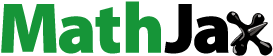
ABSTRACT
Riemerella anatipestifer (RA), the causative agent of infectious serositis that targets ducklings and other poultry, secretes protein via the type IX secretion system (T9SS). The proteins transported by T9SS are located on the bacterial cell surface or secreted into the extracellular milieu. In this study, a sprA deletion mutant was constructed encoding a core protein of T9SS to investigate its influence on outer membrane protein expression and its role in virulence. Compared with the wild-type RA-YM strain, the deletion mutant ΔsprA failed to digest gelatin, showed the same growth rate in the logarithmic phase and exhibited greater sensitivity to the bactericidal activity of duck sera, whereas the complemented strain restored these phenotypes. The outer membrane proteome of RA-YM and the ΔsprA mutant were analyzed by Tandem Mass Tags, which revealed 198 proteins with predicted localization to the cell envelope. Sixty-three of these proteins were differentially expressed in the outer membrane, with 43 up-regulated and 20 down-regulated. Among the twelve outer membrane proteins which were secreted by T9SS, four proteins were up-regulated and one protein was down-regulated. Animal experiments demonstrated that the median lethal dose of the mutant strain ΔsprA was about 500 times higher than that of the wild-type RA-YM strain, and bacterial loads in blood, brain, heart, liver and spleen of the ΔsprA-infected ducks were significantly reduced. Our results indicate that the SprA is a virulence-associated factor of RA, and its absence results in altered abundance of outer membrane proteins, and secretion disorders associated with some of the T9SS effector proteins.
Introduction
Riemerella anatipestifer (RA) is a short rod-shaped, aflagellar, nonspore-forming Gram-negative bacterium belonging to the family Flavobacteriaceae that infects ducks, geese, turkeys and other poultry (Segers et al., Citation1993; Subramaniam et al., Citation1997). Ducks infected with RA display lethargy, diarrhoea, respiratory and nervous signs, with a high rate of mortality that inflicts immense losses to the economy (Sandhu, Citation2008). As this bacterium has twenty serotypes and poor cross protection and resistance to antibacterial agents, it becomes increasingly more challenging to control (Sandhu, Citation2008; Zhong et al., Citation2009). Currently, it is one of the bacterial diseases that impose serious damage to the global duck industry.
The protein secretion machinery plays a critical role in the progression of microbial pathogenesis by secreting extracellular enzymes, proteinases, and virulence factors that destroy the host cells and induce the ensuing pathological damage and tissue necrosis (Sato, Citation2011). Recently, the type IX secretion system (T9SS), which is associated with motility, secretion of proteins and pathogenic mechanism of bacteria, has been found to be widespread among members of the phylum Bacteroidetes, e.g. Flavobacterium johnsoniae, Porphyromonas gingivalis, Tannerella forsythia (McBride & Zhu, Citation2013). The T9SS comprises a group of membrane or periplasmic proteins GldK, GldL, GldM, GldN, SprA, SprE, and SprT (Sato et al., Citation2010). Though the RA protein systems have not been studied comprehensively, genes encoding the T9SS proteins have been identified (Zhou et al., Citation2011; McBride & Zhu, Citation2013). SprT is a functional component of T9SS in RA that is responsible for the transport of gelatinase and is related to virulence (Guo et al., Citation2017b). SprA, one of the components of T9SS, is predicted to be localized in the outer membrane and is made up of 2340 amino acids(Nelson et al., Citation2007). Information about SprA is currently limited to F. johnsoniae, P. gingivalis and T. forsythia. It was reported that SprA was necessary for the secretion of the cell surface gliding motility adhesins SprB and RemA of F. johnsoniae (Shrivastava et al., Citation2013). The deletion of sov, a homologue of the sprA gene, obstructs the secretion of gingipains in P. gingivalis and results in the expression of less-glycosylated versions of the S-layer proteins TfsA and TfsB in T. forsythia (Saiki & Konishi, Citation2010; Narita et al., Citation2014).
T9SS secretes proteins either as large complexes on the bacterial cell surface or into the extracellular milieu (Sato, Citation2011). Given that the outer membrane proteins (OMPs) are hallmark structures of the Gram-negative bacterial cell wall, which function as the interface between the cell and its external environment, uncovering their distribution on the surface of bacteria is key to resolving the pathogenic mechanisms. In this light, this study constructed a deletion mutant of sprA (RAYM_09602), which encodes one of the core components of T9SS, and then studied its biological characteristics and pathogenicity. Outer membrane proteins of wild-type RA-YM and deletion mutant ΔsprA were extracted, and analyzed by the Tandem Mass Tags (TMT) technique. The bioinformatics-based identification and proteomic analysis of OMPs differentially secreted by T9SS, will facilitate understanding of the relationship between proteins secreted by T9SS and pathogenicity of RA.
Materials and methods
Bacterial strains, plasmids and culture conditions
The strains, plasmids and primers used in this study are listed in . The wild-type strain R. anatipestifer RA-YM was isolated from the brain tissue of a diseased duck and preserved in Huazhong Agricultural University Veterinary Microbiology Laboratory. R. anatipestifer strains were cultured at 37°C in 5% CO2 in tryptic soy agar (TSA, Difco, USA) or in tryptic soy broth (TSB, Difco, USA) with shaking at 200 rpm. E. coli strains were cultured at 37°C in Luria Bertani (LB) broth (Sigma-Aldrich, St. Louis, USA). When needed, the culture media used for both RA strains and E. coli strains were supplemented with antibiotics at the following final concentrations: spectinomycin (Spc), 100 μg/ml; chloramphenicol (Cm), 50 μg/ml; ampicillin (Amp), 50 μg/ml; kanamycin (Km), 50 μg/ml, Diaminopimelic acid (DAP), 50 μg/ml.
Table 1. Strains, plasmids and primers used in this study.
Construction of mutant strain ΔsprA and complemented strain CΔsprA
The 1055-bp fragment (Leftarm) and 1226-bp fragment (Rightarm) of the sprA gene were amplified from RA-YM genomic DNA using primers sprA-F1, sprA-F2 and sprA-R1, sprA-R2, respectively (). The 1086-bp spectinomycin resistance gene (Spc) fragment was amplified from plasmid pIC333 using the primers spc-F1 and spc-F2. The integrated fragment LSR that contains the Leftarm fragment, Spc fragment, and the Rightarm fragment was produced by overlap PCR and then cloned into the pMD18-T vector to generate pMD-18T-LSR. The pMD-18T-LSR and pRE112 plasmids were digested with KpnI and SacI, and then the LSR fragment and pRE112 skeleton fragment were ligated to create the recombinant suicide plasmid pRE112-LSR. The pRE112-LSR plasmid was transformed into the E. coli strain X7213, acting as the donor strain. The mutant strain ΔsprA was constructed by allelic exchange through the recombinant suicide plasmid pRE112. The mutant was selected by culture in the presence of Spc (100 μg/ml) and verified by PCR amplification of the spc gene and sprA gene using primers spc-F1 plus spc-F2 and sprA-N1 plus sprA-N2 which was not available to the wild-type strain RA-YM.
