ABSTRACT
Infectious bursal disease virus (IBDV) is the causative agent of a highly contagious immunosuppressive disease affecting young chickens. The recently described “distinct IBDV” (dIBDV) genetic lineage encompasses a group of worldwide distributed strains that share conserved genetic characteristics in both genome segments making them unique within IBDV strains. Phenotypic characterization of these strains is scarce and limited to Asiatic and European strains collected more than 15 years ago. The present study aimed to assess the complete and comprehensive phenotypic characterization of a recently collected South American dIBDV strain (1/chicken/URY/1302/16). Genetic analyses of both partial genome segments confirmed that this strain belongs to the dIBDV genetic lineage and that it is not a reassortant. Antigenic analysis with monoclonal antibodies indicated that this strain has a particular antigenic profile, similar to that obtained in a dIBDV strain from Europe (80/GA), which differs from those previously found in the traditional classic, variant and very virulent strains. Chickens infected with the South American dIBDV strain showed subclinical infections but had a marked bursal atrophy. Further analysis using Newcastle disease virus-immunized chickens, previously infected with the South American and European dIBDV strains, demonstrated their severe immunosuppressive effect. These results indicate that dIBDV strains currently circulating in South America can severely impair the immune system of chickens, consequently affecting the local poultry industry. Our study provides new insights into the characteristics and variability of this global genetic lineage and is valuable to determine whether specific control measures are required for the dIBDV lineage.
Research Highlights
A South American strain of the dIBDV lineage was phenotypically characterized.
The strain produced subclinical infections with a marked bursal atrophy.
Infected chickens were severely immunosuppressed.
The dIBDV strains are antigenically divergent from other IBDV lineages.
Introduction
Infectious bursal disease (IBD) is a highly contagious viral infection that causes severe economic losses in the global poultry industry. Its aetiologic agent is infectious bursal disease virus (IBDV), which belongs to the genus Avibirnavirus within the family Birnaviridae (Dobos et al., Citation1979). The virus performs a lytic replication cycle in developing B lymphocytes of the bursa of Fabricius and other lymphoid and non-lymphoid tissues (Burkhardt & Müller, Citation1987; Abdul et al., Citation2013). The resulting B lymphocyte depletion affects the immune system of young chicks and leads to an increased susceptibility to other infectious diseases, and poor vaccine responses (Allan et al., Citation1972; Faragher et al., Citation1974; Rosenberger & Gelb, Citation1978).
IBDV has a non-enveloped icosahedral capsid containing a double-stranded RNA genome with two segments, designated A and B (Müller et al., Citation1979). Segment A (3.3 kbp) encodes a polyprotein that is self-cleaved to release the capsid protein VP2, the viral protease VP4, the ribonucleoprotein VP3 and four peptides necessary for viral entry and assembly (Sánchez & Rodriguez, Citation1999; Lejal et al., Citation2000; Da Costa et al., Citation2002; Chevalier et al., Citation2005; Luque et al., Citation2009). A 5ʹ-terminal overlapping open reading frame encodes the non-structural protein VP5 (Mundt et al., Citation1995). Segment B (2.9 kbp) encodes the RNA-dependent RNA polymerase VP1 (Spies et al., Citation1987).
Two viral serotypes (1 and 2) are currently known but only the serotype 1 causes disease in chickens (McFerran et al., Citation1980; Ismail et al., Citation1988). According to antigenic and pathogenic characteristics, serotype 1 was traditionally subdivided into classic, antigenic variant and very virulent strains. The classic strains were first detected in North America, causing high morbidity and mortality before specific vaccines were developed to partially control the disease (Cosgrove, Citation1962; Snedeker et al., Citation1967; Edgar & Cho, Citation1976). Classic attenuated strains cause less severe effects than typical “classic virulent” strains (Giambrone & Clay, Citation1986; Mazariegos et al., Citation1990), and have been used as live attenuated vaccines since the mid-1970s. Variants with antigenic changes in the neutralizing epitopes of VP2 were detected in North America in the early 1980s (Rosenberger et al., Citation1985). These antigenic variants were able to evade the maternal immunity induced by classic-type vaccines and produced subclinical infections with marked immunosuppression (Rosales et al., Citation1989; Sharma et al., Citation1989). Very virulent strains were first reported in Europe in the late 1980s and caused extremely severe clinical signs, gross and microscopic lesions, and immunosuppression with unusual high mortality (Chettle et al., Citation1989; van den Berg et al., Citation1991). The very virulent strains are antigenically similar to the classic strains, but they can break through high levels of maternally-derived antibodies (van den Berg et al., Citation1996).
IBDV strains can be also distinguished by the affinity profile of different panels of monoclonal antibodies (mAbs) and by sequence analysis of the partial viral genome (Snyder et al., Citation1992; Eterradossi et al., Citation1997; Le Nouën et al., Citation2005). Phylogenetic analysis using sequences corresponding to the hyper-variable region of VP2 (hvVP2 region) can be used to distinguish the genetic lineages corresponding to most antigenically significant strains and also identify new lineages that usually comprise less phenotypically characterized strains (Ignjatovic & Sapats, Citation2002; Hernández et al., Citation2015; Lupini et al., Citation2016). A recent comprehensive analysis proposed a new classification scheme of IBDV strains into genogroups, which is expected to improve the current somewhat confusing nomenclature (Michel & Jackwood, Citation2017; Jackwood et al., Citation2018). According to this new scheme, the classic, antigenic variant and very virulent strains correspond to Genogroup 1, Genogroup 2 and Genogroup 3, respectively.
The recently described “distinct IBDV” (dIBDV) lineage is a major lineage that is highly genetically divergent from traditional IBDV subtypes (Hernández et al., Citation2015). This corresponds to the Genogroup 4 according to the new proposed nomenclature. This lineage includes strains collected in the last four decades from several countries of South and North America, Asia and Europe (Shcherbakova et al., Citation1998; Kwon et al., Citation2000; Ikuta et al., Citation2001; Jackwood et al., Citation2001; Domanska et al., Citation2004; Jackwood & Sommer-Wagner, Citation2007; Ojkic et al., Citation2007; Jeon et al., Citation2009; Hernández et al., Citation2015; Vera et al., Citation2015; Michel & Jackwood, Citation2017; Yamazaki et al., Citation2017). In the past, dIBDV constituted the majority of IBDV strains identified in Brazil and Canada (Ikuta et al., Citation2001; Ojkic et al., Citation2007). More recent epidemiological reports have indicated that dIBDV strains circulate at high frequency in poultry farms from Argentina and Uruguay (Hernández et al., Citation2015; Vera et al., Citation2015).
