ABSTRACT
Scanning electron microscopy observations of the distal leg region of the poultry red mite Dermanyssus gallinae (De Geer 1778) identified the presence of a compound ambulacrum, the part of the leg that contacts the substratum when the mite walks. The ambulacrum is comprised of a praetarsus (the ambulacrum stalk), a pulvillus and two claws. The pulvillus is a weakly sclerotized structure that can be partly expanded or retracted in the praetarsus. When expanded, the pulvillus shows a cushion-like shape which can, as a result of its soft surface, function as a sucker, thus allowing D. gallinae to adhere to a smooth surface. When traversing an irregular surface, or clinging to a soft surface, the mite retracts the pulvillus and uses only its strongly sclerotized movable claws. These observed morphological adaptations explain the ability of D. gallinae to climb upwards on a slippery surface, resist an air flux, walk on smooth and rigid feathers of its avian hosts, and cling to the bird’s or human's soft skin. This knowledge is important to better understand the attachment mechanism of this species to its host and to the substratum on which it moves, and also to provide insight into the circumstances under which it is able to move.
RESEARCH HIGHLIGHTS
The ambulacrum is the distal part of the leg touching the substratum
D. gallinae’s ambulacrum consists of a praetarsus, a pulvillus and two claws
The weakly sclerotized pulvillus can be part expanded/retracted in the praetarsus
The expanded pulvillus functions as a sucker to adhere to smooth surfaces
The claws are used to walk on an irregular surface or cling to a soft surface
Introduction
Dermanyssus gallinae (De Geer 1778), commonly known as the poultry red mite (PRM), is a haematophagous ectoparasitic mite of wild, domestic and synanthropic birds. Worldwide, D. gallinae is the single most significant pest in egg-laying hen facilities, with infestations causing reduced animal welfare via disturbance to the birds. Depending upon infestation levels, D. gallinae can cause flocks to experience irritation, anaemia, transfer of diseases, diminished disease resistance, reduced weight gain, and, in severe cases, even death (Axtel & Arends, Citation1990; Chauve, Citation1998; Chirico et al., Citation2003; Moro et al., Citation2007; Kilpinen et al., Citation2005; Van Emous et al., Citation2005; Fossum et al., Citation2009; Mul et al., Citation2009; Sparagano & Giangaspero, Citation2011). From a production perspective, D. gallinae is responsible for reduced egg-quality, quantity and grading, resulting in high economic losses. Infestations can quickly develop to high levels as a result of the D. gallinae life cycle being as short as 7 days under optimal conditions (25–35°C and 65–90% RH and presence of the natural host) (Maurer & Baumgärtner, Citation1992, Citation1994; Chauve, Citation1998; Tucci & Guimareas, Citation1998; Nordenfors et al., Citation1999). Control and production losses combined have been estimated at €130 million per annum for egg-producing poultry farms in Europe (Mul et al., Citation2010). Furthermore, D. gallinae can act as a potential vector and reservoir for several infectious disease agents (including eastern and western equine encephalitis, Saint Louis encephalitis, Newcastle disease, tick-borne encephalitis and West Nile viruses, Coxiella burnetii, Salmonella spp. and avian influenza A virus) thus representing a potential zoonotic agent whose significance may extend beyond poultry (Valiente-Moro et al., Citation2005, Citation2007; George et al., Citation2015; Sommer et al., Citation2016).
Dermanyssus gallinae has four developmental instars (larva, protonymph, deutonymph and adult) (Wood, Citation1917), with larvae being the only stage that do not feed on the host (Axtell & Arends, Citation1990). The mites usually visit host birds to feed only during hours of darkness; during daylight hours they prefer to hide in cracks and crevices in the vicinity of the hens' nightly resting places (Maurer & Baumgärtner, Citation1992; Nordenfors, Citation2000).
Once a host has been located, D. gallinae prefer to feed from the back and the neck of the hen, accessing these feeding sites by traversing the housing structure of the facility and climbing up the bird legs or by dropping from the ceiling (Maurer et al., Citation1988). After taking a blood meal, the mites actively return to their refugia where they rest (Wood, Citation1917), digest the blood meal, mate and lay eggs (Sikes & Chamberlain, Citation1954; Axtell & Arends, Citation1990).