The complemented strain was constructed by allelic exchange through the shuttle plasmid named pRES-JX-bla, which was derived from the plasmid pRE112 by replacing the replication region with the putative replication region of R. anatipestifer plasmid RA-JX (Guo et al., Citation2017a). The sprA was amplified from the RA-YM genomic DNA using the primers Promoter-sprA1 plus Promoter-sprA2 and sprA-CF plus sprA-CR. The promoter fragment and the coding fragment were joined together by overlap PCR. It was then digested with KpnI and SacI and cloned into pRES-JX-bla to create plasmid pRES-JX-bla-sprA. The recombinant plasmid pRES-JX-bla-sprA was conjugated into the mutant strain RA-YMΔsprA. The transconjugants were screened on TSA supplemented with Amp and further verified by PCR amplification.
Characterization of mutant strain ΔsprA and the complemented strain CΔsprA
To determine whether the absence of sprA gene has an effect on bacterial growth, the growth curves of mutant strain ΔsprA, the complemented strain CΔsprA, and the wild-type strain RA-YM were measured as described previously (Guo et al., Citation2017a). Briefly, the strains were cultured for about 2 days at 37°C in 5% CO2 in TSA medium and were inoculated into TSB. After reaching the mid-exponential phase, the culture was diluted with fresh TSB medium to an optical density of 1.0 at 600 nm (OD600). An equal amount of each bacterial culture was then transferred into fresh TSB medium at a ratio of 1:1000 (v/v) for growing at 37°C with shaking at 200 rpm. Bacterial growth rate was expressed in terms of OD600 values, which were measured at 1 h intervals for 19 h using a spectrophotometer (BIO-RAD, USA).
The biochemical characteristics of the mutant strain ΔsprA, the complemented strain CΔsprA and the wild-type strain RA-YM were detected with bacterial biochemical trace identification tubes (Hope Bio, Qingdao, China), including glucose, lactose, maltose, mannitol, arabinose, sucrose, citrate, hydrogen sulphde, nitrate, carbamide, and gelatin.
To visually characterize the presence of liquefied gelatin, RA-YM, ΔsprA, and CΔsprA cells were inoculated into TSB. The overnight cultures (5 μl) were spotted on solid nutrient agar medium containing 0.3% gelatin and cultured at 37°C for 24 h. Subsequently, acid mercuric chloride (2–3 ml) was added to the plates. The result was observed after 5 min. As described in previous studies, the bacteria which could liquefy gelatin were seen as a transparent halo around their bacterial lawn (Frazier, Citation1926; Thomas et al., Citation2014).
Serum survival assay
Blood was withdrawn from 12 healthy 30-day-old Cherry Valley ducks without anti-R. anatipestifer antibody, and then immediately placed on ice. After clotting for 2 h, the blood samples were centrifuged at 2000 × g for 10 min at 4°C. Then serum samples were collected and stored at −80°C until use.
To perform the serum survival assay, mutant strain ΔsprA and wild-type strain RA-YM were cultured in TSB to an OD600 of 0.8. The cells were washed and resuspended to 1.0 × 106 colony forming units (CFU)/ml in Hanks Balanced Salt Solution (HBSS) (Gibco) with 0.15 mM calcium and 1 mM magnesium (HBSS++). After incubation at 37°C for 30 min with or without duck serum, the mixture was 10-fold serially diluted and plated onto TSA plates. Colonies were counted after 48 h incubation. Heat-inactivated serum used in this experiment was generated by incubating normal duck serum at 56°C for 30 min. The control group was processed with bacteria resuspended in HBSS++ to prevent replication and viability of strains (Rosadini et al., Citation2014). The survival rate was calculated using the following formula:
Isolation of outer membrane proteins (OMPs)
Outer membrane extracts were isolated from bacterial pellets based on French press cell lysis followed by treatment with chaotropic reagents (Thein et al., Citation2010). Fifty milliters of cells were pelleted and resuspended in pre-cold Tris-HCl (6 ml, 0.1 M, pH 7.3). The cells were ruptured twice in a French press at 100 bar. Incompletely lysed cells were removed by centrifugation at 4000 × g for 20 min at 4°C (Eppendorf 5810 R centrifuge). The supernatant was diluted with pre-cold sodium carbonate (0.1 M, pH 11) to a final volume of 60 mL and stirred for 1 h at 4°C. The suspension was then ultracentrifuged at 100,000 × g for 1 h at 4°C. The pellet containing the OM was washed in Tris-HCl (2 ml, 0.1 M, pH 7.3), centrifuged at 100,000 × g for 20 min at 4°C, and finally dissolved in 500 µl SDT lytic solution (4% SDS, 100 mM Tris-HCl, 1 mM DTT, pH 7.6). The samples were quantified using the BCA Protein Assay Kit (Bio-Rad, USA). The outer membrane proteins samples of wild-type RA-YM and mutant strain ΔsprA were each prepared three times, and the samples were stored at −80°C until use.
Assessment of OMP quality
SDS-PAGE
Sodium dodecyl sulphate polyacrylamide gel electrophoresis (SDS-PAGE) was performed to detect parallelism of outer membrane extracts in RA-YM and ΔsprA. Twenty micrograms of proteins from each sample were mixed with 5 × loading buffer and boiled for 5 min before loading the gel and then the proteins were separated on 12.5% SDS-PAGE gel (constant current 14 mA, 90 min). Protein bands were visualized by Coomassie Blue R-250 staining.
Fasp digestion
Filter-aided sample preparation (FASP) digestion was performed as previously described (Wisniewski et al., Citation2009). As detailed here, 200 μg of proteins from each sample were incorporated into 30 μl SDT buffer. The detergent, DTT, as well as other low-molecular-weight components, were removed by repeated ultrafiltration (Microcon units, 10 kD) using UA buffer (8 M Urea, 150 mM Tris-HCl, pH 8.0). Subsequently, 100 μl iodoacetamide (100 mM IAA in UA buffer) was added to alkylate and block reduced cysteine residues and the samples were incubated for 30 min in darkness. The filters were washed three times with 100 μl UA buffer and then twice with 100 μl 100 mM TEAB buffer. Finally, the protein suspensions were digested overnight at 37°C with 4 μg trypsin (Promega) in 40 μL TEAB buffer, and the peptides yielded were collected as filtrate. The concentration of the peptides was estimated by a UV spectrometer (absorbance at 280 nm) assuming an extinction coefficient of 1.1 of 0.1% (g/l) solution, which was calculated based on the frequency of tryptophan and tyrosine in vertebrate proteins.