Five Hungarian and Polish dIBDV strains collected from 1977–1981 were analysed using a panel of eight mAbs in an AC-ELISA assay and showed unique antigenic profiles (Domanska et al., Citation2004). These Polish strains, besides two dIBDV strains collected in 1994 and 2002 from Asia, caused subclinical infections with bursal atrophy and underlying immunosuppression (Minta et al., Citation1985a, Citation1985b; Jeon et al., Citation2009; Yamazaki et al., Citation2017). All these studies were performed with European and Asiatic strains collected many years ago.
The present study assesses a complete and comprehensive genetic and phenotypic characterization of a currently circulating dIBDV strain from South America in order to provide updated insights into the characteristics and variability of this global genetic lineage.
Materials and methods
Ethic statements
All bird experimental infections were conducted in agreement with national regulations on animal welfare and after approval of the protocols by the host laboratory ethical committee (permit number APAFIS#4945-20 16041316546318 v6, ethical Committee registered at the national level under no. C2EA-16).
Virus strains
The dIBDV strain 1/chicken/URY/1302/16 was collected in South America (Uruguay) in 2016 from an outbreak occurring in a single poultry farm. Bursae of 25-day-old broilers exhibiting mild respiratory clinical signs were obtained and stored at −80°C. The absence of other common viral pathogens (infectious bronchitis virus, chicken anaemia virus and avian reovirus) was confirmed by RT–PCR or RT-qPCR (Xie et al., Citation1997; Callison et al., Citation2006; Techera et al., Citation2019).
The dIBDV strain 80/GA was isolated in 1980 in Europe (Poland) and was included for comparative purposes. The genetic, antigenic and pathogenic characteristics of this strain, including its immunosuppressive effect, have been already published (Minta et al., Citation1985a, Citation1985b; Domanska et al., Citation2004).
Reference strains F52/70 (classic virulent), 89163 (very virulent) and 94432 (atypical very virulent) were used for inter-strain comparison and as controls (Bygrave & Faragher, Citation1970; Eterradossi et al., Citation1992; Eterradossi et al., Citation1998). NDV strain Hertz/33 was used for challenge in the immunosuppressive assay.
Genetic characterization of the South American dIBDV strain
A specific qPCR assay was used to identify the South American strain as belonging to the dIBDV lineage (Tomás et al., Citation2017). Further genetic characterization was carried out through partial VP2 and VP1 analyses. Total RNA was extracted directly from infected bursae using the Quick-RNATM MiniPrep kit (Zymo Research, Irvine, CA, USA). Reverse transcription was carried out using the RevertAidTM H Minus First Strand cDNA Synthesis kit (Thermo Scientific, Hanover, MD, USA) with random hexamer primers. A 492-bp fragment within the hvVP2 region and a 594-bp fragment corresponding to a partial sequence of the VP1 gene were amplified. All amplifications were carried out using an Arktik Thermal Cycler (Thermo Scientific) with previously published primers and cycling conditions (Hernández et al., Citation2006). PCR products were purified using the DNA Clean & ConcentratorTM-5 kit (Zymo Research) and submitted for bidirectional sequencing (Macrogen Inc, Seoul, South Korea). Sequences were assembled and edited with the Lasergene SeqMan Pro software 15.0.1 (DNASTAR). Two datasets corresponding to the partial VP2 and VP1 coding sequences were generated including the South American strain and representatives of other previously described genetic lineages. Sequence alignments were performed using the MUSCLE algorithm implemented in MEGA 7.0 software (Kumar et al., Citation2016). The hvVP2 alignment encompassed a 374-bp region of segment A, and the VP1 alignment corresponds to a 543-bp region of segment B. Best-fit nucleotide substitution models were selected under the Akaike information criterion using jModelTest2 (Guindon & Gascuel, Citation2003; Darriba et al., Citation2012). Maximum-likelihood trees, with an approximate likelihood ratio test (aLRT) for internal nodes support, were inferred using the PhyML 3.0 software (Guindon et al., Citation2010). Phylogenetic trees were visualized and edited with the TreeGraph2 software and the iTOL v4 online tool (Stöver & Müller, Citation2010; Letunic & Bork, Citation2016).
Virus isolation and titration
Bursae of Fabricius from birds infected with the South American strain were homogenized and treated with 1,1,2-trichlorotrifluoroethane for virus extraction as previously described (Eterradossi et al., Citation1992). The strain was reinoculated into five specific pathogen-free (SPF) chickens maintained in confined facilities (ANSES, Ploufragan, France). Bursae from the inoculated chickens were harvested 4 days post infection and used to prepare primary viral stocks.
Remaining strains were obtained from viral stocks maintained in facilities of the OIE reference laboratory Ploufragan, France. Virus titration was carried out using primary bursal cell cultures as previously described (Soubies et al., Citation2018) for the pathogenicity assay, and SPF embryonated chicken eggs for the immunosuppression assay. In both procedures, 10-fold serial dilutions were used as inoculum with eight replicates each. Nine- to eleven-day-old embryonated chicken eggs were used and inoculated by the chorio-allantoic membrane (CAM) route. Titration of NDV strain Herts/33 was performed as described for IBDV using intra-allantoic inoculation of 9-day-old embryonated chicken eggs. Titres were calculated by the Reed & Muench method and were expressed as tissue culture infectious dose 50% (TCID50) and embryo infectious dose 50% (EID50), respectively (Reed & Muench, Citation1938).
Antigenic characterization
The antigenic profile of the South American strain was determined by using a previously described antigen-capture ELISA (AC-ELISA) (Eterradossi et al., Citation1997). Briefly, IBDV antigens were captured in standardized amounts using an anti-IBDV chicken polyclonal serum and detected with a panel of eight IBDV-specific neutralizing mAbs. Binding of the mAbs to the virus particle is expressed as the percentage of the binding of a polyclonal reference reagent (percentage and binding are positively correlated). European dIBDV strain 80/GA was included in the analysis for comparative purposes. Strains F52/70 and 94432 were used as references for classic virulent and atypical very virulent antigenic profiles, respectively.
