ABSTRACT
To date, four subgroups of avian metapneumoviruses have been defined (AMPV-A, B, C and D) based on genetic and antigenic differences. The extent of infection in the three principal species (turkeys, chickens and ducks) by these subgroups is, however, not well defined. Here, a series of controlled and ethically approved experimental infections were performed in specific pathogen-free turkeys, chickens and ducks with each of the four AMPV subgroups. For subgroup C, one strain isolated from turkeys in the USA (turkey AMPV-C) and one isolated from ducks in France (duck AMPV-C) were compared. Globally, these extensive experimental trials demonstrated that AMPV-A, B, turkey C and D were well adapted to Galliformes, especially turkeys; however, chickens showed limited clinical signs and differences in seroconversion and transmission. Notably, chickens did not transmit AMPV-A to contacts and were shown for the first time to be susceptible to AMPV-D. The duck AMPV-C was well adapted to ducks; however, chickens and turkeys seroconverted and were positive by virus isolation. In addition, seroconversion of contact turkeys to duck AMPV-C demonstrated horizontal transmission of this virus in a non-palmiped species under our experimental conditions. Interestingly, in chickens and turkeys, duck AMPV-C isolation was possible despite a lack of detection of viral RNA. Likewise, the turkey AMPV-C virus was well adapted to turkeys yet was also isolated from chickens despite a lack of detection of viral RNA. These results would suggest a selection for viral genetic sequences that differ from the original strain upon adaptation to a ‘non-conventional host’.
Introduction
Avian metapneumoviruses (AMPVs) cause respiratory and genital disorders in poultry, most notably, turkeys, chickens and ducks (Jones & Rautenschlein, Citation2013; Eterradossi et al., Citation2015) and are a major economic concern for the industry. Initially detected in turkey flocks in South Africa in the late 1970s (Buys et al., Citation1989), the virus was known as turkey rhinotracheitis virus (TRT). Since then, many studies (Giraud et al., Citation1986; McDougall & Cook, Citation1986; Wilding et al., Citation1986; Cavanagh & Barrett, Citation1988; Collins & Gough, Citation1988; Brown et al., Citation2014) have led to the current classification: order Mononegavirales, family Pneumoviridae, genus Metapneumovirus (Afonso et al., Citation2016). To date, four subgroups of AMPV (A, B, C and D) and two lineages of subgroup C have been identified based on antigenic and genetic differences (Juhasz & Easton, Citation1994; Eterradossi et al., Citation1995; Seal, Citation1998; Bayon-Auboyer et al., Citation1999; Toquin, Guionie, Jestin et al., Citation2006; Brown et al., Citation2014), and literature indicates a difference in host range for these. Concerning the principal species, all subgroups have been detected in turkeys (Alexander et al., Citation1986; Giraud et al., Citation1986; Naylor et al., Citation1997; Senne et al., Citation1997; Bayon-Auboyer et al., Citation2000); however, it is important to note that the subgroup C viruses detected in this species are of a specific North American lineage (turkey AMPV-C). To date, no subgroup C virus of Eurasian lineage (duck AMPV-C), detected in ducks, has been reported in turkeys. AMPV-A, B, and C have been detected in chickens (Picault et al., Citation1987; Jones et al., Citation1991; Mase et al., Citation2003; Wei et al., Citation2013). A and B have been well documented in this species; however, the C virus has only been reported in one case in China (Wei et al., Citation2013). Only sequence of the conserved M gene is available for this virus and thus it is not known if its full-length genome sequence clusters with the turkey or duck AMPV-C lineage or if indeed it forms the third lineage. Concerning ducks, only subgroup C viruses of duck lineage have been detected (Toquin et al., Citation1999; Sun et al., Citation2014).
Although these data give a good broad picture of the host range of the different subgroups, it is not yet clear to what extent each subgroup infects a particular host. Indeed, three experimental studies have indicated that the AMPV host range picture could be more complicated (Cook et al., Citation1999; Shin et al., Citation2001; Toquin, Guionie, Allee et al., Citation2006). Interestingly, in those studies, seroconversion was observed in the absence of clinical signs in chickens, Peking ducks and Muscovy ducks inoculated with turkey AMPV-C (Cook et al., Citation1999; Shin et al., Citation2001; Toquin, Guionie, Allee, et al., Citation2006), and in turkeys inoculated with Muscovy duck AMPV-C (Toquin, Guionie, Allee, et al., Citation2006). Furthermore, turkey AMPV-C was successfully isolated from the nasal turbinate of Peking ducks in the study of Shin et al. (Citation2001) and from the tracheas of Muscovy ducks in the study of Toquin, Guionie, Allee, et al. (Citation2006). Such data demonstrating the potential for the different AMPV C lineages to infect “non-conventional” hosts, and in the absence of clinical signs, could imply that the other subgroups have the same potential, although there is no report to date of natural infection of ducks by AMPV-A, B or D.
