ABSTRACT
Lead (Pb) is an environmental pollutant and can damage organisms. Selenium (Se) can alleviate Pb poisoning. The present study aimed to investigate the alleviative effect of Se on Pb-induced immune toxicity in chicken hearts. One-hundred-and-eighty Hy-line male chickens were randomly divided into four groups at 7 days of age. The control group was offered a standard commercial diet (SD) and drinking water (DW); the Se group was offered SD supplemented with sodium selenite (SeSD) and DW; the Pb + Se group was offered SeSD and DW supplemented with lead acetate (PbDW); and the Pb group was offered SD and PbDW. Relative mRNA expression of inducible nitric oxide synthase (iNOS), interleukins (IL-2, IL-4, IL-6, IL-12β, IL-17 and IFN-γ), and heat shock proteins (HSP27, HSP40, HSP60, HSP70, and HSP90) were determined by means of quantitative real-time PCR. Relative protein expression of iNOS, HSP60, HSP70, and HSP90 was assessed, as well as nitric oxide (NO) content and iNOS activity in heart tissue. The results indicated a down-regulation of interleukin (IL)-2 and IFN-γ and an up-regulation of NO, iNOS, interleukins (IL-4, IL-6, IL-12β, IL-17), and heat shock proteins (HSP27, HSP40, HSP60, HSP70, and HSP90) in Pb-damaged hearts. Se alleviated all of the above Pb-induced changes. There were time-dependent effects on NO content, iNOS activity, and mRNA levels of iNOS, IL-2, IL-4, IL-6, IL-17, HSP27, HSP40, HSP60, HSP70, and HSP90 after Pb treatment in the chicken hearts. Se alleviated Pb-induced immune toxicity in the chicken hearts.
Introduction
Lead (Pb) is an environmental pollutant. Production industries, such as ore mining and refining, can cause Pb pollution. Pb pollution can damage humans and wild birds, and even threaten endangered birds and biodiversity. Pb pollution can increase blood Pb level in humans and cause adverse effects on human health (Zhang et al., Citation2016). Eum et al. (Citation2011) found that low-level exposure to Pb caused worse cardiac conductivity in human ventricles. Moreover, millions of wild birds died annually from Pb pollution worldwide (De Francisco et al., Citation2003). Pb pollution placed California condors (Gymnogyps californianus), one of the world’s rarest birds, at the edge of extinction from 1997 to 2010 (Finkelstein et al., Citation2012). Our previous study found that chicken heart was a target organ of Pb poisoning (Liu et al., Citation2017).
It has been reported that Pb toxicity can increase nitric oxide (NO) and inducible nitric oxide synthase (iNOS) in chicken livers (Wang et al., Citation2016) and testes (Wang et al., Citation2017). Interleukin (IL)-17 can trigger iNOS expression (Miljkovic & Trajkovic, Citation2004). Hydrogen sulphide treatment increases the mRNA level of IL-4 and decreases the mRNA level of IFN-γ in chicken jejunum (Zheng et al., Citation2019). Cadmium (Cd) decreased IL-2 and interferon-γ (IFN-γ) mRNA expression and caused immune toxicity in chicken splenic lymphocytes (Xu et al., Citation2015). Excess Pb inhibited IL-2 and IFN-γ mRNA expression, induced IL-4, IL-6, IL-12β, and IL-17 mRNA expression, and caused immune toxicity in chicken bursa of Fabricius (Jiao et al., Citation2017).
Under different stressors, heat shock proteins (HSPs) increase and protect against harmful factors. HSPs played a protective role in the bursa of Fabricius of chickens with selenium (Se) deficiency (Khoso et al., Citation2015). Pb poisoning increased HSP27, HSP40, HSP60, HSP70, and HSP90 mRNA expression in the livers (Wang et al., Citation2016) and cartilage tissues (Zheng et al., Citation2016) of chickens.
Se, an essential trace element (Zhang et al., Citation2017), can alleviate heavy metal-induced poisoning (Jin et al., Citation2018). Se alleviated histopathological damage by down-regulating the increases of NO content, iNOS activity, and iNOS mRNA expression induced by Cd in the cerebrums and cerebellums of chickens (Liu et al., Citation2014). Li et al. (Citation2017b) found that Se alleviated Pb poisoning by down-regulating iNOS protein expression in chicken neutrophils. Se can also alleviate immune toxicity via up-regulating IL-2 and IFN-γ mRNA expression in Cd-treated splenic lymphocytes (Xu et al., Citation2015) and Pb-treated bursa of Fabricius (Jiao et al., Citation2017) of chickens; and down-regulating IL-4, IL-6, IL-12β, and IL-17 mRNA expression in Pb-treated chicken bursa of Fabricius (Jiao et al., Citation2017). Wang et al. (Citation2016) reported that Se alleviated Pb-induced toxicity by down-regulating HSP27, HSP40, HSP60, HSP70, and HSP90 mRNA expression in chicken livers.
