ABSTRACT
Infectious laryngotracheitis (ILT) is a highly contagious respiratory disease of chickens, pheasants, and peafowl. It is caused by the alpha herpesvirus, infectious laryngotracheitis virus (ILTV). Glycoprotein D (gD) of ILTV is immunogenic and helps in its binding to the susceptible host cell receptor. In the present study, a recombinant gD protein was expressed in a prokaryotic system to develop a single serum dilution ELISA. In addition, two immunogenic peptides, corresponding to regions 77–89 and 317–328, were identified in gD protein. The peptides were synthesized using solid-phase peptide synthesis, purified using reversed-phase HPLC, and characterized using mass spectrometry. The peptides displayed a good titre and were found to be promising antigens to coat the ELISA plate to detect the ILTV antibodies in the serum sample. The developed ELISA showed 96.9% sensitivity, 87.5% specificity, and 95.3% accuracy as compared to OIE referenced standard indirect ILTV ELISA (whole viral coated). The assay may not differentiate vaccinated from infected birds when the flocks are administered with live attenuated vaccines. However, the assay could be useful to detect the disease condition in birds vaccinated with recombinant vaccine expressing glycoproteins other than gD. The developed ILTV single serum dilution ELISA could be an alternative to the existing diagnostics for the detection of ILTV antibodies.
Introduction
Infectious laryngotracheitis (ILT) is a highly contagious upper respiratory disease of chickens, pheasants, and peafowl (Bagust et al., Citation2000; Garcia et al., Citation2013). ILT causes production losses due to increased morbidity and mortality, decreased weight gain, and predisposition to other respiratory pathogens (Guy & Bagust, Citation2003; Garcia et al., Citation2013). Clinical signs of ILT include nasal discharge, moist rales, coughing, gasping, and decrease in egg production (Bagust, et al., Citation2000). ILT outbreaks have been reported in the United States (Dormitorio et al., Citation2013), Canada (Ojkic et al., Citation2006), Brazil (Parra et al., Citation2015), Europe (Neff et al., Citation2008), Australia (Agnew-Crumpton et al., Citation2016), China (Yan et al., Citation2016), and South Asia (Hidalgo, Citation2003; Chacon & Ferreira, Citation2009; Gowthaman et al., Citation2016). From 2000–2013, the disease was reported in many countries across the globe (Menendez et al., Citation2014).
ILT is caused by infectious laryngotracheitis virus (ILTV), also known as Gallid herpesvirus 1 (GaHV-1). ILTV belongs to the family Herpesviridae and genus Iltovirus (Roizman, Citation1982). It contains a double-stranded DNA molecule within an icosahedral capsid, which is surrounded by a proteinaceous tegument layer and an outer envelope containing viral glycoproteins (Cruickshank et al., Citation1963; Watrach et al., Citation1963). The genomic DNA of ILTV is approximately 155 kb in size and consists of unique long and short segments (UL and US) flanked by inverted repeats (Leib et al., Citation1987; Johnson et al., Citation1991). The envelope contains major immunogenic glycoproteins namely, gB, gC, gD, gE, gG, gH, gI, gJ, gK, gL, and gM, which are encoded by UL27, UL44, US6, US8, US4, UL22, US7, US5, UL53, UL1, and UL10, respectively (York et al., Citation1987; York et al., Citation1990). The envelope glycoproteins of herpesviruses are required for virus entry, cell to cell fusion, and virus egress (Spear et al., Citation2000; Pereira, Citation1994). The gB protein of herpesviruses is involved in membrane fusion and virus penetration (Dunaevskaia, Citation1988; Connolly et al., Citation2011). On the other hand, gD protein of most herpesviruses acts as a receptor for virus binding to susceptible cells (Di Giovine et al., Citation2011; Eisenberg et al., Citation2012). Both gB and gD proteins produce neutralizing antibodies upon virus infection (Rouse & Kaistha, Citation2006; Lazear et al., Citation2012). In addition, both gB and gD proteins of herpesvirus have been shown to be the target antigens for recombinant vaccine production (Stanberry et al., Citation2002; Rouse & Kaistha, Citation2006; Lazear, et al., Citation2012; Zhao et al., Citation2014). The gB and gD proteins are highly conserved structural glycoproteins of herpesviruses. Moreover, gD protein of ILTV is suggested to be a better antigen for the development of ELISA than gB (Kanabagatte Basavarajappa et al., Citation2014, Citation2015). The immunization of gD shows a higher neutralizing antibody response as compared to gB alone and in combination with other glycoproteins of ILTV (Kanabagatte Basavarajappa, et al., Citation2014).