Thus, for the first time and in an attempt to define more precisely the host range of the different AMPV subgroups, this study reports the findings of a harmonized series of controlled and ethically approved experimental infections performed between 2014 and 2016 in specific pathogen-free (SPF) turkeys, chickens and ducks with each of the four AMPV subgroups. Birds were monitored for four weeks following experimental inoculation.
Materials and methods
Viruses
All viruses had been previously isolated in Vero cells according to the method of Giraud et al. (Citation1987). All viruses had been collected from France unless otherwise stated. AMPV-A 85051 was a 3rd passage of a 1985 turkey isolate (Toquin et al., Citation2000), AMPV-B 86004 a 5th passage of a 1986 turkey isolate (Bayon-Auboyer et al., Citation1999), AMPV-C 193adv 9802 a 3rd passage of a 1997 turkey isolate from the United States, collected after 12 passages in chick embryo fibroblasts (kind gift of Dr D. Senne, APHIS), AMPV-C 99178 a 1st passage of a 1999 Muscovy duck isolate (Toquin et al., Citation1999) and AMPV-D 85035 a 3rd passage of a 1985 turkey isolate (Bayon-Auboyer et al., Citation2000). All virus stocks were titrated according to Reed and Muench (Citation1938) in Vero cells maintained in 96-well plates, using 10-fold dilutions of the virus stocks and eight repeats per dilution.
Birds
Twenty-eight-day-old SPF turkeys (Meleagris gallopavo) and chickens (Gallus gallus domesticus), and 18-day-old SPF Muscovy ducks (Cairina moschata) were used at day 0 of the bird experiments. All SPF birds were from Anses Ploufragan-Plouzané-Niort laboratory, France. Ages used in the experiments were chosen as they represent the age when the birds reach a higher susceptibility to respiratory infections.
Bird experiments
Five bird trials were performed under identical conditions. In the first trial, SPF turkeys, chickens and ducks were inoculated with AMPV-A 85051, in the second with AMPV-B 86044, in the third with AMPV-D 85035, in the fourth with AMPV-C 193adv 9802, and in the fifth with AMPV-C 99178.
In each trial, three A2 containment cells (Cc1, 2 and 3) were allocated to infection studies and a fourth (Cc4) for mock-inoculation. Turkeys were housed in one cage in Cc1, chickens in one cage in Cc2 and ducks in one cage in Cc3. In Cc4, turkeys, chickens and ducks were housed in separate cages ((a)). In Cc1, 2 and 3, 13 birds were inoculated via the intranasal route with 100 µl of virus at 104.5 TCID50/ml in MEM supplemented with HEPES (MEMH), penicillin and streptomycin at 20 mM, 200 u/ml, 0.2 mg/ml final concentration respectively. In Cc4, eight birds of each species were mock-inoculated with 100 µl of the supplemented MEM medium described above. Three days post inoculation (dpi), five turkeys, five chickens and five ducks that had not been mock-inoculated, but were housed in Cc4, were introduced as contact subjects to Cc1, 2, and 3, respectively. Details of the days when sampling was performed in each Cc and the type of sample taken with its downstream analysis are shown in (a, b), respectively. A total of 26 turkeys, 26 chickens and 26 ducks were used per experiment.
Figure 1. (a) Table summarizing how each trial was organized and detailing the specific days when birds were sampled (grey shaded boxes). Two different series (S1 and S2) within the virus-inoculated groups Cc1, 2 and 3 were constructed so that the same birds were not subjected to unpleasant swabbing of the trachea everyday. (b) Flow diagram of the experimental procedure from the day of inoculation (0) to the end of the trial (28 dpi).