In addition to navigating on- and off-host within a poultry facility, D. gallinae are known to spread between facilities. This is typically achieved via mechanical vectoring as opposed to active movement, for example by transport on people (PRM can be found in clothes, boots, hair), brooms, rearing hens, crates, containers and egg trays (Mul & Koenraadt, Citation2009).
Dermanyssus gallinae can thus be considered to be a relatively mobile species, reliant on regular active and passive movement to develop within and move between facilities. With this in mind, recent studies have focused on new methods and strategies to reduce D. gallinae population growth by hindering the movement of mites, or disrupting their typical movement patterns by reducing their available hiding places (Mul and Van Weeghel, Citation2017). Several approaches have been discussed to this end, including the obstruction of the mites’ walking routes with high-velocity air flows, slippery or sticky surfaces and by use of moving water. In order to better develop and target such approaches, an understanding of the mechanics of mite movement and its tolerance to disruption is vital.
In previous work, Mul et al. (Citation2016) reported the ability D. gallinae to climb upwards on slippery surfaces made of glass or polytetrafluoroethylene (PTFE), also determining the air velocity at which these mites were no longer able to adhere to a vertically-orientated tube. Remarkably, adult D. gallinae were able to withstand a steady air velocity of up to 20.3 ± 12.2 m/s before being dislodged, while, upon a sudden release of air, all D. gallinae life stages were dislodged at an air velocity of 47.6 ± 0.1 m/s. These observations suggest that D. gallinae possess morphological adaptations that enable them to adhere to slippery surfaces and resist relatively high air fluxes, likely evolved to aid both active and passive movement patterns displayed in this species. In order to identify optimal ways to reduce the ability of D. gallinae to spread in poultry facilities, it is important to clarify the functional morphology of the mites’ legs to better understand how they adhere to and traverse the substratum types they encounter.
In general, mite legs are divided into several segments and each leg terminates with an ambulacrum which contacts the substrate (Evans, Citation1992; Alberti & Coons, Citation1999). This ambulacrum is comprised of various components, the development and arrangement of which differ between species depending on the habitat or substratum preferred by the taxon. Obtaining a detailed knowledge of the morphology of the distal leg region (ambulacrum) in D. gallinae could be a vital step towards an explanation of the attachment mechanisms of this mite and could inform new methods for improved control of this species. Unfortunately, studies of the ambulacra in dermasyssoid mites are rare (Evans & Till, Citation1965, Citation1979; Evans, Citation1992; Alberti & Coons, Citation1999; Krantz, Citation2009; Montasser, Citation2006), with no such work detailing this feature in D. gallinae. Hence, the aim of this paper is to provide a detailed description of the morphological and functional adaptations of the leg ambulacra for all instars of D. gallinae, whilst also applying this knowledge in an attempt to explain the ability of this mite to traverse slippery surfaces, resist air flows, cling onto materials for transport, move across different types of substrates (e.g. hen house substructures and feathers) and secure itself to soft surfaces such as the skin of its avian hosts.
Materials and methods
Dermanyssus gallinae specimens used in the current study were sourced from a farm located in the Dutch province of Gelderland. Mites were collected from under the horizontal fixed u-profiles of the aviary housing system using a fine brush, and stored for transport to the laboratory at Wageningen in a vial with a lid. In the laboratory, the collected mites were placed into vials containing 70% ethanol. The mites were then sent to the laboratory at Foggia University, where they were prepared for observation by scanning electron microscopy (SEM).
When at Foggia, mites were dehydrated through a graded ethanol series (80%, 90%, 100%), dried using a Baltec CPD030 critical point dryer, mounted on SEM stubs using conductive carbon adhesive tabs and sputter-coated with palladium-gold using a Baltec SCD005 coating apparatus. Specimens were then examined and micro-graphed with a Hitachi TM3030 scanning electron microscope, equipped with a digital camera.
Results and discussion
Imaging of the legs of D. gallinae confirmed that these are divided, as in other mites (Evans, Citation1992; Alberti & Coons, Citation1999; Krantz, Citation2009), into seven segments ((a)). From the proximal to the distal region these are the coxa, trochanter, femur, genu,Footnote1 tibia, tarsus and ambulacrum, which contacts the substrate. In all instars of D. gallinae the ambulacrum features a praetarsus (the peduncle-like extension), two claws (the apotele) and a central pulvillus ((b)), providing a compound ambulacrum typical of Anactinotrichida (Alberti & Coons, Citation1999) where all elements are basically present.