Largely due to the lack of knowledge regarding its epidemiology, pathogenesis, and immune response in poultry, ILTV infection is a major threat to the poultry producing regions of the world (Hughes et al., Citation1991; Kotiw et al., Citation1995). Chicken embryo origin (CEO) and tissue culture origin (TCO) vaccines developed during 1960s have been extensively used for controlling ILT outbreaks worldwide. The recombinant vaccines expressing surface glycoproteins of ILTV were used with varying efficacy (Zhao, et al., Citation2014; Davidson et al., Citation2016). Although gD protein has been explored as a diagnostic tool using NDV as a vector, the use of recombinant protein or its immunogenic peptides has not been reported.
In the present study, prokaryotic expressed gD protein of ILTV was used to evaluate ILTV-specific antibodies from flocks showing disease. In addition, bioinformatic tools were used to map the linear B-cell epitopes of gD protein of ILTV and predict the antigenic peptides. Two peptides of gD protein were used to develop an indirect ELISA for the detection of anti-ILTV antibodies in serum from infected chickens. The proposed ELISA could be used as an alternative to screen for ILTV antibodies in the serum samples. The results suggest the use of gD and its peptides in place of whole virus as an antigen.
Materials and methods
Construction and expression of gD protein of ILTV
The gD protein gene of ILTV (accession number NC_006623) was cloned into prokaryotic expression vector pET28a (Novagen, Germany) flanked by EcoRI and XhoI restriction sites. The integrity of the cloned gD protein gene was confirmed by restriction digestion and nucleotide sequencing. The pET28a vector containing gD protein gene was transformed into Escherichia coli BL21 (DE3)pLysS and recombinant protein was produced following a standard protocol (Kumar et al., Citation2016). The concentration of the purified gD protein was determined by a protein assay kit (Pierce, NY, USA) and confirmed by SDS-PAGE and western blot analysis.
Generation of antisera against the recombinant gD protein
In order to prepare polyclonal antibodies, three 4-week-old SPF chickens (Venkateshwara Hatcheries, Pune, India) were immunized subcutaneously with 0.25 mg of purified recombinant gD protein emulsified in Freund’s complete adjuvant (Sigma, MO, USA). Subsequently, two booster injections were given by purified recombinant gD protein with incomplete adjuvant (Sigma, USA) at an interval of 21 days. The serum samples were collected 21 days after final injection and stored for further use. All experimental procedures were performed in compliance with the guidelines of institutional animal use and institutional biosafety committees. The antibody against recombinant gD protein was confirmed by western blot using goat anti-chicken IgG conjugated to horseradish peroxidase (Thermo Scientific, MA, USA) as well as by commercial ILTV kit (IDEXX, ME, USA).