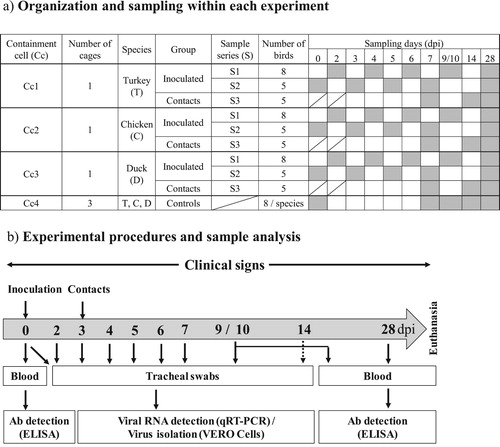
For sampling ethics, virus inoculated birds in Cc1, 2 and 3 were separated into two series that were sampled alternatively every second day, so that the same birds were not subjected to swabbing of the trachea every day.
Clinical signs
Clinical signs were observed by trained examiners, using previously reported criteria (Toquin, Guionie, Allee et al., Citation2006). Briefly, birds were observed for nasal discharge, nasal crust on one or two nostrils and mild or serious cough or rale.
Detecting viral RNA loads by qRT-PCR
RNA was extracted from tracheal swabs that had been re-suspended in 1.2 ml of MEMH supplemented with penicillin and streptomycin at 200 u/ml and 0.2 mg/ml final concentrations, respectively. Extractions were performed using MagAttract Virus Mini M48 Kit (Qiagen) according to the manufacturers’ instructions. The levels of AMPV-specific RNA in these extracts were then assessed using subtype-specific qRT-PCRs as previously described (Guionie et al., Citation2007). Results are given as mean viral RNA copy (cp), expressed in log10/reaction (5 µl).
Detecting excretion of infectious virus
Tracheal swabs that had been re-suspended as described earlier were inoculated directly onto Vero cells (250 µl/well of a 6 well plate) for virus isolation as previously described (Giraud et al., Citation1987; Toquin, Guionie, Allee, et al., Citation2006). Inoculated cell layers were observed daily under light microscopy and checked for AMPV typical cytopathogenic effect (CPE) (both focal and syncytial). Samples were considered negative after three serial passages in Vero cells.
Detecting anti-AMPV-A, B, C or D antibody
Serological testing was performed with an in-house ELISA using subgroup specific antigens as previously described (Toquin et al., Citation2000).
Results
Clinical signs
Turkeys inoculated with either AMPV-B, D or the turkey AMPV-C isolate had observable clinical signs from 3 to 11, 3 to 7, and 3 to 6 dpi, respectively. These encompassed watery eyes with a clear, then turbid nasal exudate, occasionally accompanied by a dry tracheal cough. Turkeys inoculated with AMPV-A demonstrated the same clinical signs around the eyes and beak from 3 to 10 dpi, although these were much less pronounced. No coughing was observed. In all cases turkeys had recovered by 10 or 11 dpi and from this point on remained free from clinical signs. Contact turkeys developed the same clinical signs as their respective inoculated co-inhabitants within 2 or 3 days after introduction. No clinical signs were observed in turkeys inoculated with the duck AMPV-C isolate.
Ducks inoculated with the duck AMPV-C isolate had observable clinical signs from 5 to 10 dpi with heavy congestion in the trachea (rales audible at 2 or 3 metres) and occasional sneezing with nasal discharge. Ducks had recovered by 12 dpi and, from this point, remained without any clinical signs until the end of the experiments. Contact ducks developed the same clinical signs as their respective, inoculated co-inhabitants within 4 or 5 days after introduction. No clinical signs were observed in ducks following inoculation with AMPV-A, B, D or the turkey AMPV-C isolate.
Clinical signs were very limited or absent in chickens in all trials.
Detection of viral RNA, anti-AMPV antibodies and isolation of viruses
The following data summarizes , which should be used alongside this text.
Figure 2. Results of the five bird trials undertaken in this study. Each row of three graphics shows how turkeys, chickens and ducks responded to a particular subgroup of AMPV, from top to bottom: AMPV-A, B, turkey C, duck C and D. The X-axis in each histogram shows the days post inoculation. The Y-axis to the left of each histogram should be used to read the cumulative number of positive and negative subjects by real-time qRT-PCR in the bars above the X-axis, and by ELISA in the bars below the X-axis. The Y-axis to the right of each histogram should be used to read the detail of RNA detection (dots above the X-axis), expressed as mean RNA copies/reaction, and of antibody detection (dots below the X-axis) expressed as mean ELISA optical density (OD). + Symbols identify positive virus isolation.