However, it is unclear whether NO, iNOS, cytokines (IL-2, IL-4, IL-6, IL-12β, IL-17, and IFN-γ), and HSPs (HSP27, HSP40, HSP60, HSP70, and HSP90) play a role in the alleviative effect of Se on Pb-induced immune toxicity in chicken hearts. Therefore, in this experiment, we designed an interaction model of Pb and Se in chickens to study the above factors and the possible mechanism of the alleviating effect of Se on Pb immune toxicity in chicken hearts.
Materials and methods
Animal model and tissue samples
In this study, all procedures were approved by the Institutional Animal Care and Use Committee of Northeast Agricultural University (approval number SRM-06). One-day-old Hy-line male chickens were offered a standard commercial diet (SD) (containing 0.49 mg/kg Se) and drinking water (DW). After one-week-long acclimatization period, 180 healthy birds were randomly divided into four groups (45 chickens in each group). The control group was offered SD and DW. The Se group was offered SD supplemented with sodium selenite (Na2SeO3·5H2O, analytical reagent grade, Tianjinzhiyuan Chemical Reagent Co., Ltd. Tianjin, China) (containing 1 mg/kg Se) (SeSD) and DW. The Pb group was offered SD and DW supplemented with lead acetate ((CH3OO)2Pb·3H2O, analytical reagent grade, Tianjinzhiyuan Chemical Reagent Co., Ltd. Tianjin, China) (containing 350 mg/L Pb) (PbDW) according to the median lethal dose (LD50) of Pb for chickens (Vengris & Maré, Citation1974) and the need for chicken experiments in toxicology (Klaassen & Watkins, Citation2013). The Pb + Se group was offered SeSD and PbDW. All chickens were allowed ad libitum access to feed and water. The chickens were housed in the Laboratory Animal Center, Animal Medical College, Northeast Agricultural University (Harbin, China).
Fifteen birds from each group were randomly sacrificed after being reared for 30, 60, and 90 days. Hearts were immediately excised and rinsed with ice-cold 0.9% NaCl solution. Each heart was divided into three parts. The first part was fixed with 5% paraformaldehyde solution for histopathology. The second part was homogenized to detect NO content and iNOS activity. The final part was immediately frozen in liquid nitrogen and stored at −80°C to detect mRNA expression of iNOS, cytokines, and HSPs; and protein expression of iNOS and HSPs.
Histopathology
The haematoxylin-eosin staining method was performed as described previously (Shi et al., Citation2019). Then, histologic sections were observed using an optical microscope (Eclipse 80i, Nikon, Tokyo, Japan) and photographs were taken.
NO content and iNOS activity
Detection kits produced by Nanjing Jiancheng Bioengineering Institute (Nanjing, China) were used to detect NO content and iNOS activity following the instructions of the reagent company.
Relative mRNA expression of iNOS, cytokines, and HSPs
Specific primers () for iNOS, cytokines (IL-2, IL-4, IL-6, IL-12β, IL-17, and IFN-γ), HSPs (HSP27, HSP40, HSP60, HSP70, and HSP90), and β-actin, published in GenBank, were synthesized by Sangon Biotech Co., Ltd. (Shanghai, China). β-actin was used as an internal reference gene.
Table 1. Gene specific primers used in quantitative real-time PCR.
Total RNA was extracted from heart tissues using RNAiso Plus (Takara, Japan) following the manufacturer’s instructions. The purity of total mRNA met the experimental requirements according to OD260/OD280 using a GeneQuant 1300/100 spectrophotometer (Healthcare Bio-Sciences AB, Sweden). Complementary DNA (cDNA) was synthesized with reverse transcription system (HaiGene, Harbin, China) following the manufacturer’s instructions. The synthesized cDNA was diluted fivefold with sterile water and then stored at −20°C for quantitative real-time PCR.
Quantitative real-time PCR was performed using a LightCycler® 96 System (Roche, Switzerland) following the instructions of FastStart Universal SYBR Green Master Mix (ROX) (Roche, Switzerland). Each sample was measured three times. The melting curve analysis showed only one peak for each PCR product. Relative mRNA expression was calculated according to the method of Pfaffl (Citation2001).