Design, synthesis and characterization of gD peptides
The linear B cell epitopic regions of the gD protein of ILTV were predicted by Immune Epitope Database Analysis Resource (IEDB) (Vita et al., Citation2010; Sun et al., Citation2013). The gD protein was analyzed for its physicochemical properties such as hydrophilicity, flexibility, and exposed surface using DNAstar software (Hopp & Woods, Citation1981). In addition, the gD protein was also analyzed for its property towards surface probability, beta-turn, antigenicity and hydrophilicity (Figure S1). The predicted peptide sequences, 77SAAKEKEKGGPFE89 towards N-terminal region and 317TEHDDPNSDPDY328 towards C-terminal region, were named gDn and gDc, respectively. The peptides were synthesized manually on Rink amide resin by employing Fmoc chemistry. The coupling was achieved using N,N,N′,N′-Tetramethyl-O-(1H-benzotriazol-1-yl)uronium hexafluorophosphate (HBTU), 1-Hydroxybenzotrizole (HOBt) and N,N-Diisopropylethylamine activation as described elsewhere (Fields & Noble, Citation1990; Datta et al., Citation2019). Both the peptides were acetylated at the N-terminus using five equivalents of acetic anhydride and ten equivalents of DIPEA. The synthesized peptides were cleaved from the resin by 12–14 h incubation in a cleavage cocktail containing trifluoroacetic acid, m-cresol, thioanisole, and ethanedithiol (20:2:2:1). The cleaved peptides were precipitated in ice-cold diethyl ether, washed 5–6 times with diethyl ether to remove the scavengers, and air-dried. The dried peptides were dissolved in water and purified using reversed-phase HPLC on a C18 column using a linear gradient of acetonitrile. The purified peptides were characterized using MALDI-TOF mass spectrometry.
Collection of serum samples
A total of 193 serum samples were screened for ILTV antibodies from different farms in and around Guwahati, Assam, India. Serum samples of suspected broiler birds (4-8 weeks) were collected under aseptic conditions and stored at −20°C until use. In addition, the tissue samples were collected from the birds showing clinical signs of ILT infection. The post mortem tissue samples were found positive for ILTV genome upon PCR using thymidine kinase gene-specific primers (Kirkpatrick et al., Citation2006). There was no history of ILTV vaccination to the birds while regular vaccination against Newcastle disease and infectious bursal disease was done. In addition, serum samples were screened negative for Newcastle disease, avian influenza, and infectious bronchitis using a commercial kit (IDEXX, ME, USA).
Development of ELISA using recombinant gD and its peptides
The recombinant gD protein and synthesized peptides were incubated with carbonate and bicarbonate buffer (pH 9.6) at 4°C overnight. The wells were blocked with 2% BSA and incubated with serum samples. Chicken serum samples were diluted to 1:100 in PBS and further two-fold dilutions were made serially up to 211. The final dilution was added in duplicate to antigen-coated wells and incubated at 37°C for one hour. The optimal concentrations of gD protein and peptides were determined by the checkerboard titration method as described earlier (Robinson et al., Citation1985; Kumar, et al., Citation2016).
The positive/negative threshold (PNT) line was constructed using 20 negative serum samples collected from SPF chickens, and mean absorbance plus three standard deviations was calculated for each sample (Snyder et al., Citation1983). The resultant PNT line was used to determine the observed antibody titres of positive serum samples by the subtraction method as described earlier (Snyder, et al., Citation1983; Ramadass et al., Citation2008). The absorbance of the collected serum samples from the field was then corrected by subtracting from each serial absorbance reading of internal negative control. Observed ELISA titres were determined by computing the intersection with the PNT baseline.