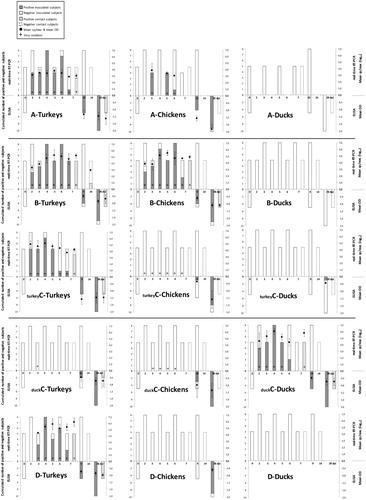
In inoculated and contact turkeys
Turkeys demonstrated similar responses to infection with either AMPV-A, B, D or the turkey AMPV-C isolate in terms of the onset and duration of detectable viral RNA in the trachea, excretion of infectious virus, and the production of anti-AMPV antibody. In each case viral RNA was detected from 2 or 3 dpi to 7 dpi in the tracheas of inoculated turkeys and in the tracheas of contact turkeys 5 days post contact (7 dpi, ). Viral RNA loads were highest in turkeys infected with AMPV-D with a maximum of 106.21 cp/5 µl. This figure was one log10 higher than those observed in turkeys infected with either AMPV-B or the turkey AMPV-C isolate (maximums, 105.27 and 105.28 cp/5 µl, respectively). Maximum viral RNA loads in the tracheas of turkeys infected with AMPV-A were notably lower at 103.78 cp/5 µl. Virus was isolated in Vero cells from tracheal swabs of inoculated and contact turkeys in these four trials from 2 or 3 dpi to 7 dpi and 5 days post contact (7 dpi, ), respectively. Infected Vero cells demonstrated typical focal (APMV-A, B, D and turkey AMPV-C) and syncytial (AMPV-A and turkey AMPV-C) type CPE. Inoculated and contact turkeys of each trial seroconverted, with the exception of one turkey in the AMPV-B trial.
Concerning turkeys infected with the duck AMPV-C isolate, no viral RNA was detected in the tracheal swabs throughout the trial. However, virus was isolated in Vero cells from tracheal swabs of inoculated birds at 3 dpi. Infected Vero cells demonstrated syncytial type CPE akin to duck AMPV-C.
At 28 dpi, all inoculated birds and two out of five contact birds were AMPV-C seropositive.
In inoculated and contact chickens
Chickens had very similar responses to AMPV-B infection as turkeys, with viral RNA being detected from 2 or 3 dpi to 7 dpi in the trachea of inoculated chickens and at 5 days post contact in the tracheas of contact chickens. Maximum viral RNA loads were of a similar magnitude as those observed in turkeys at 105.51 cp/5 µl. Virus was also isolated in Vero cells from tracheal swabs of inoculated and contact chickens from 2 or 3 dpi to 7 dpi and 5 days post contact (7 dpi, ), respectively. Infected Vero cells demonstrated focal type CPE akin to AMPV-B. Seroconversion was observed in all but one inoculated chicken and in all contact birds by 28 dpi. A limited response was observed in chickens infected with AMPV-A in terms of numbers of birds positive for viral RNA in the trachea (five out of 13 chickens), although viral RNA loads in these birds were of similar magnitude as those observed in turkeys (maximum 103.89 cp/5 µl) and virus was isolated. All but one inoculated chicken was AMPV-A seropositive at 28 dpi. No viral RNA, infectious virus or anti-AMPV-A antibodies were detected in contact chickens. Inoculated and contact chickens of the AMPV-D, turkey AMPV-C and duck AMPV-C trials, were negative for viral RNA throughout and only inoculated chickens were seropositive to these viruses at 28 dpi. However, virus was isolated in Vero cells from the tracheas of inoculated chickens from 2 to 7 dpi and from 3 to 6 dpi following infection with turkey AMPV-C and duck AMPV-C, respectively. Infected Vero cells demonstrated focal + syncytial and syncytial-type CPE akin to turkey and duck AMPV-C, respectively.
In inoculated and contact ducks
Ducks had very similar responses after infection with duck AMPV-C to those observed in turkeys after infection with AMPV-B, D and turkey AMPV-C. Viral RNA was detected from 2 to 6 dpi with maximum viral RNA loads of 106.07 cp/5 µl. Virus was isolated in Vero cells from the tracheal swabs of inoculated and contact ducks from 2 to 5 dpi and 5 days post contact (7 dpi, ), respectively. Infected Vero cells demonstrated syncytial-type CPE akin to duck AMPV-C. Seroconversion was observed in all inoculated and contact ducks at 28 dpi. No viral RNA, infectious virus or anti-AMPV antibodies were detected in ducks inoculated with AMPV-A, B, D or the turkey AMPV-C virus with the exception of one duck that seroconverted to the turkey AMPV-C virus.