Figure 1. Dermanyssus gallinae: (a) nymph: third leg clearly displaying seven segments; (b) larva: dorso-lateral view of the ambulacrum of the second leg with the praetarsus (the peduncle-like extension), two claws and the central pulvillus visible; (c) nymph: lateral view of the ambulacrum of the third leg with the pulvillus and the claws completely retracted into the praetarsus and the paradactyli covering them; (d) nymph: lateral view of the ambulacrum of the first leg showing the pulvillus completely inflated, the claws laterally located and the absence of the paradactyli. Abbreviations: Am: ambulacrum; cl: claw; Cx: coxa; Fe: femur; Ge: genua; pd: paradactylus; pr: praetarsus; pv: pulvillus; Ta: tarsus; Ti: tibia; Tr: trochanter. Scale bars: 50 µm (a); 10 µm (b–d).
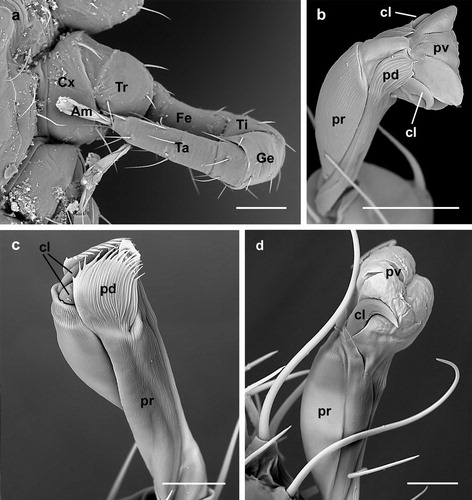
The pulvillus is a lobate weakly sclerotized structure situated ventrally between the claws, which themselves appear strongly sclerotized ((b)). Laterally to the claws, a pair of denticulate processes (paradactyli) (Evans & Till, Citation1965, Citation1979) are present ((b,c)) in all ambulacra, except those of the first pair of legs, and in all mite instars. It is reported from other species that the ambulacra of the first pair of legs are often reduced or lost, reduction usually being seen in the size of the pulvillus and claws (Evans, Citation1992; Krantz, Citation2009). In D. gallinae, however, no such reduction is observed; in fact, both the claws and pulvillus are normally present and shaped in the first pair of legs ((d)), the only difference being that the paradactyli are absent.
The pulvillus of D. gallinae shows an enlarged lobate shape when it is completely inflated ((b,d) and (a,b)). In this state the pulvillus is the first component of the ambulacrum to contact the substratum and, hence, it functions as a sucker to adhere to a smooth surface.
Figure 2. Dermanyssus gallinae: detail of the ambulacra with the pulvilli completely inflated; (a) male: ambulacrum of the first leg, ventro-lateral view (note that pulvillus and claws are well developed while the paradactyli are absent in the first leg); (b) nymph: ambulacrum of the third leg, dorsal view. In both (a) and (b) the pulvilli are completely inflated and lobate in shape; in this state these structures contact the substratum while the claws do not. Abbreviations: cl: claw; pd: paradactylus; pr: praetarsus; pv: pulvillus. Scale bars: 20 µm (a); 10 µm (b).
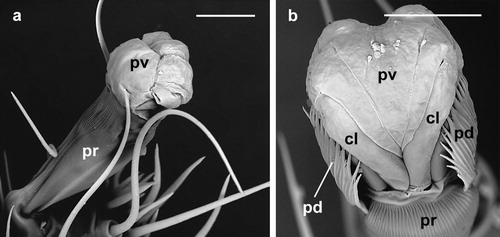
A similar arrangement has been reported in the female of Varroa destructor (Anderson & Trueman, 2000) (Acari, Dermanyssoidea, Varroidae), where the pulvilli are extremely large, thus providing adhesion to the cuticle of the bee host, while the claws are reduced (Alberti & Coons, Citation1999). The same adaptation has also been observed in the legs of Chirodiscoides caviae Hirst (Acari, Sarcoptoidea, Atopomelidae), where ambulacral discs (pulvilli) aid attachment to the hairs of their hosts (Alberti & Coons, Citation1999). This last example is particularly intriguing since hairs in mammals and feathers in birds are relatively similar in composition, even though they are visually distinct. Moreover, cuticle, hairs and feathers have a compact and smooth surface, so it is reasonable to assume that mites needing to move on similarly smooth surfaces have developed similar adaptions to do so.