Observed antibody titres were subjected to separate logarithmic regression analysis (log absorbance vs. log dilution) against their corresponding corrected test absorbance at a single antiserum dilution. The log of the corrected absorbance value at a single working dilution of the various test sera was plotted against the log of the observed antiserum titre after interpolation. Titres could then be predicted with the corrected absorbance from a single test dilution by means of the regression line equation or a standard curve. Regression analysis was performed on this relationship for all data treatment methods at several working dilutions. The regression line equation gives the linear relationship between the observed and predicted antibody titres. The highest correlation coefficient corresponds to a highly significant relationship between observed and predicted antibody titres at a particular dilution. The dilution which gives the highest correlation coefficient is used to predict the antibody titre of other test samples. The absorbance values obtained for every logarithmic dilution were compared with observed titre and the highest correlation coefficient was selected to calculate the titre from that dilution. Further, antibody titres could be satisfactorily predicted with corrected absorbance readings from a single serum dilution by using a regression line equation (for a given dilution) of the form: log10 titre = (y-intercept – log10absorbance) / slope (Snyder, et al., Citation1983). The observed antibody titres were compared in the highest correlation coefficient as described earlier (Snyder, et al., Citation1983). The sensitivity, specificity, and accuracy of the single dilution assay were compared with the commercially available indirect ELISA kit from BioChek, ME, USA (York et al., Citation1983; York & Fahey, Citation1988).
Results
Construction and expression of gD protein of ILTV
The PCR amplified product of gD protein gene of ILTV was cloned into pET28a prokaryotic vector flanked with EcoRI and XhoI. The restriction digestion showed the release of the cloned fragment after digestion with EcoRI and XhoI ((A)). Furthermore, the nucleotide sequencing of the cloned recombinant gD protein gene confirmed its identity. The recombinant gD protein expressed in BL21 cells as a fusion protein with His tag showed a band of approximately 50 kDa ((B)). The recombinant gD protein was further confirmed by western blot using polyclonal gD and anti-His antibodies ((C)). The average yield of the different fractions of the recombinant gD protein was 0.8 mg/ml. The purified gD protein was concentrated using ultra concentrator filter of 10 kDa cut-off (Merck, Germany) for its immunological characterization.
Figure 1. Cloning and expression analysis of recombinant gD protein of Infectious laryngotracheitis virus (ILTV). The cloning of gD protein of ILTV as confirmed by restriction digestion and PCR amplification (A). Lane 1: marker; lane 2: undigested pET28a; lane 3: EcoRI and XhoI digested pET28a; lane 4: gD gene cloned pET28a; lane 5: EcoRI and XhoI digested gD gene cloned pET28a; lane 6: PCR amplification of 1065 nt gD gene fragment. The SDS-PAGE (B) and western blot analysis (C) of the recombinant gD protein of ILTV expressed in a prokaryotic expression vector. L: protein molecular weight marker; U: uninduced recombinant pET28a containing gD protein gene; I: expression of the protein in recombinant pET28a containing gD protein gene after 4 h of 0.5 mM IPTG induction; P: purified gD protein.
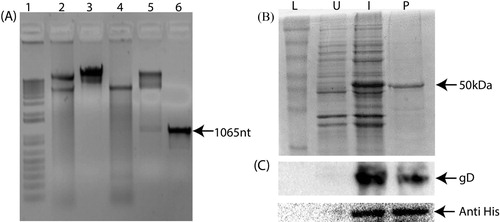
Characterization of gD peptides
The Immune Epitope Database (IEDB) predicted 13 peptides of different lengths on the basis of sequence characteristics of the antigen using amino acid scales and Hidden Markov Model (HMMs) (Figure S1). Based on the predicted epitopic propensity and physicochemical properties of amino acids, we chose two peptides; one towards the N-terminus and the other towards the C-terminus of gD protein. The HPLC chromatograms of the purified peptides are shown in . The identity of the peptides was ascertained using MALDI-TOF mass spectrometry. The calculated monoisotopic masses of gDn and gDc peptides are 1417.71 and 1444.53 Da, respectively. The m/z peaks for MH+ ions of gDn and gDc were observed at 1419.65 and 1446.04, respectively.
