two groups of one-day-old chicks were inoculated SC with two different dose formulations of HVT-NDV-ILT vaccine + IBDV 89/03 strain. An additional group of one-day-old chicks was inoculated SC with a placebo (HVT-NDV-ILT vaccine only) as a challenge control. In the groups vaccinated with 89/03 IBDV plus HVT-NDV-ILT vaccine, 97% and 100% of birds were protected from challenge with classical STC IBDV strain. On the other hand, 90% of placebo-vaccinated birds showed bursal lesions typical of IBDV (). For assessment of protection against variant IBDV, groups of chickens were vaccinated, either in ovo or SC, with the live IBDV 89/03 vaccine doses indicated in mixed in the same ampoules with an HVT-NDV-ILT vaccine formulation. Protection against variant E challenge was evaluated by determination of bursal/body weight ratio in birds necropsied 10 days following challenge with a variant E IBDV strain. As shown in , 94% of birds vaccinated by the in ovo route were protected against challenge with variant E IBDV, whereas 100% of birds inoculated in ovo with a diluent placebo were positive for IBDV disease. On the other hand, 97% and 100% of birds vaccinated SC were protected whereas 96% of birds inoculated SC with a diluent placebo were positive for IBDV disease.
Table 7. Protection against classical IBDV.
Table 8. Protection against variant IBDV.
Discussion
Vaccination is considered the most effective strategy for control of many infectious agents causing severe economic losses in poultry flocks worldwide (Sharma, Citation1999; Marangon & Busani, Citation2006). However, a large number of live vaccines are required to be given at a young age to chickens sometimes causing difficulties with compatibility, vaccine reactions and potentially reversion to virulence (Guy et al., Citation1991; Gelb et al., Citation2007; Landman et al., Citation2017). The development of recombinant HVT vaccines has significantly improved some of these aspects thus improving the welfare of chickens and user convenience. Several recombinant HVT-based vaccines have been developed and used successfully to protect poultry flocks against a number of important avian pathogens (Reddy et al., Citation1996; Le Gros et al., Citation2009; Johnson et al., Citation2010; Esaki et al., Citation2013; Kapczynski et al., Citation2015). However, to date, while some of these vaccines have apparently been shown to be compatible (Paniago et al., Citation2016), our own extensive experience (unpublished) and published work (Slacum et al., Citation2009; Godoy et al., Citation2011) have demonstrated that there is significant interference when these vaccines are given simultaneously, affecting the efficacy of one or both vaccines in an unpredictable manner. Until now, the development of recombinant vaccines that can protect against multiple diseases from one vector with a single dose delivered by the mass application has proven difficult (Iqbal, Citation2012) especially in the case of HVT. To overcome the above described shortcomings, we constructed a double recombinant HVT-vectored vaccine (HVT-NDV-ILT) and assessed its safety and potential as a polyvalent vaccine for protection against three key avian pathogens; MDV, NDV and ILTV.
The safety of the double recombinant vaccine described here was confirmed by overdose and backpassage studies. In the overdose study, SPF embryos at 18 days of age or one-day-old chicks were vaccinated in ovo or SC, respectively, with a dose that contains more than 10 times as much viable virus as will be contained in a single dose of the vaccine. These birds did not show any adverse reactions or MDV lesions. In addition, vaccination with this overdose did not affect hatchability. Moreover, at the end of the study, there was no statistically significant difference between the body weights of HVT-NDV-ILT vaccinated birds and those vaccinated with a Marek’s vaccine diluent as a placebo. In the backpassage study, HVT-NDV-ILT virus did not cause disease or become virulent after five backpassages in chicken indicating that insertion of foreign genes did not alter the safety profile of HVT.