Characterization of acute pathogenicity
Acute pathogenicity assessment was performed using 6-week-old SPF White-Leghorn chickens (ANSES) maintained in biosafety level 2 isolators. On the first day, chickens were weighed and divided into three groups of comparable sex and weight. Chickens were identified by coloured rings on their legs. One group of five chickens was mock-inoculated by the intranasal route with sterile culture medium (uninfected controls) and housed in a positive-pressure filtered air isolator. Two other groups containing 13 and 15 chickens were inoculated with 106.3 TCID50 (South American isolate) or 108.1 TCID50 (very virulent 89163), respectively. Clinical signs were recorded daily for 10 days using a symptomatic index ranging from 0 (no clinical sign) to 3 (severely affected or dead bird) (Le Nouën et al., Citation2012). Five birds belonging to both virus-inoculated groups were euthanatized at 4 days post infection (dpi) to determine gross lesions and record body and bursa weights. The same procedure was performed at 21 dpi with the surviving birds from the infected and uninfected groups. Additionally, serum samples were taken at 21 dpi and screened for antibodies against a panel of avian viruses that included IBDV, avian influenza virus, infectious laryngotracheitis virus, avian adenovirus CELO, avian metapneumovirus, infectious bronchitis virus, chicken anaemia virus and avian reovirus as previously described (Soubies et al., Citation2017). Bursa to body weight (BBW) ratios were calculated with the formula BBW ratio = bursa weight (g)/body weight (g).
Evaluation of immunosuppression
Two groups of 13 one-day-old chicks were infected by the eye drop route with 102 EID50 of either South American or European dIBDV strains. Two other groups composed of 15 one-day-old chicks were maintained uninfected and used as unvaccinated and vaccinated controls. At day 15, all groups except the unvaccinated control group received one dose of Newcastle disease virus (NDV) live vaccine (Zoetis, Poulvac Hitchner B1) by the eye drop route. At day 30, blood samples were taken from all chicks for NDV serology using haemagglutination inhibition (HI) test. Additionally, three chicks per group were euthanized for necropsic examination. All remaining chicks were then injected intra-muscularly with 2 × 105 EID50 of the NDV virulent strain Herts/33. Clinical signs were followed and mortality was recorded daily for 15 days.
Statistical analyses
Statistical differences were evaluated using the R version 3.4.4 software (R Core Team, Citation2014). BBW ratios as well as HI log2 titres were compared using the unpaired t-test for two groups of data or the one-way ANOVA followed by Tukey’s test when more than two groups of data were compared. Mortality percentage differences were evaluated using Fisher’s exact test. Values of P < 0.05 were considered statistically significant.
Accession numbers
The new sequences corresponding to the hvVP2 region and the partial VP1 gene were submitted to the Genbank database with the accession numbers MH981945 and MH981946, respectively.
Results
Genetic characterization
The South American (1/chicken/URY/1302/16) and European (80/GA) strains form a dIBDV phylogenetic group (Genogroup 4) based on the hvVP2 nucleotide sequence together with other strains from South America, Europe and Asia (). The same clustering is observed with the partial sequence of VP1 region but fewer strains are analysed as segment B is less frequently studied. The remaining observed lineages correspond to classic virulent, classic attenuated, variant and very virulent strains.
Figure 1. Phylogenetic inferences deduced from nucleotide sequences corresponding to the partial hvVP2 region (left) and the partial VP1 gene (right). Main genetic lineages are indicated with shaded boxes. The names of the corresponding genogroups are indicated within brackets. Both trees were inferred using the maximum-likelihood method with the GTR + I+G nucleotide substitution model. Approximate likelihood ratio test support values are shown for relevant nodes. South American and European dIBDV strains analysed in this study are indicated with bold letters and black circles.
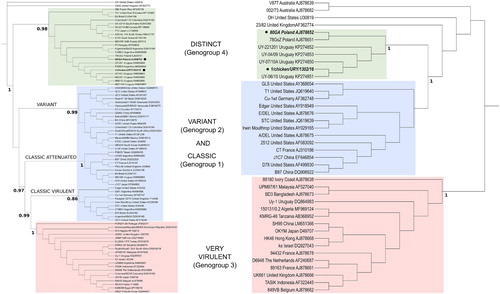
The amino acid sequences of the hvVP2 region of the South American and European dIBDV strains are highly conserved and have the signature T272, P289, I290 and F296. Additionally, they have a few common amino acid changes (e.g. similar substitutions at positions 222 and 254) and the only differentiating amino acid is at the position 299 (Supplementary material S1A).
The amino acid sequences of VP1 differ at residue 145 (Supplementary material S1B); European strains have N, which is also present in some classic virulent, classic attenuated and variant strains, whereas the South American dIBDV strains have S. Both dIBDV strains have the P243 VP1 marker.
Antigenic characterization
The classical virulent strain F52/70 had high reactivity with all eight mAbs tested, while the atypical very virulent strain 94432 lacked reactivity to mAbs 3 and 4, and had a reduced binding affinity with mAbs 5 and 6 (). South American and European dIBDV strains showed the same antigenic profile. They both lacked binding sites for mAbs 3, 4 and 5, and had a reduced binding affinity with mAbs 1 and 8.
Table 1. Antigenic characterization of the Uruguayan dIBDV strain by AC-ELISA. Light grey = reduced binding; black grey = lack of binding.
Characterization of acute pathogenicity
No mortality or clinical signs were observed in both uninfected control group and chickens infected with the South American dIBDV strain ((A,B)). The reference very virulent strain 89163 caused 46.7% mortality (7/15) and typical signs of acute infectious bursal disease, including ruffled feathers, diarrhoea and prostration, and had the highest symptomatic index value of 1.67 at 3 dpi.
Figure 2. Pathogenicity of the South American dIBDV strain compared to the European very virulent strain 89163. (A) Survival rate of 6-week-old SPF chickens infected intranasally with each strain or mock-inoculated, during a 10-day period post inoculation. (B) Mean symptomatic indexes of chickens recorded within 10 days post infection. (C) Bursa to body weight (BBW) ratios calculated 21 days post infection. Statistically significant differences (P < 0.05) between selected groups are indicated with different lowercase letters.