Relative protein expression of iNOS, HSP60, HSP70, and HSP90
Total protein was extracted from heart tissues with cell lysis buffer. The concentration of total protein was measured using a GeneQuant 1300/100 spectrophotometer (Healthcare Bio-Sciences AB, Sweden) according to Bradford’s method (Bradford, Citation1976). Total protein was separated using 15% sodium dodecyl sulphate-polyacrylamide gel electrophoresis. The separated proteins were transferred onto nitrocellulose membranes (Biotrace, Randburg, S.A.) in Tris-glycine buffer containing 20% methanol at 200 mA for 2 h. The membranes were blocked with 5% skimmed milk at 4°C overnight and incubated with corresponding primary rabbit antibody against iNOS (1:200, Abcam, Cambridge, MA, USA), and HSP60, HSP70, and HSP90 (1:1000, provided by Dr. Xu, College of Veterinary Medicine, Northeast Agricultural University) for 1 h. Then the membranes were incubated with horseradish peroxidase (HRP)-conjugated secondary antibody against rabbit IgG (1:1500, Santa Cruz Biotechnology, Santa Cruz, CA, USA) for 1 h (Wang et al., Citation2018). β-actin was used as an internal reference. The membrane was incubated with a monoclonal β-actin antibody (1:1000, Santa Cruz Biotechnology, Santa Cruz, CA, USA) for 1 h and then incubated with a HRP-conjugated goat anti-mouse IgG (1:1000, Santa Cruz Biotechnology, Santa Cruz, CA, USA) for 1 h. The signals were detected using chemiluminescence imaging system (ChemiScope5300 Pro, Clinx Science Instruments, Shanghai, China).
Statistical analysis
All experimental data were analysed in one-way (for protein expression) and two-way (for kit detection and mRNA expression) analyses of variance using SPSS for Windows (Version 19.0; SPSS Inc., Chicago, IL, USA). The statistical comparisons of results were verified by nonparametric Kruskal–Wallis ANOVA and Mann-Whitney U tests. The results obtained were expressed as the mean ± standard deviation. Bars with different upper-case letters were significantly different (P < 0.05) in the same group among different time points. Bars with different lower-case letters were significantly different (P < 0.05) among different groups at the same time point.
Results
Histopathology
Histological findings are shown in . Heart sections of the control group displayed normal structure with regular arrangement of cardiac muscle fibres at the end of the experiment (90th day). Histopathological cardiac lesions such as cardiac muscle fibre disarrangement, focal necrotic areas, and inflammatory cell infiltration were observed in all animals of the Pb group and also in the animals of the Pb + Se group on 90th day. In the Pb group, lesions increased in intensity over time, being more intense at the end of the experiment (90th day). Compared with the Pb group on the 90th day, histopathological lesions of the Pb + Se group were of lower intensity.
Figure 1. Histopathology (haematoxylin-eosin staining, ×400) of chicken hearts. (a) The control group on the 90th day. (b) The Pb group on the 30th day. (c) The Pb group on the 60th day. (d) The Pb group on the 90th day. (e) The Pb + Se group on the 90th day. (cmf: cardiac muscle fibres, v: vacuole, fn: focal necrosis, ici: inflammatory cell infiltration).
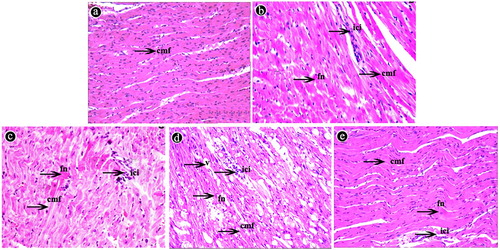
NO content, iNOS activity, and iNOS mRNA and protein expression
We determined NO content, iNOS activity, and iNOS mRNA expression on the 30th, 60th, and 90th days, and iNOS protein expression on the 90th day, as shown in . All results were significantly different (P < 0.05) among all groups except between the control group and the Se group (P > 0.05). All results in the Pb group were the highest, followed by the Pb + Se group, and the control and Se groups at all time points. For the Pb group, NO content, iNOS activity, and iNOS mRNA expression increased significantly (P < 0.05) with the increase of Pb-treatment time.
Relative mRNA expression of cytokines
To study the alleviative effect of Se on immune toxicity induced by Pb, mRNA expression of IL-2, IL-4, IL-6, IL-12β, IL-17, and IFN-γ was detected in chicken hearts. As shown in , there were significant differences (P < 0.05) in IL-2, IL-4, IL-6, IL-12β, IL-17, and IFN-γ mRNA expression among all groups except between the control group and the Se group (P > 0.05) on the 30th, 60th, and 90th days. IL-2 and IFN-γ mRNA expression in the control and Se groups was the highest, followed by the Pb + Se group, and the Pb group at all time points. IL-4, IL-6, IL-12β, and IL-17 mRNA expression was opposite to that of the above two factors. Relative mRNA expression of the four interleukins in the control and Se groups was significantly lower (P < 0.05) than that in the Pb + Se and Pb groups. Relative mRNA expression of the four interleukins in the Pb + Se group was significantly lower (P < 0.05) than that in the Pb group. For the Pb group, with the increase of Pb-treatment time, IL-2 mRNA expression decreased significantly (P < 0.05); and IL-4, IL-6, and IL-17 mRNA expression increased significantly (P < 0.05). IL-12β mRNA expression on the 90th day was significantly higher (P < 0.05) than that on the 30th and 60th days. IFN-γ mRNA expression on the 60th and 90th days was significantly lower (P < 0.05) than that on the 30th day.