In control birds
Control turkeys, chickens and ducks of each of the five clinical trials were negative throughout the experiments (data not shown).
Discussion
Current literature indicates that AMPV-A and B are mainly viruses of turkeys and chickens, that the North American and Eurasian AMPV-C viruses are mainly viruses of turkeys or ducks, respectively, and that the AMPV-D is a virus of turkeys. However, it also shows that, under experimental conditions, ducks and chickens can become seropositive to the turkey C lineage and that turkeys can become seropositive to the duck C lineage of AMPV-C (Cook et al., Citation1999; Shin et al., Citation2001; Toquin, Guionie, Allee et al., Citation2006). Until now, however, no single series of harmonized experimental trials have been performed that provide one set of reference data concerning the extent of infection of AMPV-A, B, both C lineages and D in the three principal species (turkeys, chickens, and ducks) in terms of clinical signs induced, serological responses, onset and duration of detectable viral RNA in the trachea, excretion of infectious virus and horizontal transmission to naïve contacts.
From the current study, it is clear that both turkeys and chickens are susceptible to AMPV-A and B infection; however, subtle differences were observed. Firstly, in terms of clinical signs, at the peak of infection (around 3–6 dpi) chickens presented very little, as has been previously reported (Jones et al., Citation1987; Majo et al. Citation1995; Cook, Citation2000; Jones & Rautenschlein, Citation2013), whereas turkeys had clear visible cold-like signs with dry tracheal coughs and mucus secretions from the beak. Secondly, chickens did not transmit AMPV-A horizontally to naïve contacts which was a surprising result, as infectious virus was isolated from the trachea as late as 6 dpi. Perhaps this means that infectious virus in the trachea is not easily secreted into the environment in the absence of coughing and that virus replication higher up in the respiratory pathway, such as in the nasal turbinates where virus can be more easily secreted in mucus from the beak for example, is required for efficient transmission. In support of this idea is the fact that, in all cases where horizontal transmission was observed in galliforms, nasal exudates were present.
The transposition of experimental findings to the field in which infections can be, and often are, complicated by opportune secondary pathogens, is always to be treated with caution. However, this incapacity of chickens to transmit AMPV-A horizontally may be one explanation for the global predominance of subgroup B over A in galliforms.
The AMPV-D virus is clearly well adapted to turkeys as this species was the only species in which inoculated and contact birds were positive in all laboratory tests. That said, seroconversion was observed in inoculated chickens, showing for the first time that this species is susceptible to AMPV-D infection. The lack of viral RNA detection and isolation of virus from the trachea though, may suggest that the replication level of AMPV-D in chickens, under experimental conditions, is limited. It is also possible that AMPV-D has a different target organ for replication in the chicken. This merits further investigation. Looking at these results, it is surprising that AMPV-D viruses have only been detected in turkeys in one case in France in the 1980s (Bayon-Auboyer et al., Citation2000); however, this may reflect the fact that most molecular diagnostic tools applied in cases of AMPV suspicion for galliforms are AMPV-A, B or C specific. Thus, field studies using either broad range or subgroup-specific diagnostic assays merit implementation, to either assess the presence of any AMPV subgroup including potentially un-discovered or future emerging AMPVs, or achieve a more precise distribution of the four known subgroups, respectively. Muscovy ducks were not at all susceptible to AMPV-A, B and D infection, as previously reported (Toquin, Guionie, Allee et al., Citation2006).