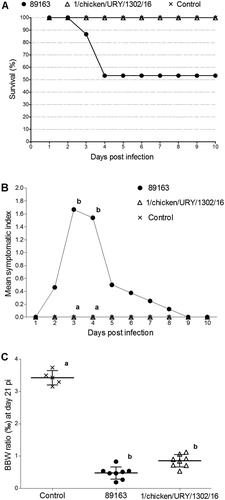
At 4 dpi, the mean BBW ratios of the very virulent and South American dIBDV strain were 3.76% and 2.45%, respectively; this difference was not statistically significant (P > 0.05). At 21 dpi, the uninfected control had the largest mean value (3.42%), while the very virulent and South American dIBDV strains had significantly lower values (0.48% and 0.85%, respectively) ((C)). Serological analyses assessed at the end of the experiment evidenced the presence of antibodies against IBDV and the lack of antibodies against seven other viral pathogens in the infected chickens (data not shown).
Evaluation of immunosuppression
The absence of clinical signs combined with the marked bursal atrophy at 21 dpi in chicks infected with the South American dIBDV strain prompted us to specifically evaluate the immunosppressive properties of this virus, in comparison with the European dIBDV strain 80/GA (Minta et al., Citation1985b). Immunization with NDV elicited high titres of neutralizing antibodies in the control group (mean value: 5.3 log2) in comparison with the unvaccinated group (mean titre: <2.0 log2) ((A)). These titres were significantly lower (P < 0.05) in groups inoculated with the South American (mean value: 2.5 log2) or the European virus (mean value: 2.6 log2), with only two out of 10 birds (South American strain) and five out of 10 birds (European strain) exhibiting an antibody response, respectively.
Figure 3. Immunosuppressive effect produced by the South American and European dIBDV strains. (A) HI mean titres observed 15 days after administration of the NDV vaccine in the vaccinated control group, European dIBDV strain (80/GA)-infected group and South American dIBDV strain (1/chicken/URY/1302/16)-infected group. Mean HI titres of unvaccinated control group were also measured. The dotted line indicates the positivity threshold. (B) Number of dead and alive chicks 15 days after infection with the NDV virulent strain Herts/33 for each group. Mortality percentages are shown. Statistically significant differences (P < 0.05) between selected groups are indicated with different lowercase letters.
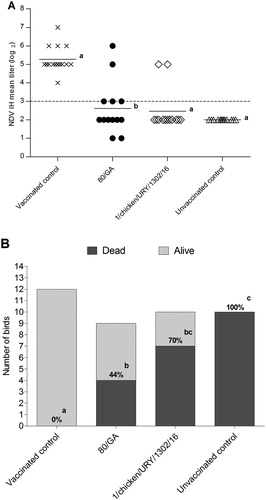
Birds belonging to the vaccinated control group survived the challenge with the NDV virulent strain Herts/33 and lacked any clinical sign of Newcastle disease ((B)). All unvaccinated birds succumbed to the challenge with the NDV virulent strain Herts/33. The cumulated mortalities of the dIBDV strains, 70% (7/10) for the South American strain and 44.4% (4/9) for the European strain, were statistically significantly different compared to the vaccinated control (P < 0.05). Collectively, these data show that the South American dIBDV strain 1/chicken/URY/1302/16 interfered with NDV vaccinal response and demonstrate the immunosuppressive potential of this IBDV strain.
Discussion
The emergence of classic, variant and very virulent strains are milestones in the evolutionary history of IBDV that were immediately detected by the severity of the clinical signs, mortality and histopathological lesions in the affected flocks (Cosgrove, Citation1962; Chettle et al., Citation1989; Snyder, Citation1990). Strain emergence was strongly linked with changes in the genome that provide genetic identity to the strains and lead to their classification in genogroups (shared genetic markers) or lineages (common evolutionary origin) (Jackwood et al., Citation2018). The characterization of lineages that diverge from traditional strains provides useful information to understand IBDV epidemiology and control. Although most novel lineages are spatially and temporally confined and should be more properly considered as events of local differentiation, the dIBDV lineage is widespread and has persisted for several decades (Domanska et al., Citation2004; Hernández et al., Citation2015). Here, we achieved a complete phenotypic characterization of a dIBDV strain, recently collected from South America, to get a comprehensive view of the behaviour and impact of current dIBDV isolates for the poultry industry. Importantly, several lines of evidence support the absence of contaminating virus in the initial field sample. This sample tested negative by RT–PCR and RT-qPCR for chicken anaemia virus, infectious bronchitis virus and avian reovirus. Furthermore, seroconversion of infected chickens at 21 dpi was observed for IBDV but not for seven other common avian viral pathogens.
The dIBDV strains from South America and Europe share the same genetic (, Supplementary material S1A and S1B) and antigenic characteristics (). The similar phylogenetic relationship inferred from the nucleotide sequences of hvVP2 and VP1 supports that dIBDV strains share similar evolutionary trends for both segments and are not reassortants. The correlation of amino acid sequences and mAb binding patterns evidences a direct link between specific residues and antigenic properties (Vakharia et al., Citation1994; Eterradossi et al., Citation1998; Letzel et al., Citation2007). The lack of reactivity with mAbs 3 and 4 is related to changes within the major hydrophilic peak A, particularly changes in residues P222 and G223 (Eterradossi et al., Citation1998). Thus, the Pro→Ser change in position 222 may explain the lack of binding of mAbs 3 and 4 in both dIBDV strains. The absence of reactivity with mAb 5 is usually observed when G254 is mutated, although reduced levels of binding occur in strains with an unaltered residue (Eterradossi et al., Citation2004; Martin et al., Citation2007; Soubies et al., Citation2017). The dIBDV strains analysed here have D254, which may account for the lack of binding of mAb 5. Reduced binding of mAb 1 has not been associated with any amino acid alteration in hvVP2. It is possible that residues outside the hvVP2 region additionally affect the antigenic behaviour (Domanska et al., Citation2004; Durairaj et al., Citation2011). MAb 8 exhibits reduced binding affinity when some mutations occur within Loop HI (major hydrophilic peak B); however, this is not the case for 1/chicken/URY/1302/16 and 80/GA strains (Eterradossi et al., Citation1998, Citation2004). The reduced binding affinity of the mAb 8 may also be caused by the Gly→Asp change at residue 254 due to its spatial proximity with the major hydrophilic peak B (Letzel et al., Citation2007). All these genetic and antigenic characteristics are consistent with those reported by Domanska et al. (Citation2004) with a Polish dIBDV isolate.