Relative mRNA and protein expression of HSPs
Relative mRNA expression of HSPs (HSP27, HSP40, HSP60, HSP70, and HSP90) on the 30th, 60th, and 90th days, and protein expression of HSPs (HSP60, HSP70, and HSP90) on the 90th day, are shown in . We found that there were no significant differences (P > 0.05) in the expression of any of the detected HSPs at the three time points between the control group and the Se group except for HSP70 protein expression in the Se group that was significantly lower (P < 0.05) than that in the control group. Relative mRNA expression of all the detected HSPs throughout the experiment, and protein expression of HSP60 and HSP70 in the Pb + Se group, increased significantly (P < 0.05) compared with the control and Se groups except for HSP90 protein expression. The expression of all the detected factors in the Pb group was significantly higher (P < 0.05) than those in the other three groups. In the Pb group, HSP27, HSP40, HSP70, and HSP90 mRNA expression increased significantly (P < 0.05) with the increase of Pb-treatment time. HSP60 mRNA expression on the 60th and 90th days was significantly higher (P < 0.05) than that on the 30th day in the Pb group.
Discussion
Our previous study found that focal necrosis, inflammatory cell infiltration, and inflammatory injury occurred in chicken hearts after Pb treatment (Liu et al., Citation2017). It has been described that neuroinflammation and neurodegeneration were tightly linked to overproduction of NO by iNOS (Pannu & Singh, Citation2006). We found that excess Pb increased NO content and iNOS activity, as well as iNOS mRNA and protein expression in the chicken hearts. Therefore, the results implied that excess Pb damaged chicken hearts. Other researchers also found that excess heavy metals can induce NO and iNOS, and damage animals. For instance, Cd poisoning induced NO content, iNOS activity, and iNOS mRNA and protein expression, and caused injury in chicken kidneys (Liu et al., Citation2015). Copper (Cu) treatment increased iNOS mRNA expression and caused inflammatory injury in chicken hearts (Li et al., Citation2017a). NO content, iNOS activity, and iNOS mRNA expression increased after Pb treatment, and inflammatory response occurred in chicken testes (Wang et al., Citation2017). In addition, we found that Pb had time-dependent effects on NO content, iNOS activity, and iNOS mRNA expression in the chicken hearts. Wang et al. (Citation2016) also found that there were time-dependent effects on Pb-induced increases of NO content and iNOS mRNA expression in chicken livers.
Pb can affect the immune response in humans and animals. Early inflammation induces the activation and infiltration of immune cells from the blood, and initiates an immune response through pro-inflammatory factors (such as iNOS) and cytokines (such as IL-6) (Venkataramana et al., Citation2015). Type 1 T helper (Th1) cells mediate cellular immunity (Dong & Flavell, Citation2001). Type 2 T helper (Th2) cells instruct humoral immunity (Dong & Flavell, Citation2001). IL-4 inhibits Th1 cell activity, and IFN-γ inhibits Th2 cell activity (Elenkov et al., Citation2005). Th2 cells produce IL-4 and IL-6 (Romagnani, Citation2000). In mice, IL-4 and IFN-γ are markers of the cellular and humoral immune response (Radbin et al., Citation2014). IL-4 can promote IL-12β in human myeloid dendritic cells (DCs) and mouse bone-marrow derived DCs after treatment with lipopolysaccharide (Guenova et al., Citation2015). IL-6 promotes the production of Th17 cells which can produce IL-17 (Jin and Dong, Citation2013). In human peripheral mononuclear blood cells, Pb down-regulated the IFN-γ level and inhibited the Th1 immune response; and up-regulated the IL-4 level and induced a Th2 immune response (Hemdan et al., Citation2005). Pb up-regulated the IL-4 mRNA level and induced a Th2-driven immune response in the spleens of male red deer (Rodríguez-Estival et al., Citation2013). IFN-γ level decreased and IL-6 level increased in murine marrow haematopoietic stem cells after treatment with Pb (Queiroz et al., Citation2011). Pb treatment during late-gestation increased IL-12β mRNA expression and caused immune toxicity in rat offspring splenocytes (Bunn et al., Citation2001). After treatment with Pb, IL-2 and IFN-γ mRNA expression decreased; IL-4, IL-6, and IL-17 mRNA expression increased; and immune damage occurred in the chicken bursa of Fabricius (Jiao et al., Citation2017). In our study, IL-2 and IFN-γ mRNA expression decreased; and IL-4, IL-6, IL-12β, and IL-17 mRNA expression increased in Pb-treated chicken hearts. Our results indicated that excess Pb inhibited the Th1 immune response, induced a Th2 immune response, and caused immunotoxicity in the chicken hearts. In addition, we also found that there were time-dependent effects on the decreases of IL-2 and IFN-γ mRNA expression and the increases of IL-4, IL-6, and IL-17 mRNA expression in Pb-treated chicken hearts.