LC-MS/MS analysis
LC-MS/MS analysis was performed for 60/90 min on a Q Exactive mass spectrometer (Thermo Scientific, USA) that was coupled to Easy nLC (Proxeon Biosystems, now Thermo Fisher Scientific, USA) in the positive ion mode with the peptide recognition mode enabled. MS data were recorded using a data-dependent top10 method by dynamically choosing the most abundant precursor ions from the survey scan (300–1800 m/z) for the high energy C trap dissociation (HCD) fragmentation. Automatic gain control (AGC) target was set to 3e6, and maximum inject time to 10 ms. Dynamic exclusion duration was 40.0 s. Survey scans were obtained at a resolution of 70,000 at m/z 200, the resolution for HCD spectra was set to 17500 at m/z 200 (TMT 6plex), and the isolation width was 2 m/z. Normalized collision energy was 30 eV and the underfill ratio, which specifies the minimum percentage of the target value that is likely to be reached at maximum fill time, was defined as 0.1%.
TMT labelling and peptide fractionation with high pH reversed-phase
A 100-μg peptide mixture was labelled for each sample, using TMT reagent according to the instruction from the manufacturer (Thermo Fisher Scientific). TMT-labeled digest samples were fractionated into 10 fractions by an increasing acetonitrile step-gradient elution, using the Pierce High pH Reversed-Phase Fractionation Kit (Thermo scientific, USA).
Identification and quantification of the outer membrane extracts
MS/MS spectra were searched using MASCOT engine (Matrix Science, London, UK; version 2.2) embedded into Proteome Discoverer 1.4. The data were analyzed by MASCOT 2.2 software and protein identification was achieved using UniProt_Riemerella-Anatipestifer_1940_20160912.fasta database (Riemerella anatipestifer strain ATCC 11845/ DSM 15868/ JCM9532/ NCTC 11014) (http://www.uniprot.org). Trypsin was used as the required enzyme, and two missed cleavages were permitted. Carbamidomethylation of cysteine was set as a fixed modification, while TMT 6 plex labelling at N-terminal, K, and Y were used as variable modifications for the oxidation of methionine. The peptide mass tolerance allowed for precursor mass was set to 20 ppm, and fragment mass tolerance was 0.1 Da. Protein quantification required at least one unique peptide; FDR less than 0.01 was deemed acceptable for both the peptide and protein level.
The theoretical pIs and molecular weights (MW) of the identified proteins were obtained from the protein sequence data bank UniProt. Functional enrichment analysis was achieved via Gene Ontology (GO) (http://www.geneontology.org/) and Kyoto Encyclopedia of Genes and Genomes (KEGG) (http://www.genome.jp/kegg/).
The determination of bacterial virulence in vivo
One-hundred-and-eighty healthy ten-day-old Cherry Valley ducklings were purchased from Xinzhou Duck Farm (Wuhan, China). The ducklings had access to food and water ad libitum throughout the day and night. Animal experiments performed in this study were approved by the Institution Animal Experiment Committee of the Veterinary Faculty of the Huazhong Agriculture University.
The median lethal dose (LD50) was measured as described in the previously published study (Guo et al., Citation2017a). Briefly, to measure each strain, the 13-day-old healthy Cherry Valley ducks were divided evenly into five groups (ten ducks/group). The ducklings were injected intramuscularly with bacteria at 1.0 × 105, 1.0 × 106, 1.0 × 107, 1.0 × 108, and 1.0 × 109 CFU, respectively. The measured limit of infectious doses was determined following previous studies (Pathanasophon et al., Citation1996; Zhou et al., Citation2013). Moribund ducks were killed humanely and counted as dead. The mortality of each dose group was recorded up to 7 days after injection. The LD50 was calculated by Karber’s method (Gu et al., Citation2009).
The bacterial loads in the blood, heart, liver, brain, and spleen of ducklings infected with RA-YM and ΔsprA were compared to evaluate bacterial survival in vivo. Briefly, thirty 13-day-old Cherry Valley ducklings were injected intramuscularly with 1.0 × 107 CFU. Blood, heart, liver, brain, and spleen samples were collected at 24 h, 48 h post-inoculation (three ducklings per group), diluted appropriately and plated on TSA for bacterial counting. Meanwhile, the spleen, liver, heart, and brain tissue slices were prepared for pathological sections.
Statistical analysis
Statistical analysis was carried out by using SPSS v.18.0 (SPSS Inc., Chicago, IL, USA). In all cases, a P-value less than 0.05 was defined as statistically significant based on Duncan’s multiple-range test.
Results
Identification and characterization of mutant strain ΔsprA and the complemented strain CΔsprA
The RA-YM ΔsprA gene-deleted mutant strain was constructed by allelic exchange, which was replaced by a Spc cassette. The mutant strain ΔsprA was screened on TSA plates containing Spc (100 μg/mL), and verified by PCR amplification of the spc gene and deleted sprA fragments from mutant strain ΔsprA ((A)). The complemented strain was also verified by PCR ((B)).
Figure 1. Identification and characterization of mutant strain ΔsprA and complemented strain CΔsprA. Usually, a combined PCR method is used to verify the gene deletion strain. In this study, we determine whether homologous recombination occurred by amplifying spc gene. In terms of experimental design, the leftarm (1055 bp) and rightarm (1226 bp) of the constructed suicide plasmid were 5742 bp (containing a 121 bp fragment) shorter than the target sprA gene (7023 bp), which could be used as an internal control to determine whether the complete recombination occurred. If the gene deletion strain could not amplify this 121 bp fragment, it indicated that the gene deletion strain was successfully constructed; otherwise, the construction of the gene deletion strain had failed. Thus, in the gene deletion strain, the 121 bp fragment could not be amplified (lane 4), while the spc gene could be amplified (lane 2). The gene of the wild-type strain did not change, thus, in the wild-type strain, the 121 bp fragment could be amplified (lane 3), but the spc gene could not be amplified (lane 1). (A) PCR amplification of the spc gene and the deleted part of the sprA gene. Lane M: DL2000 DNA Marker. Lane 1: No fragment was amplified from wild-type strain RA-YM using primer pairs spc-F1/spc-F2 for the spc gene; Lane 2: The spc gene (1086 bp) was amplified from mutant strain ΔsprA using primer pairs spc-F1/spc-F2; Lane 3: The deleted fragment (121 bp) was amplified from wild-type strain RA-YM using primer pairs sprA-N1 and sprA-N2; Lane 4: No fragment was amplified from mutant ΔsprA using primer pair sprA-N1 and sprA-N2 for deleted fragment. (B) PCR amplification of complemented strain CΔsprA. Lane M: DL2000 DNA Marker. Lane 1: The deleted fragment (121 bp) was amplified from complemented strain CΔsprA using primer pair sprA-N1 and sprA-N2; Lane 2: The deleted fragment (121 bp) was amplified from wild-type strain RA-YM using primer pair sprA-N1 and sprA-N2; Lane 3: The Amp gene (1019 bp) was amplified from complemented strain CΔsprA using primer pairsbla-F1/bla-F2; Lane 4: No fragment was amplified from wild-type strain RA-YM using primer pairs bla-F1/bla-F2 for Amp gene. (C) Growth curves. The mutant strain ΔsprA, wild-type strain RA-YM and the complemented strain CΔsprA were grown on TSB medium, and growth of each strain was monitored by measuring the OD600 values. (D) Liquefied gelatin following cultivation of RA-YM, ΔsprA, and CΔsprA on a nutrient gelatin plate.