The comparative antigenic profiles assessed here for the dIBDV, classic virulent and very virulent strains, in addition to previously obtained results for other strains including antigenic variants (Eterradossi et al., Citation1998; Domanska et al., Citation2004), support that the dIBDV strains are antigenically different from traditional strains.
The genetic characteristics and phenotypic behaviour of the South American strain analysed here are similar to those observed in a strain collected in 1980 from another continent (Polish strain 80/GA); there is only one amino acid mutation between these two strains in the hvVP2 region analysed (at the position 299). Viral strains usually undergo different evolutionary processes driven by different forces, including vaccine pressures, which promotes the occurrence of neutral and selective mutations and concomitant phenotypic modifications (Boni, Citation2008; Chong & Ikematsu, Citation2017). Thus, the conservation of the dIBDV strains suggests that it has not been subjected to strong selective pressures or that it is unable to change its adaptive peak in response to evolutionary forces.
Chickens infected with the South American dIBDV strain showed subclinical infections but had a marked bursal atrophy ((A–C)). This is in agreement with previous reports assessing the pathogenicity of Asiatic and European dIBDV strains (Minta et al., Citation1985a; Jeon et al., Citation2009; Yamazaki et al., Citation2017). Thus, the absence of clinical signs seems to be an ancestral characteristic of dIBDV strains that remained unaltered over time, explaining the reduced and sporadic number of strains reported in normal clinical surveillance through the years.
Virus-induced bursal atrophy is typically associated with concomitant immunosuppression, and this was demonstrated for the dIBDV strains analysed here. The antibody levels induced by the NDV HD vaccine were reduced by around 85% and, consequently, there was a decreased protection against subsequent challenge with NDV virulent strain Herts/33 ((A,B)). The subclinical manifestation and induced immunosuppression are characteristics shared by the dIBDV and variant IBDV strains. However, genetic analyses based on both genome segments and antigenic analyses clearly show that the dIBDV and variant strains represent different genetic lineages with different antigenic profiles (Domanska et al., Citation2004; Hernández et al., Citation2015; Vera et al., Citation2015; Michel & Jackwood, Citation2017).
The economic impact of strains with subclinical manifestation should not be underestimated. A recent study quantified the economic losses generated by circulating strains in the period 2007–2011 in Saskatchewan province, Canada, where variant and dIBDV strains were prevalent (Zachar et al., Citation2016). The authors concluded that the Saskatchewan poultry industry is losing around 3.9 thousand tons of meat per year, approximately 10% of its annual production. Considering that Argentina and Brazil are among the most important poultry producers, accounting for 17% of total world broiler meat production in 2017, the impact of dIBDV in these countries might be very important and deserves a more thorough evaluation (USDA, Citation2018).
The protection against dIBDV strains elicited by currently used vaccines is not known. Current vaccines do not include dIBDV strains in their formulation, and may therefore have reduced protection. This is concordant with the frequent identification of dIBDV strains in Argentina and Uruguay despite continuous vaccination with classic vaccine strains, and in Canada despite the use of variant vaccine strains (Ojkic et al., Citation2007; Hernández et al., Citation2015; Vera et al., Citation2015). This hypothesis is reinforced by the recent report of poor cross-neutralization between the Japanese dIBDV strain TY2 and the classic virulent strain K (Yamazaki et al., Citation2017). In vivo protection assays should be done in the future to determine the level of protection of commonly used classic and variant vaccines with dIBDV strains.
In conclusion, the recent South American isolate belonging to the dIBDV genetic lineage has a particular antigenic profile, provokes a subclinical infection which results in a marked bursal atrophy, and exhibits significant immunosuppressive properties. The absence of clinical signs and mortality makes dIBDV strains phenotypically similar to variant strains. However, the genetic and antigenic divergence support that the dIBDV lineage has similar status to traditional IBDV strains and its emergence should be also considered a major evolutionary event in the IBDV history. More efforts are needed to detect and characterize dIBDV strains worldwide in order to provide new insights into their epidemiology and to determine whether specific tools are needed to control this IBDV lineage.
Supplemental Material
Download MS Word (218.1 KB)Disclosure statement
No potential conflict of interest was reported by the authors.
Additional information
Funding
References
- Abdul, R., Murgia, M., Rodriguez-Palacios, A., Lee, C. & Saif, Y. (2013). Persistence and tissue distribution of infectious bursal disease virus in experimentally infected SPF and commercial broiler chickens. Avian Diseases, 57, 759–766. doi: 10.1637/10448-110812-Reg.1
- Allan, W.H., Faragher, J.T. & Cullen, G.A. (1972). Immunosuppression by the infectious bursal agent in chickens immunised against Newcastle disease. The Veterinary Record, 90, 511–512. doi: 10.1136/vr.90.18.511
- Boni, M.F. (2008). Vaccination and antigenic drift in influenza. Vaccine, 26 (Suppl 3), C8–14. doi: 10.1016/j.vaccine.2008.04.011
- Burkhardt, E. & Müller, H. (1987). Susceptibility of chicken blood lymphoblasts and monocytes to infectious bursal disease virus (IBDV). Archives of Virology, 94, 297–303. doi: 10.1007/BF01310722
- Bygrave, A.C. & Faragher, J. (1970). Mortality associated and Gumboro disease. The Veterinary Record, 86, 758–759.
- Callison, S.A., Hilt D.A., Boynton T.O., Sample B.F., Robison R., Swayne D.E., & Jackwood M.W. (2006). Development and evaluation of a real-time Taqman RT-PCR assay for the detection of infectious bronchitis virus from infected chickens. Journal of Virological Methods, 138, 60–65. doi: 10.1016/j.jviromet.2006.07.018
- Chettle, N., Stuart, J.C. & Wyeth, P.J. (1989). Outbreak of virulent infectious bursal disease in East Anglia. The Veterinary Record, 125, 271–272. doi: 10.1136/vr.125.10.271
- Chevalier, C., Galloux, M., Pous, J., Henry, C., Denis, J., Da Costa, B., Navaza, J., Lepault, J. & Delmas, B. (2005). Structural peptides of a nonenveloped virus are involved in assembly and membrane translocation. Journal of Virology, 79, 12253–12263. doi: 10.1128/JVI.79.19.12253-12263.2005
- Chong, Y. & Ikematsu, H. (2017). Effect of seasonal vaccination on the selection of influenza A/H3N2 epidemic variants. Vaccine, 35, 255–263. doi: 10.1016/j.vaccine.2016.11.084
- Cosgrove, A.S. (1962). An apparently new disease of chickens: avian nephrosis. Avian Diseases, 6, 385–389. doi: 10.2307/1587909
- Da Costa, B., Chevalier, C., Henry, C., Huet, J.-C., Petit, S., Lepault, J., Boot, H. & Delmas, B. (2002). The capsid of infectious bursal disease virus contains several small peptides arising from the maturation process of pVP2. Journal of Virology, 76, 2393–2402. doi: 10.1128/jvi.76.5.2393-2402.2002
- Darriba, D., Taboada, G.L., Doallo, R. & Posada, D. (2012). Jmodeltest 2: more models, new heuristics and parallel computing. Nature Methods, 9, 772. doi: 10.1038/nmeth.2109
- Dobos, P., Hill, B.J., Hallett, R., Kells, D.T., Becht, H. & Teninges, D. (1979). Biophysical and biochemical characterization of five animal viruses with bisegmented double-stranded RNA genomes. Journal of Virology, 32, 593–605.