HSPs are the regulators of the immune response and have a protective effect against the toxicity caused by excess heavy metals (Pockley, Citation2003). Zhao et al. (Citation2017) found that the increases of mRNA expression of HSPs (HSP27, HSP40, HSP60, HSP70, and HSP90) and protein expression of HSPs (HSP60 and HSP70) were an important protective mechanism against arsenic-induced neurotoxicity in chicken brains. Excess Cu induced HSP70 protein expression in rainbow trout hepatocytes, and the increase of HSP70 may be crucial for protecting liver cells against Cu toxicity (Feng et al., Citation2003). High mRNA expression of HSP60, HSP70, and HSP90 may play a role in the resistance of duck spleen toxicity induced by molybdenum or/and Cd (Cao et al., Citation2016). Our study found that excess Pb induced mRNA expression of HSP27, HSP40, HSP60, HSP70, and HSP90; and protein expression of HSP60, HSP70, and HSP90 in the chicken hearts. It might be that HSPs played a protective role against Pb-induced immune toxicity in the chicken hearts. We also found that there were time-dependent effects on HSP27, HSP40, HSP60, HSP70, and HSP90 mRNA expression in Pb-induced chicken hearts. Choi et al. (Citation2008) reported that Cd increased HSP90 mRNA expression in a time-dependent effect in Pacific oyster gills and digestive glands.
Se can protect against poisoning caused by excess heavy metals. Se can alleviate immune damage caused by Pb in chicken bursa of Fabricius (Jiao et al., Citation2017). Se relieved Pb-induced injury in chicken kidneys (Jin et al., Citation2017) and hearts (Liu et al., Citation2017). In chicken splenic lymphocytes, Se alleviated Cd-induced increases of NO content, iNOS activity, and iNOS mRNA expression (Liu et al., Citation2015); and Cd-induced decreases of IL-2 and IFN-γ mRNA expression and immune toxicity (Xu et al., Citation2015). El-Boshy et al. (Citation2015) reported that Se alleviated Cd-induced decreases of IFN-γ level and increases of IL-6 level, and alleviated Cd-induced immune suppression in rat livers and kidneys. Se alleviated the increases of HSP27, HSP40, HSP60, HSP70, and HSP90 mRNA expression induced by Pb and alleviated Pb toxicity in chicken livers (Wang et al., Citation2016), peripheral blood lymphocytes (Sun et al., Citation2016), and cartilage tissues (Zheng et al., Citation2016). In our study, we found that Se alleviated histological damage; the increases of NO content, iNOS activity, and iNOS mRNA and protein expression; the decreases of IL-2 and IFN-γ mRNA expression; the increases of IL-4, IL-6, IL-12β, IL-17, HSP27, HSP40, HSP60, HSP70, and HSP90 mRNA expression; and the increases of HSP60, HSP70, and HSP90 protein expression in the chicken hearts. Our data indicate that Se alleviates Pb-induced immune toxicity in the chicken hearts.
Conclusions
Pb induced heart damage; increased NO content, iNOS activity, and iNOS mRNA and protein expression; decreased IL-2 and IFN-γ mRNA expression; increased mRNA expression of IL-4, IL-6, IL-12β, IL-17, HSP27, HSP40, HSP60, HSP70, and HSP90 as well as protein expression of HSP60, HSP70, and HSP90 in the chicken hearts. Se alleviated all of the above Pb-induced changes in the chicken hearts. There were time-dependent effects on NO content; iNOS activity; and iNOS, IL-2, IL-4, IL-6, IL-17, HSP27, HSP40, HSP70, and HSP90 mRNA expression after Pb treatment in the chicken hearts. Se alleviated Pb-induced immune toxicity by regulating NO, iNOS, cytokines, and HSPs in the chicken hearts.
Disclosure statement
No potential conflict of interest was reported by the authors.