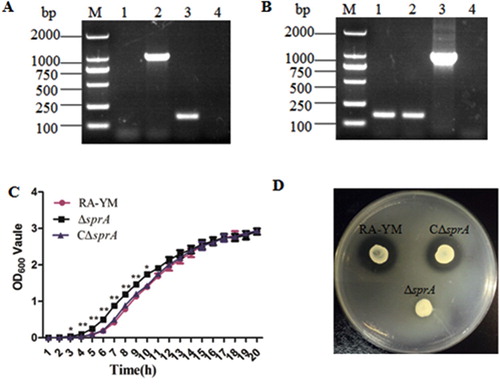
The growth characteristic results showed there was no difference among the wild-type RA-YM, mutant strain ΔsprA and the complemented strain CΔsprA except in the lag phase. The OD600 value of ΔsprA was higher than that of wild-type RA-YM from 3-10 h (P < 0.05) ((C)).
Biochemical analysis demonstrated that both were incapable of fermenting saccharides (glucose, lactose, maltose, sucrose, mannitol, arabinose), and producing urease. However, RA-YM liquefied gelatin while ΔsprA did not ((D)).
The serum survival assay was conducted to compare the ability of the mutant strain ΔsprA in resisting the complement-mediated killing with its wild-type strain RA-YM. When wild-type strain RA-YM was incubated with 12.5% normal duck serum at 37°C for 30 min, the survival rate of RA-YM was 117.5%. In sharp contrast, the survival rate of mutant strain ΔsprA incubated under the same conditions was 0.87%, whereas that of complemented strain CΔsprA was 95.1% in 12.5% normal duck serum, which was comparable to the rate of RA-YM ((A)). The 12.5% serum was effective in killing the mutant strain ΔsprA, which displayed higher sensitivity to killing by serum than the wild-type strain RA-YM.
Figure 2. (A) Serum survival of wild-type strain RA-YM, mutant strain ΔsprA, and complemented strain CΔsprA. Effect of sprA mutation on resistance of RA to duck serum. Strains were treated with 12.5% normal duck serum for 30 min at 37°C and plated for survival analysis. Data were analyzed with Student’s t-test (*P < 0.05, **P < 0.01). (B) SDS-PAGE of outer membrane extracts of RA-YM and ΔsprA. M: Protein marker; Lane 1: The outer membrane extract of RA-YM; Lane 2: The outer membrane extract of ΔsprA.
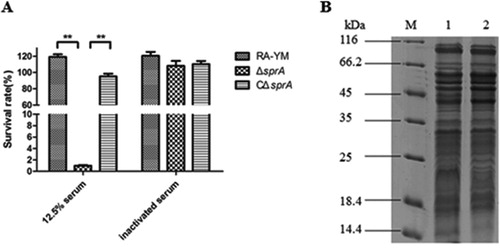
Identification and quantification of the OMPs
A preliminary experiment was conducted to ensure the smooth conduct of the TMT quantitative proteomics experiment. Outer membrane extracts of RA-YM and ΔsprA were analyzed with SDS-PAGE ((B)), FASP Digestion, and LC-MS/MS in order to detect parallelism of protein expression patterns between samples, evaluate the total contents and concentration of protein, and judge rationality of the selected protein database query. The results showed the preliminary test operated normally (data not shown), and the subsequent trials could be carried out.
TMT labelling and LC-MS/MS were used to compare outer membrane protein expression between wild-type strain RA-YM and mutant strain ΔsprA. The obtained data were searched and analyzed in uniprot_Riemerella-Anatipestifer_1940_20160912.fasta (http://www.uniprot.org). In this database, the type strains used for analysis include Riemerella anatipestifer (strain ATCC 11845 / DSM 15868 / JCM 9532 / NCTC 11014), but exclude the experimental strain RA-YM that was used in this study. Therefore, we had to obtain the corresponding outer membrane proteins locus of the RA-YM strain through NCBI Protein BLAST analysis. A total of 1243 proteins were identified using at least one unique peptide with ≥95% confidence (Table S1). Among these, 307 proteins were considered differentially expressed based on an operationally defined ratio of 1.2 fold-change (up- or down-regulation) plus P < 0.05. Of this differentially expressed subpopulation, 137 proteins were up-regulated and 170 were down-regulated (data not shown).
Different algorithms were conducted to obtain an estimate of the theoretical number of OMPs. As a result, an online predictive software, CELLO v.2.5, was selected due to its high accuracy in predicting OMPs (Yu et al., Citation2006). By referring to the RA-YM genome sequence (Accession: NZ_AENH00000000), among the 1243 predicted OMP proteins, 198 proteins were identified as outer membrane proteins (Table S2), and 63 of them were differentially expressed, with 43 up-regulated and 20 down-regulated (shown in and ).
Table 2. Up-regulated outer membrane proteins in mutant strain ΔsprA.
Table 3. Down-regulated outer membrane proteins in mutant strain ΔsprA.
GO and KEGG pathway analysis of differentially expressed OMPs
According to GO annotation, functions of the identified differentially-expressed proteins were classified into two major categories: biological process and molecular function. Among the 43 up-regulated OMPs, the most general biological process was signal transduction, while other proteins participated in proteolysis, membrane assembly, DNA duplex unwinding, and nucleic acid phosphodiester bond hydrolysis. The most common molecular function was protein binding, while other proteins possessed DNA binding, endonuclease activity, transporter activity and receptor activity ((A,B)). For the 20 down-regulated OMPs, the most general biological process was also signal transduction, while other proteins took part in proteolysis, phosphorylation, cell redox homeostasis and peptidoglycan catabolic processes. The most common molecular function was also protein binding, while other proteins possessed receptor activity, catalytic activity, kinase activity, transferase activity, hydroxyisourate hydrolase activity and N-acetylmuramoyl-L-alanine amidase activity ((C,D)).