- Domanska, K., Mato, T., Rivallan, G., Smietanka, K., Minta, Z., de Boisseson, C., Toquin, D., Lomniczi, B., Palya, V. & Eterradossi, N. (2004). Antigenic and genetic diversity of early European isolates of infectious bursal disease virus prior to the emergence of the very virulent viruses: early European epidemiology of infectious bursal disease virus revisited? Archives of Virology, 149, 465–480. doi: 10.1007/s00705-003-0230-6
- Durairaj, V., Sellers, H.S., Linnemann, E.G. Icard, A.H. & Mundt, E. (2011). Investigation of the antigenic evolution of field isolates using the reverse genetics system of infectious bursal disease virus (IBDV). Archives of Virology, 156, 1717–1728. doi: 10.1007/s00705-011-1040-x
- Edgar, S.A. & Cho, Y. (1976). The epizootiology of infectious bursal disease and prevention of it by immunization. Developments in Biological Standardization, 33, 349–356.
- Eterradossi, N., Arnauld, C., Toquin, D. & Rivallan, G. (1998). Critical amino acid changes in VP2 variable domain are associated with typical and atypical antigenicity in very virulent infectious bursal disease viruses. Archives of Virology, 143, 1627–1636. doi: 10.1007/s007050050404
- Eterradossi, N., Gauthier, C., Reda, I., Comte, S., Rivallan, G., Toquin, D., de Boisséson, C., Lamandé, J., Jestin, V., Morin, Y., Cazaban, C. & Borne, P. (2004). Extensive antigenic changes in an atypical isolate of very virulent infectious bursal disease virus and experimental clinical control of this virus with an antigenically classical live vaccine. Avian Pathology, 33, 423–431. doi: 10.1080/03079450410001724049
- Eterradossi, N., Picault, J.P., Drouin, P., Guittet, M., L’Hospitalier, R. & Bennejean, G. (1992). Pathogenicity and preliminary antigenic characterization of six infectious bursal disease virus strains isolated in France from acute outbreaks. Journal of Veterinary Medicine. Series B, 39, 683–691. doi: 10.1111/j.1439-0450.1992.tb01222.x
- Eterradossi, N., Toquin, D., Rivallan, G. & Guittet, M. (1997). Modified activity of a VP2-located neutralizing epitope on various vaccine, pathogenic and hypervirulent strains of infectious bursal disease virus. Archives of Virology, 142, 255–270. doi: 10.1007/s007050050075
- Faragher, J.T., Allan, W.H. & Wyeth, P.J. (1974). Immunosuppressive effect of infectious bursal agent on vaccination against Newcastle disease. The Veterinary Record, 95, 385–388. doi: 10.1136/vr.95.17.385
- Giambrone, J.J. & Clay, R.P. (1986). Evaluation of the immunogenicity, stability, pathogenicity, and immunodepressive potential of four commercial live infectious bursal disease vaccines. Poultry Science, 65, 1287–1290. doi: 10.3382/ps.0651287
- Guindon, S., Dufayard, J.-F., Lefort, V., Anisimova, M., Hordijk, W. & Gascuel, O. (2010). New algorithms and methods to estimate maximum-likelihood phylogenies: assessing the performance of PhyML 3.0. Systematic Biology, 59, 307–321. doi: 10.1093/sysbio/syq010
- Guindon, S. & Gascuel, O. (2003). A simple, fast, and accurate algorithm to estimate large phylogenies by maximum likelihood. Systematic Biology, 52, 696–704. doi: 10.1080/10635150390235520
- Hernández, M., Banda, A., Hernández, D., Panzera, F. & Pérez, R. (2006). Detection of very virulent strains of infectious bursal disease virus (vvIBDV) in commercial broilers from Uruguay. Avian Diseases, 50, 624–631. doi: 10.1637/7530-032306R1.1
- Hernández, M., Tomás, G., Marandino, A., Iraola, G., Maya, L., Mattion, N., Hernández, D., Villegas, P., Banda, A., Panzera, Y. & Pérez, R. (2015). Genetic characterization of South American infectious bursal disease virus reveals the existence of a distinct worldwide-spread genetic lineage. Avian Pathology, 44, 212–221. doi: 10.1080/03079457.2015.1025696
- Ignjatovic, J. & Sapats, S. (2002). Confirmation of the existence of two distinct genetic groups of infectious bursal disease virus in Australia. Australian Veterinary Journal, 80, 689–694. doi: 10.1111/j.1751-0813.2002.tb11299.x
- Ikuta, N., El-Attrache, J., Villegas, P., García, E.M., Lunge, V.R., Fonseca, A.S., Oliveira, C. & Marques, E.K. (2001). Molecular characterization of Brazilian infectious bursal disease viruses. Avian Diseases, 45, 297–306. doi: 10.2307/1592968
- Ismail, N.M., Saif, Y.M. & Moorhead, P.D. (1988). Lack of pathogenicity of five serotype 2 infectious bursal disease viruses in chickens. Avian Diseases, 32, 757–759. doi: 10.2307/1590995
- Jackwood, D.J., Schat, K.A., Michel, L.O. & de Wit, S. (2018). A proposed nomenclature for infectious bursal disease virus isolates. Avian Pathology, 47, 576–584. doi: 10.1080/03079457.2018.1506092
- Jackwood, D.J. & Sommer-Wagner, S. (2007). Genetic characteristics of infectious bursal disease viruses from four continents. Virology, 365, 369–375. doi: 10.1016/j.virol.2007.03.046
- Jackwood, D.J., Sommer, S.E. & Knoblich, H. V. (2001). Amino acid comparison of infectious bursal disease viruses placed in the same or different molecular groups by RT/PCR-RFLP. Avian Diseases, 45, 330–339. doi: 10.2307/1592972