The tropism of the HVT-NDV-ILT vaccine was evaluated and compared to that of parental FC126 HVT strain in groups of SPF chickens separately vaccinated SC with the respective vaccine. PCR analysis of samples from multiple organs and tissue types collected over 5 weeks after vaccination shows that the distribution of the recombinant virus within the host is similar to that of the parental HVT FC126 strain. The immunofluorescence results likely showed lower distribution based on the sensitivity of this test compared to PCR. Overall, these results confirm that the recombinant virus was not altered in its tropism as a result of the insertion of multiple genes from two different pathogens.
The efficacy of the recombinant HVT-NDV-ILT as a polyvalent vaccine was assessed in vaccination/challenge studies as prescribed in 9CFR. For all three pathogens (ILTV, NDV and MDV), the double recombinant vaccine provided excellent protection after a single dose given by the in ovo or SC routes. These results indicate that the insertion of several foreign genes did not adversely affect the capacity of HVT to protect against Marek’s disease. In addition, experiments in which HVT-NDV-ILT was combined with live IBDV 89/03 vaccine strain demonstrate solid protection against IBDV STC challenge when SPF embryos or one-day-old chicks were vaccinated by in ovo or SC routes, respectively. Moreover, the capacity of this vaccine to provide solid protection against MDV challenge was not negatively affected when combined with other Marek’s disease vaccines.
In summary, the present report ushers in a new generation of safe and well-characterized HVT-based recombinant vaccines that provide excellent protection against three key poultry pathogens from a single HVT backbone with a single dose given by mass application. We suggest that this will help the poultry industry with their vaccination processes and improve bird welfare.
Acknowledgment
The authors thank the Animal Care Staff at Elkhorn, Nebraska and Millsboro, Delaware facilities for their excellent support in the conduct of these studies. In addition the authors thank Sara Darby for skillful assistance with preparation of this manuscript.
Disclosure statement
The authors of this study are employees of Intervet Inc. USA and Intervet UK Ltd.
References
- Ashraf, A. & Shah, M.S. (2014). Newcastle disease: present status and future challenges for developing countries. African Journal of Microbiology Research, 8, 411–416. doi: 10.5897/AJMR2013.6540
- Boyle, D.B. & Coupar, B.E. (1988). Construction of recombinant fowlpox viruses as vectors for poultry vaccines. Virus Research, 10, 343–356. doi: 10.1016/0168-1702(88)90075-5
- Bwala, D.G., Fasina, F.O., Van Wyk, A. & Duncan, N.M. (2011). Effect of vaccination with lentogenic vaccine and challenge with virulent Newcastle disease virus (NDV) on egg production in commercial and SPF chickens. International Journal of Poultry Science, 10, 98–105. doi: 10.3923/ijps.2011.98.105
- Coppo, M.J.C., Hartley, C.A. & Devlin, J.M. (2013a). Immune responses to infectious laryngotracheitis virus. Developmental and Comparative Immunology, 41, 454–462. doi: 10.1016/j.dci.2013.03.022
- Coppo, M.J.C., Noormohammadi, A.H., Browning, G.F. & Devlin, J.M. (2013b). Challenges and recent advancements in infectious laryngotracheitis vaccines. Avian Pathology, 42, 195–205. doi: 10.1080/03079457.2013.800634
- Esaki, M., Noland, L., Eddins, T., Godboy, A., Saeki, S., Saitoh, S., Yasuda, A. & Dorsey, K.M. (2013). Safety and efficacy of a turkey herpes virus vector laryngotracheitis vaccine for chickens. Avian Diseases, 57, 192–198. doi: 10.1637/10383-092412-Reg.1
- Gelb, Jr. J., Ladman, B.S., Lacata, M.J., Shapiro, M.H. & Campion, L.R. (2007). Evaluating viral interference between infectious bronchitis virus and Newcastle disease virus vaccine strains using quantitative reverse transcription-polymerase chain reaction. Avian Diseases, 51, 924–934. doi: 10.1637/7930-020807-REGR.1
- Godoy, A., Esaki, M., Flegg, P., Rosenberger, J.K., Rosenberger, S., Dorsey, K.M. & Gardin, Y. (2011). Compatibility of vectored LT vaccines and Vectormune HVT NDV. Proceedings of the annual AAAP meeting, Saint Louis, July 16–19, (p. 23).