Dermanyssus gallinae does not possess reduced claws, as in V. destructor, thus, allowing it to switch from using the pulvillus as an adhering structure, to utilizing these claws to cling to the substrate. From work in other dermanyssoids, it is known that retraction and protraction of the pulvillus and claws is “controlled” by the mite and realized by to two antagonistic muscles, the pulvillar retractor (originating in the tarsus segment of the leg) and the basilar protractor (originating in the tibia segment of the leg) (Evans & Till, Citation1965, Citation1979). As in these species, the pulvillus can be withdrawn in D. gallinae, together with the claws, into the praetarsus (the peduncle-like extension of the ambulacrum), as visualized in several specimens where the pulvillus and the claws appear partly or fully retracted in the ambulacrum stalk (). The paradactyli (the denticulate processes) are not retracted and, when the ambulacrum is completely withdrawn, they cover the claws and the pulvillus ((c) and 3(a)).
Figure 3. Dermanyssus gallinae: ambulacra; (a) female: dorsal view of the ambulacrum of the third leg with the pulvillus and claws partly retracted in the praetarsus and surrounded by the paradactyli; (b) nymph: lateral view of the ambulacrum of the first leg with the pulvillus fully retracted while the claws are still visible (and would thus contact the substratum); (c) larva: ventral view of the ambulacrum of the first leg with the pulvillus and claws fully retracted in the praetarsus (ambulacrum stalk). Note the absence of the paradactyli in the first leg. Abbreviations: cl: claw; pd: paradactylus; pr: praetarsus; pv: pulvillus. Scale bars: 10 µm.
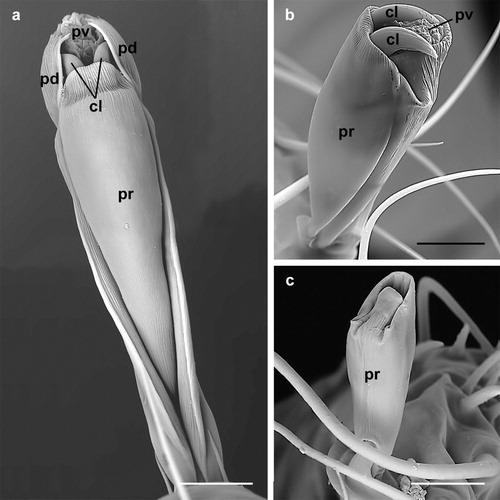
Retraction and protraction of the pulvilli and claws can be assumed to enable D. gallinae to utilize these features independently depending on the surface upon which the mite is moving. Furthermore, where D. gallinae needs to adhere to a smooth surface, as on feathers, ceilings, ventilation pipes, etc., it can inflate (protract) the pulvillus and use it as a sucker ((b,d) and 2); simultaneously, if these mites need to move on an irregular substratum, they can partly retract the pulvillus and utilize their claws ((b)) for more effective movement.
Aside from the locomotion, invertebrate legs serve several other functions that may strongly influence their organization (Alberti & Coons, Citation1999). A characteristic of D. gallinae’s behaviour is that it not only climbs up and down its host, but also navigates its surroundings. These “off-host” environments can be comprised of a wide range of materials, making it likely that this species has developed morphological adaptations of the ambulacra to permit effective movement over a diverse range of substrates. Such adaptations would have initially evolved in “wild-living” D. gallinae but would also prove beneficial for movement within poultry facilities. Maurer et al. (Citation1988), for example, report the ability of D. gallinae to walk upside down on the ceiling, while, according to Mul et al. (Citation2016), this species can also climb vertically upwards on a dry and clean wall with a very low friction coefficient. Also, as noted earlier, Mul et al. (Citation2016) report that all D. gallinae instars are able to resist an airflow on a vertically orientated tube. Whilst such capabilities would allow “wild” specimens to locate and adhere to birds nesting in trees, they serve equally well in allowing mites in managed poultry flocks to locate and adhere to perching hens.