- Jeon, W.-J., Choi, K.-S., Lee, D.-W., Lee, E.-K., Cha, S.-H., Cho, S.-H., Kwon, J.-H., Yoon, Y., Kim, S.-J., Kim, J.-H. & Kwon, H.-J. (2009). Molecular epizootiology of infectious bursal disease (IBD) in Korea. Virus Genes, 39, 342–351. doi: 10.1007/s11262-009-0394-6
- Kumar, S., Stecher, G. & Tamura, K. (2016). MEGA7: Molecular evolutionary genetics analysis version 7.0 for bigger datasets. Molecular Biology and Evolution, 33, 1870–1874. doi: 10.1093/molbev/msw054
- Kwon, H.M., Kim, D.K., Hahn, T.W., Han, J.H. & Jackwood, D.J. (2000). Sequence of precursor polyprotein gene (segment A) of infectious bursal disease viruses isolated in Korea. Avian Diseases, 44, 691–696. doi: 10.2307/1593113
- Le Nouën, C., Rivallan, G., Toquin, D. & Eterradossi, N. (2005). Significance of the genetic relationships deduced from partial nucleotide sequencing of infectious bursal disease virus genome segments A or B. Archives of Virology, 150, 313–325. doi: 10.1007/s00705-004-0409-5
- Le Nouën, C., Toquin, D., Müller, H., Raue, R., Kean, K.M., Langlois, P., Cherbonnel, M. & Eterradossi, N. (2012). Different domains of the RNA polymerase of infectious bursal disease virus contribute to virulence. PloS one, 7, e28064. doi: 10.1371/journal.pone.0028064
- Lejal, N., Costa, B.D., Huet, J.C., Delmas, B. & Da Costa, B. (2000). Role of Ser-652 and Lys-692 in the protease activity of infectious bursal disease virus VP4 and identification of its substrate cleavage sites. Journal of General Virology, 81, 983–992. doi: 10.1099/0022-1317-81-4-983
- Letunic, I. & Bork, P. (2016). Interactive tree of life (iTOL) v3: an online tool for the display and annotation of phylogenetic and other trees. Nucleic Acids Research, 44, W242–W245. doi: 10.1093/nar/gkw290
- Letzel, T., Coulibaly, F., Rey, F.A., Delmas, B., Jagt, E., van Loon, A.A.M.W. & Mundt, E. (2007). Molecular and structural bases for the antigenicity of VP2 of infectious bursal disease virus. Journal of Virology, 81, 12827–12835. doi: 10.1128/JVI.01501-07
- Lupini, C., Giovanardi, D., Pesente, P., Bonci, M., Felice, V., Rossi, G., Morandini, E., Cecchinato, M. & Catelli, E. (2016). A molecular epidemiology study based on VP2 gene sequences reveals that a new genotype of infectious bursal disease virus is dominantly prevalent in Italy. Avian Pathology, 45, 458–464. doi: 10.1080/03079457.2016.1165792
- Luque, D., Saugar, I., Rejas, M.T., Carrascosa, J.L., Rodríguez, J.F. & Castón, J.R. (2009). Infectious bursal disease virus: ribonucleoprotein complexes of a double-stranded RNA virus. Journal of Molecular Biology, 386, 891–901. doi: 10.1016/j.jmb.2008.11.029
- Martin, A.M., Fallacara, F., Barbieri, I., Tosi, G., Rivallan, G., Eterradossi, N., Ceruti, R. & Cordioli, P. (2007). Genetic and antigenic characterization of infectious bursal disease viruses isolated in Italy during the period 2002-2005. Avian Diseases, 51, 863–872. doi: 10.1637/7904-020107-REGR.1
- Mazariegos, L.A., Lukert, P.D. & Brown, J. (1990). Pathogenicity and immunosuppressive properties of infectious bursal disease “intermediate” strains. Avian Diseases, 34, 203–208. doi: 10.2307/1591353
- McFerran, J.B., McNulty, M.S., McKillop, E.R., Connor, T.J., McCracken, R.M., Collins, D.S. & Allan, G.M. (1980). Isolation and serological studies with infectious bursal disease viruses from fowl, turkeys and ducks: demonstration of a second serotype. Avian Pathology, 9, 395–404. doi: 10.1080/03079458008418423
- Michel, L.O. & Jackwood, D.J. (2017). Classification of infectious bursal disease virus into genogroups. Archives of Virology, 162, 3661–3670. doi: 10.1007/s00705-017-3500-4
- Minta, Z., Roszkowski, J., Karczewski, W. & Kozaczynski, W. (1985a). Properties of indigenous strains of infectious bursal disease virus. II. pathogenicity. Bulletin of the Veterinary Institute in Pulawy, 28–29, 67–73.
- Minta, Z., Roszkowski, J., Karczewski, W. & Kozaczynski, W. (1985b). Properties of indigenous strains of infectious bursal disease virus. III. immunosuppressive effect. Bulletin of the Veterinary Institute in Pulawy, 28–29, 73–76.
- Müller, H., Scholtissek, C. & Becht, H. (1979). The genome of infectious bursal disease virus consists of two segments of double-stranded RNA. Journal of Virology, 31, 584–589.
- Mundt, E., Beyer, J. & Muller, H. (1995). Identification of a novel viral protein in infectious bursal disease virus-infected cells. Journal of General Virology, 76, 437–443. doi: 10.1099/0022-1317-76-2-437
- Ojkic, D., Martin, E., Swinton, J., Binnington, B. & Brash, M. (2007). Genotyping of Canadian field strains of infectious bursal disease virus. Avian Pathology, 36, 427–433. doi: 10.1080/03079450701598408
- R Core Team (2014). R: A language and environment for statistical computing. R Foundation for Statistical Computing: Vienna.