- Guy, J.S., Barnes, H.J. & Smith, L.G. (1991). Increased virulence of modified-live infectious laryngotracheitis vaccine virus following bird-to-bird passage. Avian Diseases, 35, 348–355. doi: 10.2307/1591188
- Iqbal, M. (2012). Progress toward the development of polyvalent vaccination strategies against multiple viral infections in chickens using herpes virus of turkeys as vector. Bioengineered, 3, 222–226. doi: 10.4161/bioe.20476
- Johnson, D.I., Vagnozzi, A., Dorea, F., Riblet, S.M., Mundt, A., Zavala, G. & Garcia, M. (2010). Protection against infectious larynogotracheitis by in ovo vaccination with commercially available viral vector recombinant vaccines. Avian Diseases, 54, 1251–1259. doi: 10.1637/9401-052310-Reg.1
- Kapczynski, D.R., Alfonso, C.L. & Miller, P.J. (2013). Immune responses of poultry to Newcastle disease virus. Developmental and Comparative Immunology, 41, 447–453. doi: 10.1016/j.dci.2013.04.012
- Kapczynski, D.R., Esaki, M., Dorsey, K.M., Jiang, H., Jackwood, M., Moraes, M. & Gardin, Y. (2015). Vaccine protection of chickens against antigenically diverse H5 highly pathogenic avian influenza isolates with a live HVT vector vaccine expressing the influenza hemagglutinin gene derived from a clade 2.2 avian influenza. Vaccine, 33, 1197–1205. doi: 10.1016/j.vaccine.2014.12.028
- Kim, S.-H., Wanasen, N., Paldurai, A., Xiao, S., Collins, P.L. & Samal, S.K. (2013). Newcastle disease virus fusion protein is the major contributor to protective immunity of genotype-matched vaccines. PLoS ONE, 8, e74022. doi: 10.1371/journal.pone.0074022
- Kumar, S., Nayak, B., Collins, P.L. & Samal, S.K. (2011). Evaluation of the Newcastle disease virus F and HN proteins in protective immunity by using a recombinant avian paramyxovirus type 3 vector in chickens. Journal of Virology, 85, 6521–6534. doi: 10.1128/JVI.00367-11
- Landman, W.J.M., Vervaet, C., Remon, J.P., Huyge, K. & van Eck, J.H.H. (2017). Primary Newcastle disease vaccination of broilers: comparison of the antibody seroresponse and adverse vaccinal reaction after eye–nose drop or coarse spray application, and implication of the results for a previously developed coarse dry powder vaccine. Avian Pathology, 46, 451–461. doi: 10.1080/03079457.2017.1307941
- Le Gros, F.X., Dancer, A., Giacomini, C., Pizzoni, L., Bublot, M., Graziani, M. & Prandini, F. (2009). Field efficacy trial of a novel HVT-IBD vector vaccine for 1-day-old broilers. Vaccine, 27, 592–596. doi: 10.1016/j.vaccine.2008.10.094
- Marangon, S. & Busani, L. (2006). The use of vaccination in poultry production. Scientific and Technical Review of the Office International des Epizooties (Paris), 26, 265–274. doi: 10.20506/rst.26.1.1742
- Martinez-Torrecuadrada, J.L., Saubi, N., Pages-Mante, A., Caston, J.R. & Casal, J.I. (2003). Structure-dependent efficacy of infectious bursal disease virus (IBDV) recombinant vaccines. Vaccine, 21, 3342–3350. doi: 10.1016/S0264-410X(02)00804-6