Figure 3. (A) GO annotation for differentially expressed OMPs of up-regulated proteins; (B) Molecular function of up-regulated proteins; (C) GO annotation of down-regulated proteins; (D) Molecular function of down-regulated proteins.
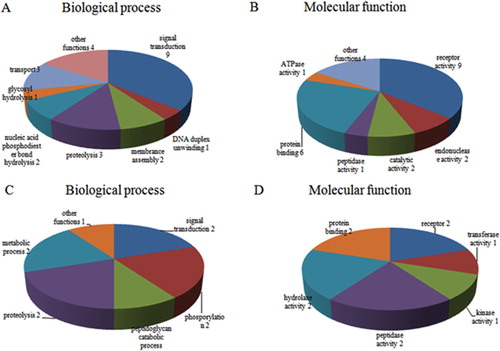
Annotations for only five of the 63 differentially expressed OMPs were found in the KEGG database. Two up-regulated OMPs were respectively involved in mismatch repair, and nucleotide excision repair; three down-regulated OMPs participated in purine metabolism, terpenoid backbone biosynthesis, and cationic antimicrobial peptide (CAMP) resistance respectively (data not shown).
Analysis of OMPs secreted by T9SS
Proteins secreted by T9SS have N-terminal signal peptides, which allow for transit across the inner membrane through the Sec system, and conserved C-terminal domains (CTDs) that direct secretion across the outer membrane (Glew et al., Citation2012; McBride & Zhu, Citation2013). The conserved domains of the 198 identified OMPs were compared on the basis of the structure of CTDs which typically belong to TIGRFAM family TIGR04131 or TIGR04183 (Kharade & McBride, Citation2014). The results found 12 proteins with CTDs that were likely located in the outer membrane, including four up-regulated proteins (RAYM_08375, RAYM_01812, RAYM_06120, RAYM_07574) and one down-regulated protein (RAYM_02622) (shown in ).
Table 4. CTD proteins identified in RA-YM outer membrance by TMT analysis.
Determination of the bacterial pathogenicity in vivo
The results indicated that the LD50 of ΔsprA was 3.61 × 107 CFU, about 500 times higher than that of wild-type strain RA-YM, whose LD50 was 7.21 × 104 CFU. The virulence of the complemented strain CΔsprA was rescued by complementation (5.34 × 105 CFU). It demonstrated that, compared to the wild-type RA-YM strain, the virulence of the mutant ΔsprA was significantly attenuated. The bacterial load in blood, heart, liver, brain, and spleen of infected ducks was quantified. The bacterial load of mutant strain ΔsprA in blood and spleen was significantly lower than that of wild-type strain RA-YM 24 h after infection ((A)). The bacterial load of mutant strain ΔsprA in blood, liver, brain, and spleen was also obviously lower than that of wild-type strain RA-YM after 48 h infection ((B)). Tissue sections confirmed the above mentioned results. For the RA-YM group, noticeable congestion in hepatic sinusoids, fatty degeneration of hepatocytes, and a great quantity of epicardial fibrin exudation and inflammatory cells were observed at both 24 h and 48 h post-challenge; in the subarachnoid space epicardial there was fibrin exudation and inflammatory cell infiltration at 48 h after infection; meanwhile, lymphocyte proliferation of splenic white pulp was noticed at 48 h post challenge. The gross lesions of the mutant strain ΔsprA group were mild at 24 h and 48 h post-challenge, while no apparent gross lesions were observed in the control group ( and ).
Figure 4. Bacterial loads of tissues. (A) Tissue samples were collected at 24 h post-challenge. (B) Tissue samples were collected at 48 h post-challenge. Data were presented as mean ± standard deviations from five infected ducks. Student’s t-test was used to analyse the data (*P < 0.05, **P < 0.01).
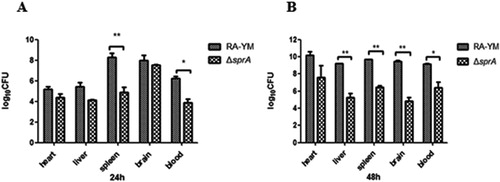
Figure 5. Photomicrograph of histopathological study on tissues from ducklings infected with wild-type strain RA-YM or mutant strain ΔsprA at 24 h post-challenge (HE, × 400). The arrows in the figure indicate the normal (control group) and lesion (RA-YM or ΔsprA infected group) regions of the organs at 24 h post-challenge. Compared with the control group (the left column), there were obvious histopathological changes in the wild-type RA-YM infected group (the middle column): in heart, the visceral pericardium became thickened due to fibrin exudation and inflammatory cell infiltration; in liver, severe fatty degeneration was found, and white pulp lymphocytes hyperplasia occurred in the spleen; no obvious pathological change was found in brain tissue. The histopathological change in the mutant strain ΔsprA infected group (the right column) was slight, thus the tissues from this group looked similar to those of the control group.
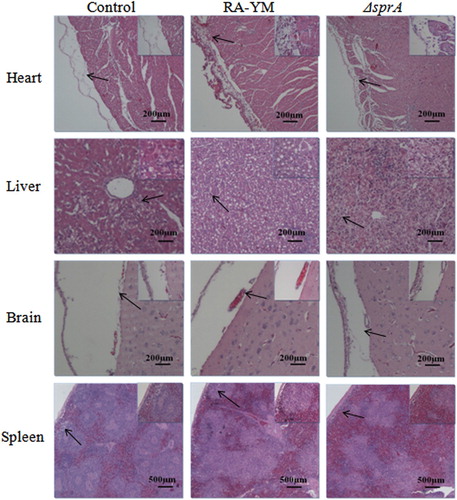
Figure 6. Photomicrograph of histopathological study on tissues from ducklings infected with wild-type strain RA-YM or mutant strain ΔsprA at 48 h post-challenge (HE × 400). The arrows in the figure indicate the normal (control group) and lesion (RA-YM or ΔsprA infected group) regions of the organs at 48 h post-challenge. Compared with the control group (the left column), there were obvious histopathological changes in the wild-type RA-YM infected group (the middle column): in heart, epicardium became thickened due to fibrin exudation and interstitial inflammatory cell infiltration; the spaces between myocardial fibres were significantly widened due to oedema; in liver, moderate to marked congestion was found in central vein and hepatic sinus, and fatty degeneration of the hepatic cells was more severe; in brain, fibrin exudation and inflammatory cell infiltration also occurred in cerebral arachnoid cavity; in spleen, white pulp lymphocyte hyperplasia occurred, and boundaries between white and red pulp became blurred, while germinal centres disappeared. The histopathological change in the mutant strain ΔsprA infected group (the right column) was slight, thus the tissues from this group looked similar to those of the control group.