Additional information
Funding
References
- Bradford, M.M. (1976). A rapid and sensitive method for the quantitation of microgram quantities of protein utilizing the principle of protein-dye binding. Analytical Biochemistry, 72, 248–254. doi: 10.1016/0003-2697(76)90527-3
- Bunn, T.L., Parsons, P.J., Kao, E. & Dietert, R.R. (2001). Exposure to lead during critical windows of embryonic development: differential immunotoxic outcome based on stage of exposure and gender. Toxicological Sciences, 64, 57–66. doi: 10.1093/toxsci/64.1.57
- Cao, H.B, Zhang, M., Xia, B., Xiong, J., Zong, Y.B., Hu, G.L. & Zhang, C.Y. (2016). Effects of molybdenum or/and cadmium on mRNA expression levels of inflammatory cytokines and HSPs in duck spleens. Biological Trace Element Research, 170, 237–244. doi: 10.1007/s12011-015-0442-0
- Choi, Y.K., Jo, P.G. & Choi, C.Y. (2008). Cadmium affects the expression of heat shock protein 90 and metallothionein mRNA in the Pacific oyster, Crassostrea gigas. Comparative Biochemistry and Physiology Part C: Toxicology and Pharmacology, 147, 286–292.
- De Francisco, N., Ruiz Troya, J.D. & Agüera, E.I. (2003). Lead and lead toxicity in domestic and free living birds. Avian Pathology, 32, 3–13. doi: 10.1080/0307945021000070660
- Dong, C. & Flavell, R.A. (2001). Th1 and Th2 cells. Current Opinion in Hematology, 8, 47–51. doi: 10.1097/00062752-200101000-00009
- El-Boshy, M.E., Risha, E.F., Abdelhamid, F.M., Mubarak, M.S. & Hadda, T.B. (2015). Protective effects of selenium against cadmium induced hematological disturbances, immunosuppressive, oxidative stress and hepatorenal damage in rats. Journal of Trace Elements in Medicine and Biology, 29, 104–110. doi: 10.1016/j.jtemb.2014.05.009
- Elenkov, I.J., Iezzoni, D.G., Daly, A., Harris, A.G. & Chrousos, G.P. (2005). Cytokine dysregulation, inflammation and well-being. Neuroimmunomodulation, 12, 255–269. doi: 10.1159/000087104
- Eum, K.D., Nie, L.H., Schwartz, J., Vokonas, P.S., Sparrow, D., Hu, H. & Weisskopf, M.G. (2011). Prospective cohort study of lead exposure and electrocardiographic conduction disturbances in the Department of Veterans Affairs Normative Aging Study. Environmental Health Perspectives, 119, 940–944. doi: 10.1289/ehp.1003279
- Feng, Q., Boone, A.N. & Vijayan, M.M. (2003). Copper impact on heat shock protein 70 expression and apoptosis in rainbow trout hepatocytes. Comparative Biochemistry and Physiology – Part C: Toxicology and Pharmacology, 135C, 345–355.
- Finkelstein, M.E., Doak, D.F., George, D., Burnett, J., Brandt, J., Church, M., Grantham, J. & Smith, D.R. (2012). Lead poisoning and the deceptive recovery of the critically endangered California condor. Proceedings of the National Academy of Sciences of the Unites States of America, 109, 11449–11454. doi: 10.1073/pnas.1203141109
- Guenova, E., Skabytska, Y., Hoetzenecker, W., Weindl, G., Sauer, K., Tham, M., Kim, K.W., Park, J H., Seo, J.H., Ignatova, D., Cozzio, A., Levesque, M.P., Volz, T., Köberle, M., Kaesler, S., Thomas, P., Mailhammer, R., Ghoreschi, K., Schäkel, K., Amarov, B., Eichner, M., Schaller, M., Clark, R.A., Röcken, M. & Biedermann, T. (2015). IL-4 abrogates TH17 cell-mediated inflammation by selective silencing of IL-23 in antigen-presenting cells. Proceedings of the National Academy of Sciences of the Unites States of America, 112, 2163–2168. doi: 10.1073/pnas.1416922112
- Hemdan, N.Y.A., Emmrich, F., Adham, K., Wichmann, G., Lehmann, I., El-Massry, A., Ghoneim, H., Lehmann, J. & Sack, U. (2005). Dose-dependent modulation of the in vitro cytokine production of human immune competent cells by lead salts. Toxicological Sciences, 86, 75–83. doi: 10.1093/toxsci/kfi177
- Jiao, X.Y., Yang, K., Teng, X.J. & Teng, X.H. (2017). Alleviation of lead-induced oxidative stress and immune damage by selenium in chicken bursa of Fabricius. Environmental Science and Pollution Research International, 24, 7555–7564. doi: 10.1007/s11356-016-8329-y
- Jin, W. & Dong, C. (2013). IL-17 cytokines in immunity and inflammation. Emerging Microbes & Infections, 2, e60, 1–5. doi: 10.1038/emi.2013.58
- Jin, X., Xu, Z., Zhao, X., Chen, M.H. & Xu, S.W. (2017). The antagonistic effect of selenium on lead-induced apoptosis via mitochondrial dynamics pathway in the chicken kidney. Chemosphere, 180, 259–266. doi: 10.1016/j.chemosphere.2017.03.130
- Jin, X., Jia, T., Liu, R. & Xu, S.W. (2018). The antagonistic effect of selenium on cadmium-induced apoptosis via PPAR-γ/PI3 K/Akt pathway in chicken pancreas. Journal of Hazardous Materials, 357, 355–362. doi: 10.1016/j.jhazmat.2018.06.003
- Khoso, P.A., Yang, Z., Liu, C. & Li, S. (2015). Selenoproteins and heat shock proteins play important roles in immunosuppression in the bursa of Fabricius of chickens with selenium deficiency. Cell Stress & Chaperones, 20, 967–978. doi: 10.1007/s12192-015-0625-9
- Klaassen, C.D. & Watkins, J.B. (2013). Casarett and Doull's Toxicology: The Basic Science of Poisons. New York: Mc Graw-Hill (63, p. 104).