- Morgan, R.W., Gelb, J.C., Schreurs, S., Lutticken, D., Rosenberger, J.K. & Sondermeijer, J.P. (1992). Protection of chickens from Newcastle and Marek's diseases with a recombinant Herpes virus of turkeys vaccine expressing the Newcastle disease virus fusion protein. Avian Diseases, 36, 858–872. doi: 10.2307/1591544
- Muller, H., Mundt, E., Eterraadossi, N. & & Islam, R. (2012). Current status of vaccines against infectious bursal disease. Avian Pathology, 41, 133–139. doi: 10.1080/03079457.2012.661403
- Ou, S.C. & Giambrone, J.J. (2012). Infectious laryngotracheitis virus in chickens. World Journal of Virology, 1, 142–149. doi: 10.5501/wjv.v1.i5.142
- Palya, V., Kiss, I., Tatar-Kis, T., Mato, T., Felfoldi, B. & Yardin, Y. (2012). Advancement in vaccination against Newcastle disease: recombinant HVT NDV provides high clinical protection and reduces challenge virus shedding with the absence of vaccine reactions. Avian Diseases, 56, 282–287. doi: 10.1637/9935-091511-Reg.1
- Paniago, M., Gardin, Y., Palya, V., Cazaban C. & Lozano, F. (2016). Assessment of the compatibility between the vector RHVT-F and RHVT-H5 vaccines in SPF and commercial chickens. 65th Western Poultry Disease Conference (pp.195–196). Vancouver, BC, Canada.
- Pradhan, N.S., Prince, P.R., Madhumathi, J., Roy, P., Narayanan, R.B. & Antony, U. (2012). Protective immune responses of recombinant VP2 subunit antigen of infectious bursal disease virus in chickens. Veterinary Immunology and Immunopathology, 148, 293–301. doi: 10.1016/j.vetimm.2012.06.019
- Reddy, S.K., Sharma, J.M., Ahmad, J., Reddy, D.N., McMillan, J.K., Cook, S.M., Wild, M.A. & Schwartz, R.D. (1996). Protective efficacy of a recombinant herpesvirus of turkeys as an in ovo vaccine against Newcastle and Marek’s diseases in specific pathogen free chickens. Vaccine, 14, 469–477. doi: 10.1016/0264-410X(95)00242-S
- Reed, L.J. & Muench, H. (1938). A simple method of estimating fifty percent endpoints. The American Journal of Hygiene, 27, 493–497.
- Rodriguez-Avila, A., Oldoni, I., Riblet, S.M. & Garcia, M. (2007). Replication and transmission of live-attenuated infectious laryngotracheitis virus (ILTV) vaccines. Avian Diseases, 51, 905–911. doi: 10.1637/8011-041907-REGR.1
- Sharma, J.M. (1999). Introduction to poultry vaccines and immunity. Advances in Veterinary Medicine, 41, 481–494. doi: 10.1016/S0065-3519(99)80036-6
- Slacum, G., Hein, R. & Lynch, P. (2009). The compatibility of HVT recombinants with other Marek’s disease vaccines. 58th Western Poultry Disease Conference (p. 285), Sacramento, CA.
- Sondermeijer, P.J., Claessens, J.A., Jenniskens, P.E., Mockett, A.P., Thijssen, R.A., Willemse, M.J. & Morgan, R.W. (1993). Avian herpesvirus as a live viral vector for the expression of heterologous antigens. Vaccine, 11, 349–358. doi: 10.1016/0264-410X(93)90198-7
- Vagnozzi, A., Zavala, G., Riblet, S.M., Mundt, A. & Garcia, M. (2012). Protection induced by commercially available live-attenuated and recombinant viral vector vaccines against infectious laryngotracheitis virus in broiler chickens. Avian Pathology, 41, 21–31. doi: 10.1080/03079457.2011.631983
- Witter, R.L. & Hunt, H.D. (1994). Poultry vaccines of the future. Poultry Science, 73, 1087–1093. doi: 10.3382/ps.0731087