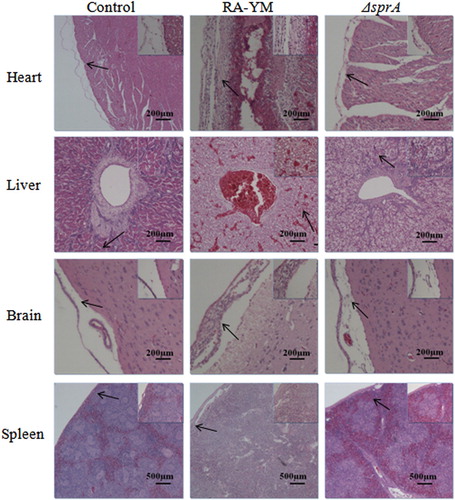
Discussion
R. anatipestifer has the ability to colonize and develop in host tissues, quickly reproducing and spreading throughout the body, leading to septicaemia and polyserositis (Sandhu, Citation2008). However, until now, the pathogenic mechanism has not been elucidated. It is known that T9SS is related to pathogenicity in several species of the phylum Bacteroidetes (Saiki & Konishi, Citation2010; Sato et al., Citation2010; Kharade & McBride, Citation2014). In the present study, a mutant strain ΔsprA encoding components of the T9SS was constructed. The biochemical characteristic profiles of the wild-type strain RA-YM and mutant strain ΔsprA were similar except that the mutant strain ΔsprA was incapable of degrading gelatinase. It has been reported that the gelatinase RAYM_01812 is transported by T9SS (Guo et al., Citation2017b). Thus, SprA is functional in R. anatipestifer, similar to its orthologues in F. johnsoniae and P. gingivalis. From the results of the animal infection experiment, the mutant strain ΔsprA displayed virulence that was attenuated by approximately 500-fold in ducks, and bacterial loads and histopathological observations revealed that the mutant strain ΔsprA also possesses attenuated virulence compared to wild-type RA-YM. These results indicated that the sprA gene is an important factor related to pathogenicity of R. anatipestifer. SprA, a component of the T9SS, is a membrane protein partially exposed on the cell surface, and is involved in secretion of gingipains, which were related to aggressive and chronic periodontitis, and in secretion of cell surface F. johnsoniae gliding motility adhesins SprB and RemA (Saiki & Konishi, Citation2010; Shrivastava et al., Citation2013). Therefore, it is concluded that SprA has a functional role in RA and it is a virulence-associated factor by virtue of its ability to change the abundance of outer membrane proteins, especially those effector proteins transported by T9SS.
The outer membrane is an important component of Gram-negative bacteria, which is related to resistance to antibiotics, digestive enzymes and immune surveillance (Nikaido, Citation2003). Since it is the point of contact for the bacterial cell and its environment, including host cells, most virulence factors in pathogenic bacteria would be expected to be localized on the cell surface. Nonetheless, there is a dearth of information about the outer proteome of RA and about the proteins that are located on the bacterial cell surface or secreted into extracellular milieu via T9SS. In this study, we focused on the differential expression of outer membrane proteins caused by the secretion disorder of the type IX secretion system after SprA protein deletion, which should be consistent in vivo and in vitro. Therefore, TSB medium and the culture conditions were selected to evaluate protein differential expression. Finally, a total of 1243 proteins were identified using TMT relative quantitative proteomics of R. anatipestifer OMPs, 198 (approximately 15.9%) of which were annotated as OMPs by CELLO v.2.5. The results agreed with a study on the E. coli OM proteome, which isolated about 13% E. coli OMPs using chaotropic reagents Na2CO3 (Thein et al., Citation2010).
The comparison of OMPs between mutant strain ΔsprA and wild-type RA-YM was performed to identify the differentially expressed proteins. Quantitative screening uncovered 63 differentially expressed OMPs, among which 43 proteins were up-regulated proteins and 20 were down-regulated. Because the relevant protein database is incomplete, there is a lack of some annotation information regarding GO and KEGG of the 63 differential proteins. With limited annotation information, the results of the present study point to these differential proteins mainly being involved in protein binding, receptor activity, DNA binding in up-regulated proteins, and protein binding and enzymatic activity in down-regulated proteins. Among the up-regulated proteins, there were several that belonged to the TonB-dependent outer-membrane protein family, such as RAYM_00850, RAYM_07669, and RAYM_05236. TonB-dependent outer-membrane bacterial proteins are important components for the uptake of substrates from the environment and transportation of substrates across the outer membranes of Gram-negative bacteria (Chimento et al., Citation2005; Wang et al., Citation2014). RA has 35 TonB-dependent outer membrane proteins, so that it has many transport systems. It was speculated that when sprA was deleted, other transport systems would be upregulated to maintain bacteria survival. This may explain the finding of a more rapid growth in the early logarithmic phase in the ΔsprA deletion mutant.
RAYM_07929, encoding cell wall hydrolase, was down-regulated when sprA was deleted. It was reported that cell wall hydrolase plays a crucial role in cell division, growth and virulence in Streptococcus pyogenes (Pancholi et al., Citation2010). In addition, RAYM_05141 and RAYM_09587 belong to the RagA/SusC family of outer membrane proteins. RAYM_09592 is a RagB/SusD family nutrient uptake outer membrane protein. RagA and RagB are stabilized on the cell surface, which constitutes the immunodominant outer membrane. It was demonstrated that they function as active transporters of protein degradation products and virulence factors in P. gingivalis (Nagano et al., Citation2007). RagB, acting as a Toll-like receptor agonist, can promote multiple inflammatory mediators, influencing epithelial cell invasion and virulence (Goulas et al., Citation2016). This might be partially responsible for the lower pathogenicity of mutant strain ΔsprA.