Results of experimental infections with the duck and turkey lineages of AMPV-C showed that, in addition to a standard infection profile in their respective original host species, the duck virus can infect turkeys and both the duck and turkey virus can infect chickens. Both inoculated and contact turkeys also seroconverted to the duck AMPV-C virus demonstrating, for the first time, horizontal transmission events of duck AMPV-C in a non-palmiped under controlled experimental conditions. Interestingly, in both cases where the different lineages of AMPV-C infected a “non-conventional host” virus was isolated from tracheas despite a lack of viral RNA detection in the same tracheal samples by a well-characterized AMPV-C specific qRT-PCR (Guionie et al., Citation2007) targeting the small hydrophobic gene. As molecular diagnostics are considered more sensitive than virus isolation, it is expected that the viral genomes of the duck and turkey AMPV-Cs underwent some level of modification within the chicken as did the duck AMPV-C in the turkey. As the small hydrophobic gene is the second most variable gene of the AMPV genome, and reverse genetics studies have shown that deleting this protein results in phenotype changes in vitro, and attenuation in vivo, then it may not be surprising to find genetic modifications in or around this area upon adaptation to a “non-conventional host”. If modifications to the AMPV-C viral sequences have occurred, however, in the current study these would appear not to have altered small hydrophobic functionality in vitro, as no change in CPE was observed for these viruses in Vero cells.
Future studies will concern deep sequencing of these isolated viruses and their parental inoculum. This will give in-depth information on sequence changes that occurred within the virus population, possibly demonstrating evolution and selection of virus sequences that were more fitting to the “non-conventional” host. Also, in light of the current findings, it is envisaged to undertake similar studies to look at the susceptibility of minor poultry species, such as Guinea fowl, quail and pheasant, in which AMPVs have also been documented.
Ethical statement
All bird experiments were performed in agreement with the national regulations on animal welfare from the French Ministry for higher education and research, and after approval from the French Agency for Food, Environmental and Occupational Health & Safety’s (ANSES) ethical committee (N°16 document 14-024).
Disclosure statement
No potential conflict of interest was reported by the authors.
Additional information
Funding
References
- Afonso, C.L., Amarasinghe, G.K., Banyai, K., Bao, Y., Basler, C.F., Bavari, S., Bejerman, N., Blasdell, K.R., Briand, F.X., Briese, T., Bukreyev, A., Calisher, C.H., Chandran, K., Cheng, J., Clawson, A.N., Collins, P.L., Dietzgen, R.G., Dolnik, O., Domier, L.L., Durrwald, R., Dye, J.M., Easton, A.J., Ebihara, H., Farkas, S.L., Freitas-Astua, J., Formenty, P., Fouchier, R.A., Fu, Y., Ghedin, E., Goodin, M.M., Hewson, R., Horie, M., Hyndman, T.H., Jiang, D., Kitajima, E.W., Kobinger, G.P., Kondo, H., Kurath, G., Lamb, R.A., Lenardon, S., Leroy, E.M., Li, C.X., Lin, X.D., Liu, L., Longdon, B., Marton, S., Maisner, A., Muhlberger, E., Netesov, S.V., Nowotny, N., Patterson, J.L., Payne, S.L., Paweska, J.T., Randall, R.E., Rima, B.K., Rota, P., Rubbenstroth, D., Schwemmle, M., Shi, M., Smither, S.J., Stenglein, M.D., Stone, D.M., Takada, A., Terregino, C., Tesh, R.B., Tian, J.H., Tomonaga, K., Tordo, N., Towner, J.S., Vasilakis, N., Verbeek, M., Volchkov, V.E., Wahl-Jensen, V., Walsh, J.A., Walker, P.J., Wang, D., Wang, L.F., Wetzel, T., Whitfield, A.E., Xie, J.T., Yuen, K.Y., Zhang, Y.Z. & Kuhn, J.H. (2016). Taxonomy of the order mononegavirales: update 2016. Archives of Virology, 161, 2351–2360. doi: 10.1007/s00705-016-2880-1
- Alexander, D.J., Gough, R.E., Wyeth, P.J., Lister, S.A. & Chettle, N.J. (1986). Viruses associated with turkey rhinotracheitis in Great Britain. The Veterinary Record, 118, 217–218. doi: 10.1136/vr.118.8.217
- Bayon-Auboyer, M.H., Arnauld, C., Toquin, D. & Eterradossi, N. (2000). Nucleotide sequences of the F, L and G protein genes of two non-A/non-B avian pneumoviruses (APV) reveal a novel APV subgroup. Journal of General Virology, 81, 2723–2733. doi: 10.1099/0022-1317-81-11-2723
- Bayon-Auboyer, M.H., Jestin, V., Toquin, D., Cherbonnel, M. & Eterradossi, N. (1999). Comparison of F-, G- and N-based RT-PCR protocols with conventional virological procedures for the detection and typing of turkey rhinotracheitis virus. Archives of Virology, 144, 1091–1109. doi: 10.1007/s007050050572
- Brown, P.A., Lemaitre, E., Briand, F.X., Courtillon, C., Guionie, O., Allee, C., Toquin, D., Bayon-Auboyer, M.H., Jestin, V. & Eterradossi, N. (2014). Molecular comparisons of full length metapneumovirus (MPV) genomes, including newly determined French AMPV-C and -D isolates, further supports possible subclassification within the MPV genus. PLoS One, 9, e102740. doi: 10.1371/journal.pone.0102740
- Buys, S.B., du Preez, J.H. & Els, H.J. (1989). The isolation and attenuation of a virus causing rhinotracheitis in turkeys in South Africa. Onderstepoort Journal of Veterinary Research, 56, 87–98.