- Li, S., Zhao, H., Wang, Y., Shao, Y., Li, J., Liu, J. & Xing, M. (2017a). The inflammatory responses in Cu-mediated elemental imbalance is associated with mitochondrial fission and intrinsic apoptosis in Gallus gallus heart. Chemosphere, 189, 489–497. doi: 10.1016/j.chemosphere.2017.09.099
- Li, X., Xing, M., Chen, M., Zhao, J., Fan, R., Zhao, X., Cao, C., Yang, J., Zhang, Z. & Xu S. (2017b). Effects of selenium-lead interaction on the gene expression of inflammatory factors and selenoproteins in chicken neutrophils. Ecotoxicology and Environmental Safety, 139, 447–453. doi: 10.1016/j.ecoenv.2017.02.017
- Liu, Y.Y., Jiao, X.Y., Teng, X.J., Gu, X.H. & Teng, X.H. (2017). Antagonistic effect of selenium on lead-induced nflammatory injury through inhibiting the nuclear actor-kB signaling pathway and stimulating slenoproteins in chicken hearts. RSC Advances, 7, 24878–24884.
- Liu, L.L., Yang, B.Y., Cheng, Y.P. & Lin, H.J. (2015). Ameliorative effects of selenium on cadmium-induced oxidative stress and endoplasmic reticulum stress in the chicken kidney. Biological Trace Element Research, 167, 308–319. doi: 10.1007/s12011-015-0314-7
- Liu, L.L., Li, C.M., Zhang, Z.W., Zhang, J.L., Yao, H.D. & Xu, S.W. (2014). Protective effects of selenium on cadmium-induced brain damage in chickens. Biological Trace Element Research, 158, 176–185. doi: 10.1007/s12011-014-9919-5
- Miljkovic, D. & Trajkovic, V. (2004). Inducible nitric oxide synthase activation by interleukin-17. Cytokine & Growth Factor Reviews, 15, 21–32. doi: 10.1016/j.cytogfr.2003.10.003
- Pannu, R. & Singh, I. (2006). Pharmacological strategies for the regulation of inducible nitric oxide synthase: neurodegenerative versus neuroprotective mechanisms. Neurochemistry International, 49, 170–182. doi: 10.1016/j.neuint.2006.04.010
- Pfaffl, M.W. (2001). A new mathematical model for relative quantification in real-time RT-PCR. Nucleic Acids Research, 29, e45, 2002–2007. doi: 10.1093/nar/29.9.e45
- Pockley, A.G. (2003). Heat shock proteins as regulators of the immune response. Lancet, 362, 469–476. doi: 10.1016/S0140-6736(03)14075-5
- Queiroz, M.L., da Rocha, M.C., Torello, C.O., de Souza Queiroz, J., Bincoletto, C., Morgano, M.A., Romano, M.R., Paredes-Gamero, E.J., Barbosa, C.M. & Calgarotto, A.K. (2011). Chlorella vulgaris restores bone marrow cellularity and cytokine production in lead-exposed mice. Food and Chemical Toxicology, 49, 2934–2941. doi: 10.1016/j.fct.2011.06.056
- Radbin, R., Vahedi, F. & Chamani, J.K. (2014). The influence of drinking-water pollution with heavy metal on the expression of IL-4 and INF-γ in mice by real-time polymerase chain reaction. Cytotechnology, 66, 769–777. doi: 10.1007/s10616-013-9626-7
- Rodríguez-Estival, J., De La Lastra, J.M., Ortiz-Santaliestra, M.E., Vidal, D. & Mateo, R. (2013). Expression of immunoregulatory genes and its relationship to lead exposure and lead-mediated oxidative stress in wild ungulates from an abandoned mining area. Environmental Toxicology and Chemistry, 32, 876–883. doi: 10.1002/etc.2134
- Romagnani, S. (2000). T-cell subsets (Th1 versus Th2). Annals of Allergy, Asthma & Immunology, 85, 9–18. doi: 10.1016/S1081-1206(10)62426-X