Proteins secreted by the T9SS are either located on the bacterial cell surface or in the extracellular milieu (Sato, Citation2011). Among the 63 proteins, 12 proteins with CTDs belonging to TIGR04183 or TIGR04131 are located on the outer membrane or are extracellular secretory proteins transported by the T9SS, four of which were up-regulated, including RAYM_08375, RAYM_01812, RAYM_03382 and RAYM_07574, and one down-regulated, RAYM_02622. RAYM_01812 is a subtilisin-like serine protease that has gelatinase activity (Guo et al., Citation2017b). Because of the deletion of the sprA gene, these proteins, notably RAYM_01812, cannot be secreted in the active form outside the bacteria, resulting in their accumulation in the lipid bilayer of the outer membrane. Gelatinase can enhance pathogenicity by degrading host collagen and providing nutrients for the pathogen. Subtilisin-like proteases are responsible for the complement C3 cleavage that is involved in pathogen virulence in T. forsythia, Enterococcus, and Streptococcus (Sifri et al., Citation2002; Bonifait et al., Citation2011; Ksiazek et al., Citation2015). EcpS, a gelatinase, has typical CTDs that belong to TIGRFAM family TIGR04183. It has been reported that they strongly inhibit the complement-dependent lysis and phagocytosis of RA (Fan et al., Citation2017). RAYM_07574 also contains a domain of fibronectin type III. Fibronectin had an influence on the bacterial adhesion and invasion, thereby impacting bacterial virulence (Somarajan et al., Citation2015; Hymes & Klaenhammer, Citation2016). Therefore, the four up-regulated proteins secreted by T9SS may be important virulence factors for R. anatipestifer pathogenicity, and warrant additional characterization of their roles in the pathogenic mechanisms.
In conclusion, SprA is involved in R. anatipestifer pathogenicity presumably because of its role in T9SS-mediated secretion of effector proteins. The relationship between these effector proteins and pathogenicity of R. anatipestifer requires further experimental explorations.
Supplemental Material
Download MS Excel (254.6 KB)Supplemental Material
Download MS Excel (28.7 KB)Acknowledgements
This work was supported by the Fundamental Research Funds for the Central Universities (Program No. 2662016PY103).
Disclosure statement
No potential conflict of interest was reported by the authors.
Additional information
Funding
References
- Bonifait, L., Vaillancourt, K., Gottschalk, M., Frenette, M. & Grenier, D. (2011). Purification and characterization of the subtilisin-like protease of Streptococcus suis that contributes to its virulence. Veterinary Microbiology, 148, 333–340. doi: 10.1016/j.vetmic.2010.09.024
- Chimento, D.P., Kadner, R.J. & Wiener, M.C. (2005). Comparative structural analysis of TonB-dependent outer membrane transporters: implications for the transport cycle. Proteins, 59, 240–251. doi: 10.1002/prot.20416
- Fan, M.N., Chen, S.H., Zhang, L.D., Bi, J.X., Peng, J.S., Huang, X.Y., Li, X., Li, H., Zhou, Q., Jiang, S. & Li, J.X. (2017). Riemerella anatipestifer extracellular protease S blocks complement activation via the classical and lectin pathways. Avian Pathology, 46, 426–433. doi: 10.1080/03079457.2017.1301648
- Frazier, W.C. (1926). A method for the detection of changes in gelatin due to bacteria. Journal of Infectious Diseases, 39, 302–309. doi: 10.1093/infdis/39.4.302
- Glew, M.D., Veith, P.D., Peng, B., Chen, Y.Y., Gorasia, D.G., Yang, Q., Slakeski, N., Chen, D., Moore, C., Crawford, S. & Reynolds, E.C. (2012). Pg0026 is the C-terminal signal peptidase of a novel secretion system of Porphyromonas gingivalis. Journal of Biological Chemistry, 287, 24605–24617. doi: 10.1074/jbc.M112.369223
- Goulas, T., Garcia-Ferrer, I., Hutcherson, J.A., Potempa, B.A., Potempa, J., Scott, D.A. & Gomis-Rüth, F.X. (2016). Structure of RagB, a major immunodominant outer-membrane surface receptor antigen of Porphyromonas gingivalis. Molecular Oral Microbiology, 31, 472–485. doi: 10.1111/omi.12140
- Gu, B., Li, Y.P., Yu, R.Y. & Wang, X.R. (2009). Summary of median lethal dose and its calculation methods. China Occupational Medicine, 72, 507–508.
- Guo, Y.Q., Hu, D., Guo, J., Li, X.W., Guo, J.Y., Wang, X.L., Xiao, Y.C., Jin, H., Liu, M., Li, Z.L., Bi, D.R. & Zhou, Z.T. (2017a). The role of the regulator fur in gene regulation and virulence of Riemerella anatipestifer assessed using an unmarked gene deletion system. Frontiers in Cellular and Infection Microbiology, 7, 382. doi: 10.3389/fcimb.2017.00382
- Guo, Y.Q., Hu, D., Guo, J., Wang, T., Xiao, Y.C., Wang, X.L., Li, S.W., Liu, M., Li, Z.L., Bi, D.R. & Zhou, Z.T. (2017b). Riemerella anatipestifer type IX secretion system is required for virulence and gelatinase secretion. Frontiers in Microbiology, 8, 2553. doi: 10.3389/fmicb.2017.02553
- Hymes, J.P. & Klaenhammer, T.R. (2016). Stuck in the middle: fibronectin-binding proteins in gram-positive bacteria. Frontiers in Microbiology, 7, 1504. doi: 10.3389/fmicb.2016.01504
- Kharade, S.S. & McBride, M.J. (2014). Flavobacterium johnsoniae chitinase ChiA is required for chitin utilization and is secreted by the type IX secretion system. Journal of Bacteriology, 196, 961–970. doi: 10.1128/JB.01170-13
- Ksiazek, M., Mizgalska, D., Eick, S., Thøgersen, I.B., Enghild, J.J. & Potempa, J. (2015). Klikk proteases of Tannerella forsythia: putative virulence factors with a unique domain structure. Frontiers in Microbiology, 6, 312. doi: 10.3389/fmicb.2015.00312
- McBride, M.J. & Zhu, Y.T. (2013). Gliding motility and por secretion system genes are widespread among members of the phylum Bacteroidetes. Journal of Bacteriology, 195, 270–278. doi: 10.1128/JB.01962-12
- Nagano, K., Murakami, Y., Nishikawa, K., Sakakibara, J., Shimozato, K. & Yoshimura, F. (2007). Characterization of RagA and RagB in Porphyromonas gingivalis: study using gene-deletion mutants. Journal of Medical Microbiology, 56, 1536–1548. doi: 10.1099/jmm.0.47289-0
- Narita, Y., Sato, K., Yukitake, H., Shoji, M., Nakane, D., Nagano, K., Yoshimura, F., Naito, M. & Nakayama, K. (2014). Lack of a surface layer in Tannerella forsythia mutants deficient in the type IX secretion system. Microbiology, 160, 2295–2303. doi: 10.1099/mic.0.080192-0
- Nelson, S.S., Glocka, P.P., Agarwal, S., Grimm, D.P. & Mcbride, M.J. (2007). Flavobacterium johnsoniae spra is a cell surface protein involved in gliding motility. Journal of Bacteriology, 189, 7145. doi: 10.1128/JB.00892-07
- Nikaido, H. (2003). Molecular basis of bacterial outer membrane permeability revisited. Microbiology and Molecular Biology Reviews, 67, 593–656. doi: 10.1128/MMBR.67.4.593-656.2003
- Pancholi, V., Boël, G. & Jin, H. (2010). Streptococcus pyogenes Ser/Thr kinase-regulated cell wall hydrolase is a cell division plane-recognizing and chain-forming virulence factor. Journal of Biological Chemistry, 285, 30861–30874. doi: 10.1074/jbc.M110.153825
- Pathanasophon, P., Sawada, T., Pramoolsinsap, T. & Tanticharoenyos, T. (1996). Immunogenicity of Riemerella anatipestifer broth culture bacterin and cell-free culture filtrate in ducks. Avian Pathology, 25, 705–719. doi: 10.1080/03079459608419176
- Rosadini, C.V., Ram, S. & Akerley, B.J. (2014). Outer membrane protein P5 is required for resistance of nontypeable Haemophilus influenzae to both the classical and alternative complement pathways. Infection and Immunity, 82, 640–649. doi: 10.1128/IAI.01224-13
- Saiki, K. & Konishi, K. (2010). The role of Sov protein in the secretion of gingipain protease virulence factors of Porphyromonas gingivalis. FEMS Microbiology Letters, 302, 166–174. doi: 10.1111/j.1574-6968.2009.01848.x
- Sandhu, T.S. (2008). Riemerella anatipestifer infection. Diseases of poultry (12th ed.). Oxford: Blackwell Publishing Ltd. pp. 758–764.