The observed morphological adaptations of D. gallinae’s ambulacrum, and the ability of D. gallinae to respond to the surface they are on by utilizing either the pulvilli (acting as suckers) or the claws, explain why this species is able to climb upwards on a slippery surface, resist an air flux, walk upside down on the ceiling, navigate both the smooth and rigid feathers of their hosts, and cling to the soft skin of hens and other birds. Moreover, D. gallinae can use its claws to cling to human clothes, skin and hairs as proved by several cases reporting this species as the cause of dermatological problems in humans, both poultry workers and urban residents (Rosen et al., Citation2002; Cafiero et al., Citation2011).
In addition, research elsewhere suggests that D. gallinae exerts a high level of control over the functioning of the ambulacrum and its various structures. Mul et al. (Citation2016), for example, observed that mites “clamped onto” the inner surface of a tube when the air flow through that tube was slowly increased, and thus effectively “prepared” themselves for higher air speeds. Also, Maurer et al. (Citation1988) reported that D. gallinae walking upside down on a ceiling can “decide” when to release from the surface to drop down onto a hen below. Soler Cruz et al. (Citation2005) described the presence of several olfactory receptors, as well as mechanoreceptors, in the foreleg tarsal sense organs of D. gallinae. These receptors are represented by a cluster of 10 blunt peg-type sensilla divided into four possible chemoreceptors, two multimodal (both chemo- and mechanoreceptor) and four mechanoreceptors. Such sensilla could explain how these mites can perceive a nearby host or change in airflow (respectively), allowing them to respond accordingly – in this case by either increasing adhesion to a surface (i.e. by expanding the pulvilli of the ambulacra), or dropping from it (i.e. by retracting the pulvilli).
In terms of practical application, this work should prove useful in informing several areas of D. gallinae management. It has been speculated elsewhere, for example, that D. gallinae might be able to use ventilation systems to move around poultry facilities (Mul et al., Citation2009), with the above observations supporting this hypothesis and the current work demonstrating that these mites certainly possess morphological adaptions that would facilitate movement through these structures. As well as potentially helping to identify “mite-friendly” features within facilities that could be “designed out” for improved D. gallinae control, this work also suggests that current research into physical management methods, such as brushing, blowing and rinsing of mites from surfaces, or any exclusion method based on the use of smooth materials as barriers to mite movement, should carefully consider the potential of D. gallinae to naturally resist such interventions.
Acknowledgement
The authors sincerely thank Dr D. R. George, Stockbridge Technology Centre, United Kingdom, for English language checking on an initial draft of the manuscript.
Disclosure statement
No potential conflict of interest was reported by the authors.
ORCID
Antonella Di Palma http://orcid.org/0000-0002-9502-9820
Additional information
Funding
Notes
1. Genu: term which is used for the “knee-segment” in the acarological literature, instead of patella.
References
- Alberti, G. & Coons, L.B. (1999). Acari – Mites, In F.W. Harrison & R.F. Foelix (Eds.). Chelicerate Arthropoda (pp. 515–1265). New York, NY: John Wiley & Sons.
- Axtell, R.C. & Arends, J.J. (1990). Ecology and management of arthropod pests of poultry. Annual Review of Entomology, 35, 101–126. doi: 10.1146/annurev.en.35.010190.000533
- Cafiero, M.A., Galante, D., Camarda, A., Giangaspero, A. & Sparagano, O. (2011). Why dermanyssosis should be listed as an occupational hazard. Occupational and Environmental Medicine, 68, 628. doi: 10.1136/oemed-2011-100002
- Chauve, C.M. (1998). The poultry red mite Dermanyssus gallinae (De Geer, 1778): current situation and future prospects for control. Veterinary Parasitology, 79, 239–245. doi: 10.1016/S0304-4017(98)00167-8
- Chirico, J., Eriksson, H., Fossum, O. & Jansson, D. (2003). The poultry red mite, Dermanyssus gallinae, a potential vector of Erysipelothrix rhusiopathiae causing erysipelas in hens. Medical and Veterinary Entomology, 17, 232–234. doi: 10.1046/j.1365-2915.2003.00428.x
- Evans, G.O. (1992). Principles of Acarology. Wallingford: C.A.B. International.