- Shi, Q.X., Jin, X., Fan, R.F., Xing, M.Y., Guo, J.M., Zhang, Z.W., Zhang, J.M. & Xu, S.W. (2019). Cadmium-mediated miR-30a-GRP78 leads to JNK-dependent autophagy in chicken kidney. Chemosphere, 215, 710–715. doi: 10.1016/j.chemosphere.2018.10.019
- Sun, G.X., Chen, Y., Liu, C.P., Li, S. & Fu, J. (2016). Effect of selenium against lead-induced damage on the gene expression of heat shock proteins and inflammatory cytokines in peripheral blood lymphocytes of chickens. Biological Trace Element Research, 172, 474–480. doi: 10.1007/s12011-015-0602-2
- Vengris, V.E. & Maré, C.J. (1974). Lead poisoning in chickens and the effect of lead on interferon and antibody production. Canadian Journal of Comparative Medicine, 38, 328–335.
- Venkataramana, S., Lourenssen, S., Miller, K.G. & Blennerhassett, M.G. (2015). Early inflammatory damage to intestinal neurons occurs via inducible nitric oxide synthase. Neurobiology of Disease, 75, 40–52. doi: 10.1016/j.nbd.2014.12.014
- Wang, H., Li, S. & Teng, X.H. (2016). The antagonistic effect of selenium on lead-induced inflammatory factors and heat shock proteins mRNA expression in chicken livers. Biological Trace Element Research, 171, 437–444. doi: 10.1007/s12011-015-0532-z
- Wang, W., Chen, M., Jin, X., Li, X., Yang, Z., Lin, H. & Xu, S.W. (2018). H2S induces Th1/Th2 imbalance with triggered NF-kappaB pathway to exacerbate LPS-induce chicken pneumonia response. Chemosphere, 208, 241–246. doi: 10.1016/j.chemosphere.2018.05.152
- Wang, Y., Wang, K.X., Huang, H. & Teng, X.H. (2017). Alleviative effect of selenium on inflammatory damage caused by lead via inhibiting inflammatory factors and heat shock proteins in chicken testes. Environmental Science and Pollution Research International, 24, 13405–13413. doi: 10.1007/s11356-017-8785-z
- Xu, F., Liu, S. & Li, S. (2015). Effects of selenium and cadmium on changes in the gene expression of immune cytokines in chicken splenic lymphocytes. Biological Trace Element Research, 165, 214–221. doi: 10.1007/s12011-015-0254-2
- Zhang, Y., Huo, X., Cao, J.J., Yang, T., Xu, L. & Xu, X.J. (2016). Elevated lead levels and adverse effects on natural killer cells in children from an electronic waste recycling area. Environmental Pollution, 213, 143–150. doi: 10.1016/j.envpol.2016.02.004
- Zhang, Z., Liu, M., Guan, Z., Yang, J., Liu, Z. & Xu, S. (2017). Disbalance of calcium regulation-related genes in broiler hearts induced by selenium deficiency. Avian Pathology, 46, 265–271. doi: 10.1080/03079457.2016.1259528
- Zhao, P., Guo, Y., Zhang, W., Chai, H., Xing, H. & Xing, M. (2017). Neurotoxicity induced by arsenic in Gallus Gallus: regulation of oxidative stress and heat shock protein response. Chemosphere, 166, 238–245. doi: 10.1016/j.chemosphere.2016.09.060
- Zheng, S.F., Song, H.Y., Gao, H., Liu, C.P., Zhang, Z.Z. & Fu, J. (2016). The antagonistic effect of selenium on lead-induced inflammatory factors and heat shock protein mRNA level in chicken cartilage tissue. Biological Trace Element Research, 173, 177–184. doi: 10.1007/s12011-016-0630-6
- Zheng, S.F., Jin, X., Chen, M.H., Shi, Q.X., Zhang, H.F. & Xu, S.W. (2019). Hydrogen sulfide exposure induces jejunum injury via CYP450s/ROS pathway in broilers. Chemosphere, 214, 25–34. doi: 10.1016/j.chemosphere.2018.09.002