- Reed, L.J. & Muench, H. (1938). A simple method of estimating fifty per cent endpoints. American Journal of Epidemiology, 27, 493–497. doi: 10.1093/oxfordjournals.aje.a118408
- Rosales, A.G., Villegas, P., Lukert, P.D., Fletcher, O.J. & Brown, J. (1989). Immunosuppressive potential and pathogenicity of a recent isolate of infectious bursal disease virus in commercial broiler chickens. Avian Diseases, 33, 724–728. doi: 10.2307/1591151
- Rosenberger, J.K., Cloud S.S., Gelb Jr. J., Odor E., and Dohms J.E. (1985). Sentinel bird survey of Delmarva broiler flocks. In Proceedings of the 20th National Meeting on Poultry Health and Condemnations (pp. 94–101). Ocean City, Oct. 15–16.
- Rosenberger, J.K. & Gelb, J. (1978). Response to several avian respiratory viruses as affected by infectious bursal disease virus. Avian Diseases, 22, 95–105. doi: 10.2307/1589512
- Sánchez, A.B. & Rodriguez, J.F. (1999). Proteolytic processing in infectious bursal disease virus: identification of the polyprotein cleavage sites by site-directed mutagenesis. Virology, 262, 190–199. doi: 10.1006/viro.1999.9910
- Sharma, J.M., Dohms, J.E. & Metz, A.L. (1989). Comparative pathogenesis of serotype 1 and variant serotype 1 isolates of infectious bursal disease virus and their effect on humoral and cellular immune competence of specific-pathogen-free chickens. Avian Diseases, 33, 112–124. doi: 10.2307/1591076
- Shcherbakova, L.O., Lomakin, A.I., Borisov, A. V, Drygin, V. V & Gusev, A.A. (1998). Comparative analysis of the VP2 variable region of the gene from infectious bursal disease virus isolates. Molekuliarnaia Genetika, Mikrobiologiia i Virusologiia, 35–40.
- Snedeker, C., Wills, F.K. & Moulthrop, I.M. (1967). Some studies on the infectious bursal agent. Avian Diseases, 11, 519–528. doi: 10.2307/1588292
- Snyder, D.B. (1990). Changes in the field status of infectious bursal disease virus. Avian Pathology, 19, 419–423. doi: 10.1080/03079459008418695
- Snyder, D.B., Vakharia, V.N. & Savage, P.K. (1992). Naturally occurring-neutralizing monoclonal antibody escape variants define the epidemiology of infectious bursal disease viruses in the United States. Archives of Virology, 127, 89–101. doi: 10.1007/BF01309577
- Soubies, S.M., Courtillon, C., Abed, M., Amelot, M., Keita, A., Broadbent, A., Härtle, S., Kaspers, B. & Eterradossi, N. (2018). Propagation and titration of infectious bursal disease virus, including non-cell-culture-adapted strains, using ex vivo-stimulated chicken bursal cells. Avian Pathology, 47, 179–188. doi: 10.1080/03079457.2017.1393044
- Soubies, S.M., Courtillon, C., Briand, F.-X., Queguiner-Leroux, M., Courtois, D., Amelot, M., Grousson, K., Morillon, P., Herin, J.-B. & Eterradossi, N. (2017). Identification of a European interserotypic reassortant strain of infectious bursal disease virus. Avian Pathology, 46, 19–27. doi: 10.1080/03079457.2016.1200010
- Spies, U., Müller, H. & Becht, H. (1987). Properties of RNA polymerase activity associated with infectious bursal disease virus and characterization of its reaction products. Virus Research, 8, 127–140. doi: 10.1016/0168-1702(87)90024-4
- Stöver, B.C. & Müller, K.F. (2010). Treegraph 2: combining and visualizing evidence from different phylogenetic analyses. BMC Bioinformatics, 11, 7. doi: 10.1186/1471-2105-11-7
- Techera C., Tomás G., Panzera Y., Banda A., Perbolianachis P., Pérez R. & Marandino A. (2019). Development of real-time PCR assays for single and simultaneous detection of infectious bursal disease virus and chicken anemia virus. Molecular and Cellular Probes, 43, 58–60. doi: 10.1016/j.mcp.2018.11.004
- Tomás, G., Hernández, M., Marandino, A., Techera, C., Grecco, S., Hernández, D., Banda, A., Panzera, Y. & Pérez, R. (2017). Development of an RT-qPCR assay for the specific detection of a distinct genetic lineage of the infectious bursal disease virus. Avian Pathology, 46, 150–156. doi: 10.1080/03079457.2016.1228827
- USDA (2018). Livestock and poultry: world markets and trade.
- Vakharia, V.N., He, J., Ahamed, B. & Snyder, D.B. (1994). Molecular basis of antigenic variation in infectious bursal disease virus. Virus Research, 31, 265–273. doi: 10.1016/0168-1702(94)90009-4
- van den Berg, T.P., Gonze, M. & Meulemans, G. (1991). Acute infectious bursal disease in poultry: isolation and characterisation of a highly virulent strain. Avian Pathology, 20, 133–143. doi: 10.1080/03079459108418748
- van den Berg, T.P., Gonze, M., Morales, D. & Meulemans, G. (1996). Acute infectious bursal disease in poultry: Immunological and molecular basis of antigenicity of a highly virulent strain. Avian Pathology, 25, 751–768. doi: 10.1080/03079459608419179
- Vera, F., Craig, M.I., Olivera, V., Rojas, F., König, G., Pereda, A. & Vagnozzi, A. (2015). Molecular characterization of infectious bursal disease virus (IBDV) isolated in Argentina indicates a regional lineage. Archives of Virology, 160, 1909–1921. doi: 10.1007/s00705-015-2449-4
- Xie Z., Fadl A.A., Girshick T. & Khan M.I. (1997). Amplification of avian reovirus RNA using the reverse transcriptase-polymerase chain reaction. Avian Diseases, 41, 654–660. doi: 10.2307/1592157
- Yamazaki, K., Ohta, H., Kawai, T., Yamaguchi, T., Obi, T. & Takase, K. (2017). Characterization of variant infectious bursal disease virus from a broiler farm in Japan using immunized sentinel chickens. Journal of Veterinary Medical Science, 79, 175–183. doi: 10.1292/jvms.16-0301
- Zachar, T., Popowich, S., Goodhope, B., Knezacek, T., Ojkic, D., Willson, P., Ahmed, K.A. & Gomis, S. (2016). A 5-year study of the incidence and economic impact of variant infectious bursal disease viruses on broiler production in Saskatchewan, Canada. Canadian Journal of Veterinary Research, 80, 255–261.