- Sato, K. (2011). Por secretion system of Porphyromonas gingivalis. Journal of Oral Biosciences, 53, 187–196. doi: 10.1016/S1349-0079(11)80001-0
- Sato, K., Naito, M., Yukitake, H., Hirakawa, H., Shoji, M., McBride, M.J., Rhodes, R.G. & Nakayama, K. (2010). A protein secretion system linked to bacteroidete gliding motility and pathogenesis. Proceedings of the National Academy of Sciences of the United States of America, 107, 276–281. doi: 10.1073/pnas.0912010107
- Segers, P., Mannheim, W., Vancanneyt, M., De Brandt, K., Hinz, K.H., Kersters, K. & Vandamme, P. (1993). Riemerella anatipestifer gen. nov., comb. nov., the causative agent of septicemia anserum exsudativa, and its phylogenetic affiliation within the Flavobacterium-Cytophaga rRNA homology group. International Journal of Systematic Bacteriology, 43, 768–776. doi: 10.1099/00207713-43-4-768
- Shrivastava, A., Johnston, J.J., van Baaren, J.M. & McBride, M.J. (2013). Flavobacterium johnsoniae GldK, GldL, GldM, and SprA are required for secretion of the cell surface gliding motility adhesins SprB and RemA. Journal of Bacteriology, 195, 3201–3212. doi: 10.1128/JB.00333-13
- Sifri, C.D., Mylonakis, E., Singh, K.V., Qin, X., Garsin, D.A., Murray, B.E., Ausubel, F.M. & Calderwood, S.B. (2002). Virulence effect of Enterococcus faecalis protease genes and the quorum-sensing locus fsr in Caenorhabditis elegans and mice. Infection and Immunity, 70, 5647–5650. doi: 10.1128/IAI.70.10.5647-5650.2002
- Somarajan, S.R., La Rosa, S.L., Singh, K.V., Roh, J.H., Höök, M. & Murray, B.E. (2015). The fibronectin-binding protein Fnm contributes to adherence to extracellular matrix components and virulence of Enterococcus faecium. Infection and Immunity, 83, 4653–4661. doi: 10.1128/IAI.00885-15
- Subramaniam, S., Chua, K.L., Tan, H.M., Loh, H., Kuhnert, P. & Frey, J. (1997). Phylogenetic position of Riemerella anatipestifer based on 16S rRNA gene sequences. International Journal of Systematic Bacteriology, 47, 562–565. doi: 10.1099/00207713-47-2-562
- Thein, M., Sauer, G., Paramasivam, N., Grin, I. & Linke, D. (2010). Efficient subfractionation of Gram-negative bacteria for proteomics studies. Journal of Proteome Research, 9, 6135–6147. doi: 10.1021/pr1002438
- Thomas, R., Hamat, R.A. & Neela, V. (2014). Extracellular enzyme profiling of Stenotrophomonas maltophilia clinical isolates. Virulence, 5, 326–330. doi: 10.4161/viru.27724
- Wang, X.J., Liu, W.B., Zhu, D.K., Yang, L.F., Liu, M.F., Yin, S.J., Wang, M.S., Jia, R.Y., Chen, S., Sun, K.F., Cheng, A.C. & Chen, X.Y. (2014). Comparative genomics of Riemerella anatipestifer reveals genetic diversity. BMC Genomics, 15, 479. doi: 10.1186/1471-2164-15-479
- Wisniewski, J.R., Zougman, A., Nagaraj, N. & Mann, M. (2009). Universal sample preparation method for proteome analysis. Nature Methods, 6, 359–362. doi: 10.1038/nmeth.1322
- Yu, C.S., Chen, Y.C., Lu, C.H. & Hwang, J.K. (2006). Prediction of protein subcellular localization. Proteins, 64, 643–651. doi: 10.1002/prot.21018
- Zhong, C.Y., Cheng, A.C., Wang, M.S., Zhu, D.K., Luo, Q.H., Zhong, C.D., Li, L. & Duan, Z. (2009). Antibiotic susceptibility of Riemerella anatipestifer field isolates. Avian Diseases, 53, 601–607. doi: 10.1637/8552-120408-ResNote.1
- Zhou, Z.T., Li, X.W., Xiao, Y.C., Wang, X.L., Tian, W.X., Peng, X.Y., Bi, D.R., Sun, M. & Li, Z.L. (2013). Gene expression responses to Riemerella anatipestifer infection in the liver of duck. Avian Pathology, 42, 129–136. doi: 10.1080/03079457.2013.770127
- Zhou, Z.T., Peng, X.Y., Xiao, Y.C., Wang, X.L., Guo, Z.S., Zhu, L., Liu, M., Jin, H., Bi, D.R. & Li, Z.L. (2011). Genome sequence of poultry pathogen Riemerella anatipestifer strain RA-YM. Journal of Bacteriology, 193, 1284–1285. doi: 10.1128/JB.01445-10