- Cavanagh, D. & Barrett, T. (1988). Pneumovirus-like characteristics of the mRNA and proteins of turkey rhinotracheitis virus. Virus Research, 11, 241–256. doi: 10.1016/0168-1702(88)90086-X
- Collins, M.S. & Gough, R.E. (1988). Characterization of a virus associated with turkey rhinotracheitis. Journal of General Virology, 69, 909–916. doi: 10.1099/0022-1317-69-4-909
- Cook, J.K. (2000). Avian rhinotracheitis. Revue Scientifique et Technique, 19, 602–613. doi: 10.20506/rst.19.2.1233
- Cook, J.K., Huggins, M.B., Orbell, S.J. & Senne, D.A. (1999). Preliminary antigenic characterization of an avian pneumovirus isolated from commercial turkeys in Colorado, USA. Avian Pathology, 28, 607–617. doi: 10.1080/03079459994407
- Eterradossi, N., Toquin, D., Guittet, M. & Bennejean, G. (1995). Evaluation of different turkey rhinotracheitis viruses used as antigens for serological testing following live vaccination and challenge. Veterinary Medicine, 42, 175–186. doi: 10.1111/j.1439-0450.1995.tb00698.x
- Eterradossi, N., Toquin, D., Picault, J.P. & Jestin, V. (2015). Métapneumoviroses Aviaires. In J. Brugère-Picoux (Ed.), Manuel de Pathologie Aviaire (pp. 156–163). Paris: AFAS.
- Giraud, P., Bennejean, G., Guittet, M. & Toquin, D. (1986). Turkey rhinotracheitis in France: preliminary investigations on a ciliostatic virus. The Veterinary Record, 119, 606–607.
- Giraud, P., Le Gros, F.X., Bouquet, J.F., Toquin, D. & Bennejean, G. (1987). Turkey rhinotracheitis: isolation of a viral agent and first trials with experimental inactivated or attenuated vaccines. In 36th Western Poultry Disease Conference (pp. 94–95), Davis, CA.
- Guionie, O., Toquin, D., Sellal, E., Bouley, S., Zwingelstein, F., Allee, C., Bougeard, S., Lemiere, S. & Eterradossi, N. (2007). Laboratory evaluation of a quantitative real-time reverse transcription PCR assay for the detection and identification of the four subgroups of avian metapneumovirus. Journal of Virological Methods, 139, 150–158. doi: 10.1016/j.jviromet.2006.09.022
- Jones, R.C., Baxter-Jones, C., Savage, C.E., Kelly, D.F. & Wilding, G.P. (1987). Experimental infection of chickens with a ciliostatic agent isolated from turkeys with rhinotracheitis. The Veterinary Record, 120, 301–302. doi: 10.1136/vr.120.13.301
- Jones, R.C., Naylor, C.J., Bradbury, J.M., Savage, C.E., Worthington, K. & Williams, R.A. (1991). Isolation of a turkey rhinotracheitis-like virus from broiler breeder chickens in England. The Veterinary Record, 129, 509–510. doi: 10.1136/vr.129.21.476
- Jones, R.C. & Rautenschlein, S. (2013). Avian metapneumovirus. In D.E. Swayne (Ed.), Diseases of Poultry (pp. 112–138). Ames, IA: Wiley-Blackwell.