- Evans, G.O. & Till, W.M. (1965). Studies on the British Dermanyssidae (Acari: Mesostigmata). part I. External morphology. Bulletin of the British Museum (Natural History), 13, 247–294. doi: 10.5962/bhl.part.16752
- Evans, G.O. & Till, W.M. (1979). Mesostigmatic mites of Britain and Ireland (Chelicerata: Acari: Parasitiformes). An introduction to their external morphology and classification. Transactions of the Zoological Society of London, 35, 139–270. doi: 10.1111/j.1096-3642.1979.tb00059.x
- Fossum, O., Jansson, D.S., Etterlin, P.E. & Vågsholm, I. (2009). Causes of mortality in laying hens in different housing systems in 2001 to 2004. Acta Veterinaria Scandinavica, 15, 51–53.
- George, D.R., Finn, R.D., Graham, K.M., Mul, M.F., Maurer, V., Valiente-Moro, C. & Sparagano, O. (2015). Should the poultry red mite Dermanyssus gallinae be of wider concern for veterinary and medical science? Parasites & Vectors, 8, 178. doi: 10.1186/s13071-015-0768-7
- Kilpinen, O., Roepstorff, A., Permin, A., Nørgaard-Nielsen, G., Lawson, L.G. & Simonsen, H.B. (2005). Influence of Dermanyssus gallinae and Ascaridia galli infections on behaviour and health of laying hens (Gallus gallus domesticus). British Poultry Science, 45, 26–34. doi: 10.1080/00071660400023839
- Krantz, G.W. (2009). Form and function. In G.W. Krantz & D.E. Walter (Eds.). A Manual of Acarology 3rd edn (pp. 5–53). Lubbock: Texas Tech University Press.
- Maurer, V. & Baumgärtner, J. (1992). Temperature influence on life table statistics of the chicken mite Dermanyssus gallinae (Acari: Dermanyssidae). Experimental and Applied Acarology, 15, 27–40. doi: 10.1007/BF01193965
- Maurer, V. & Baumgärtner, J. (1994). A population model for Dermanyssus gallinae (Acari, Dermanyssidae). Experimental and Applied Acarology, 18, 409–422. doi: 10.1007/BF00051523
- Maurer, V., Bieri, M. & Fölsch D.W. (1988). Das Suchverhalten von Dermanyssus gallinae in Hühnerställen. Archiv für Geflügelkunde, 52, 209–215.
- Montasser, A.A. (2006). Scanning electron microscopy observations on male Echinolaelaps echidninus (Berlese, 1887) (Dermanyssoidea: Laelapidae) with emphasis on its gnathosoma, palpal organ and pulvilli. International Journal of Zoological Research, 2, 204–212. doi: 10.3923/ijzr.2006.204.212
- Moro, C. V., Fravalo, P., Amelot, M., Chauve, C., Zenner, L. & Salvat, G. (2007). Colonization and organ invasion in chicks experimentally infected with Dermanyssus gallinae contaminated by Salmonella Enteritidis. Avian Pathology, 36(4), 307–311. doi: 10.1080/03079450701460484
- Mul, M. & Van Weeghel, E. (2017). A structured design approach with focus on the PRM problem in laying hen facilities. COREMI design session, April 12th 2017, London, UK. COST Action FA1404. https://www.coremi.eu/fileadmin/documents_organicresearch/coremi/4-8_Design_approach_meeting_summary.pdf (retrieved: 15 February 2019).