- Juhasz, K. & Easton, A.J. (1994). Extensive sequence variation in the attachment (G) protein gene of avian pneumovirus: evidence for two distinct subgroups. Journal of General Virology, 75, 2873–2880. doi: 10.1099/0022-1317-75-11-2873
- Majo, N., Allan, G.M., O’Loan, C.J., Pages, A. & Ramis, A.J. (1995). A sequential histopathologic and immunocytochemical study of chickens, turkey poults, and broiler breeders experimentally infected with turkey rhinotracheitis virus. Avian Diseases, 39, 887–896. doi: 10.2307/1592428
- Mase, M., Yamaguchi, S., Tsukamoto, K., Imada, T., Imai, K. & Nakamura, K. (2003). Presence of avian pneumovirus subtypes A and B in Japan. Avian Diseases, 47, 481–484. doi: 10.1637/0005-2086(2003)047[0481:POAPSA]2.0.CO;2
- McDougall, J.S. & Cook, J.K. (1986). Turkey rhinotracheitis: preliminary investigations. The Veterinary Record, 118, 206–207. doi: 10.1136/vr.118.8.206
- Naylor, C., Shaw, K., Britton, P. & Cavanagh, D. (1997). Appearance of type B avian pneumovirus in Great Britain. Avian Pathology, 26, 327–338. doi: 10.1080/03079459708419215
- Picault, J.P., Giraud, P., Drouin, P., Guittet, M., Bennejean, G., Lamande, J., Toquin, D. & Gueguen, C. (1987). Isolation of a TRTV-like virus from chickens with swollen-head syndrome. The Veterinary Record, 121, 135. doi: 10.1136/vr.121.6.135-a
- Reed, L.J. & Muench, H. (1938). A simple method of estimating fifty percent end points. American Journal of Hygiene, 27, 493–497.
- Seal, B.S. (1998). Matrix protein gene nucleotide and predicted amino acid sequence demonstrate that the first U. S. avian pneumovirus isolate is distinct from European strains. Virus Research, 58, 45–52. doi: 10.1016/S0168-1702(98)00098-7
- Senne, D.A., Edson, R.K., Pederson, J.C. & Panigrahy, B. (1997). Avian Pneumovirus Update. Schaumburg, IL: American Veterinary Medical Association.
- Shin, H.J., Njenga, M.K., Halvorson, D.A., Shaw, D.P. & Nagaraja, K.V. (2001). Susceptibility of ducks to avian pneumovirus of turkey origin. American Journal of Veterinary Research, 62, 991–994. doi: 10.2460/ajvr.2001.62.991
- Sun, S., Chen, F., Cao, S., Liu, J., Lei, W., Li, G., Song, Y., Lu, J., Liu, C., Qin, J. & Li, H. (2014). Isolation and characterization of a subtype C avian metapneumovirus circulating in Muscovy ducks in China. Veterinary Research, 45, 74. doi: 10.1186/s13567-014-0074-y
- Toquin, D., Bayon-Auboyer, M.H., Eterradossi, N. & Jestin, V. (1999). Isolation of a pneumovirus from a Muscovy duck. The Veterinary Record, 23, 680.
- Toquin, D., Bayon-Auboyer, M.H., Senne, D.A. & Eterradossi, N. (2000). Lack of antigenic relationship between French and recent North American non-A/non-B turkey rhinotracheitis viruses. Avian Diseases, 44, 977–982. doi: 10.2307/1593075
- Toquin, D., Guionie, O., Allee, C., Morin, Y., Le CoQ, L., Zwingelstein, F., Jestin, V. & Eterradossi, N. (2006). Compared susceptibility of SPF ducklings and SPF turkeys to the infection by avian metapneumoviruses belonging to the four subgroups. In U. Heffels-Redman & E.F. Kaleta (Eds.), V. International Symposium on Avian Corona and Pneumoviruses and Complicating Pathogens (pp. 70–76). University of Giessen Rauischholzhausen, Germany: VVB Laufersweiler Verlag.
- Toquin, D., Guionie, O., Jestin, V., Zwingelstein, F., Allee, C. & Eterradossi, N. (2006). European and American subgroup C isolates of avian metapneumovirus belong to different genetic lineages. Virus Genes, 32, 97–103. doi: 10.1007/s11262-005-5850-3
- Wei, L., Zhu, S., Yan, X., Wang, J., Zhang, C., Liu, S., She, R., Hu, F., Quan, R. & Liu, J. (2013). Avian metapneumovirus subgroup C infection in chickens, China. Emerging Infectious Diseases, 19, 1092–1094. doi: 10.3201/eid1907.121126
- Wilding, G.P., Baxter-Jones, C. & Grant, M. (1986). Ciliostatic agent found in rhinotracheitis. The Veterinary Record, 118, 735. doi: 10.1136/vr.118.26.735-b