- Mul, M., van Niekerk, T., Chirico, J., Maurer, V., Kilpinen, O., Sparagano, O., Thind, B., Zoons, J., Moore, D., Bell, B., Gjevre, A.-G. & Chauve, C. (2009). Control methods for Dermanyssus gallinae in systems for laying hens: results of an international seminar. World's Poultry Science Journal, 6, 589–600. doi: 10.1017/S0043933909000403
- Mul, M.F. & Koenraadt, C.J.M. (2009). Preventing introduction and spread of Dermanyssus gallinae in poultry facilities using the HACCP method. Experimental and Applied Acarology, 48, 167–181. doi: 10.1007/s10493-009-9250-6
- Mul, M.F., Ploegaert, J.P.M., George, D.R., Meerburg, B.G., Dicke, M. & Groot Koerkamp, P.W.G. (2016). Structured design of an automated monitoring tool for pest species. Biosystems Engineering, 151, 126–140. doi: 10.1016/j.biosystemseng.2016.08.023
- Mul, M.F., Van Niekerk, T.G.C.M., Reuvekamp, B.F.J. & Van Emous, R.A. (2010). Dermanyssus gallinae in Dutch poultry farms: Results of a questionnaire on severity, control treatments, cleaning, and biosecurity. Trends in acarology: proceedings of the 12th international congress, 513–516.
- Nordenfors, H. (2000). Epidemiology and Control of the Poultry Red Mite, Dermanyssus gallinae. Doctoral thesis.
- Nordenfors, H., Höglund, J, & Uggla, A. (1999). Effects of temperature and humidity on oviposition, molting and longevity of Dermanyssus gallinae (Acari: Dermanyssidae). Journal of Medical Entomology, 36, 68–72. doi: 10.1093/jmedent/36.1.68
- Rosen, S., Yeruham, I., & Braverman, Y. (2002). Dermatitis in humans associated with the mites Pyemotes tritici, Dermanyssus gallinae, Ornithonyssus bacoti and Androlaelaps casalis in Israel. Medical and Veterinary Entomology, 16, 442–444. doi: 10.1046/j.1365-2915.2002.00386.x
- Sikes, R.K. & Chamberlain, R.W. (1954). Laboratory observations on three species of bird mites. Journal of Parasitology, 40, 691-697. doi: 10.2307/3273713
- Soler Cruz, M.D., Vega Robles, M.C., Jespersen, J.B., Kilpinen, O., Birkett, M., Dewhirst, S. & Pickett, J. (2005). Scanning electron microscopy of foreleg tarsal sense organs of the poultry red mite, Dermanyssus gallinae (DeGeer) (Acari: Dermanyssidae). Micron, 36, 415–421. doi: 10.1016/j.micron.2005.03.003
- Sommer, D., Heffels-Redmann, U., Köhler, K., Lierz, M. & Kaleta, E.F. (2016). Role of the poultry red mite (Dermanyssus gallinae) in the transmission of avian influenza A virus. Tierarztliche Praxis Ausgabe G: Grosstiere - Nutztiere, 44(1), 26-33. doi: 10.15653/TPG-150413
- Sparagano, O.A.E. & Giangaspero, A. (2011). Parasitism in egg production systems: the role of the red mite (Dermanyssus gallinae). In Y. Nys, M. Bain, & F. Van Immerseel (Eds.). Improving the safety and quality of eggs and egg products: Egg chemistry, production and consumption (Volume 1, pp. 394–414). Cambridge: Woodhead Publishing Series in Food Science, Technology and Nutrition.
- Tucci, E.C. & Guimaraes, J.H. (1998). Biology of Dermanyssus gallinae (De Geer, 1778) (Acari: Dermanyssidae). Revista Brasileira de Parasitologia Veterinaria, 7, 27–30.
- Valiente-Moro, C., Chauve, C. & Zenner, L. (2005). Vectorial role of some Dermanyssoid mites (Acari, Mesostigmata, Dermanyssoidea). Parasite, 12, 99–109. doi: 10.1051/parasite/2005122099
- Valiente-Moro, C., Chauve, C. & Zenner, L. (2007). Experimental infection of Salmonella Enteritidis by the poultry red mite, Dermanyssus gallinae. Veterinary Parasitology, 146, 329–336. doi: 10.1016/j.vetpar.2007.02.024
- Van Emous, R.A., Fiks-van Niekerk, T.G.C.M. & Mul, M.F. (2005). Bloedluizen (vogelmijten) op papier en in de praktijk. Praktijk Rapport 17, Animal Sciences Group – Praktijkonderzoek ISSN 1570 8624 (In Dutch).
- Wood, H.P. (1917). The chicken mite: its life history and habits. Washington, DC: U.S. Dept. of Agriculture, p. 553.