Development of ELISA
The checkerboard titration of purified recombinant gD protein showed 1 µg/well of protein was optimum for the working range. In addition, the optimum amount of gDn and gDc peptides was calculated to be 10 µg/well. The PNT baseline was constructed by the mean plus three standard deviations (M + 3SD) values of the eight different negative serum samples ((A)). The observed titre (end-point) was determined as the dilution at which the sample absorbance crosses the PNT line. The highest correlation coefficient (0.906) was calculated at a dilution of 1:400 ((B)). Subsequently, a dilution of 1:400 was used to predict the titres of serum samples using all the three antigens. The slope and intercept were 16.27 and 0.105, respectively. The observed and predicted OD for all the serum samples were calculated using the regression equation, observed OD = 16.27 (predicted OD) + 0.105. A linear relation was observed between the predicted and observed titres. All sera that were positive by recombinant gD protein ELISA were also positive in gD peptide ELISA. The comparison of recombinant gD protein and peptide ELISA using reference sera indicated that both the peptides were capable of identifying ILTV-infected positive and uninfected negative sera samples (). The absorbance of gDn peptide was recorded higher than gDc in the tested serum samples. However, the titre of gDn peptide was recorded lower than complete gD protein in all the tested serum samples.
Figure 3. The standard serial dilution method of determining observed ILTV ELISA antiserum titres from corrected absorbance with positive–negative threshold (PNT) baseline (A). The relationship between the observed antibody titres of the serum samples obtained after serial dilution and their corresponding predicted antibody titres obtained from a single dilution ELISA at a 1:400 dilution (B).
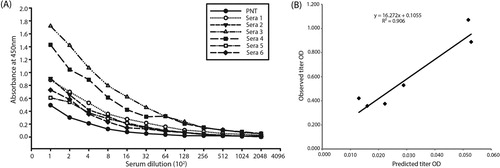
Figure 4. The recombinant glycoprotein D and its peptides (gDn and gDc) were coated alone and in combination for detecting the absorbance of the ILTV serum sample. The absorbance value of the negative serum samples was subtracted in order to get the actual absorbance of the ILTV positive samples. The ILTV ELISA having full-length gD shows higher absorbance as compared to gDn and gDc alone and in combination.
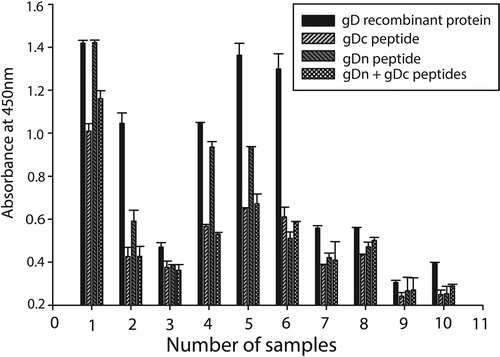
The sensitivity, specificity, and accuracy of the assay relative to the standard diagnostic ELISA are shown in . The sensitivity, specificity, and accuracy of recombinant gD protein-based ELISA were calculated 96.9%, 87.5%, and 95.3%, respectively.
Table 1. Relative sensitivity, specificity and accuracy of the developed recombinant glycoprotein D of infectious laryngotracheitis virus (ILTV) based ELISA to detect antibodies. Serum samples of 193 broiler birds (4–8 weeks) suspected for ILTV antibodies were collected under aseptic conditions and stored at −20°C until use. The serum samples were screened negative for Newcastle disease, avian influenza and infectious bronchitis using a commercial kit (IDEXX, USA).
Discussion
The surface glycoproteins of ILTV have been shown to have virus neutralization activity (Zhao, et al., Citation2014; Kanabagatte Basavarajappa, et al., Citation2015). The detection of ILTV in field conditions requires a rapid and simple serological method. The gD protein-based ELISA has been shown to detect ILTV-specific antibodies (Kanabagatte Basavarajappa et al., Citation2015). In view of the above facts, gD protein of ILTV was expressed using a bacterial expression system and the purified protein was used as an antigen for diagnostic ELISA. Recombinant protein-based serological tests are considered to have higher sensitivity and specificity as the target antigen is immuno-dominant and devoid of any non-specific moieties present in whole cell preparations. We have successfully cloned and expressed the gD protein of ILTV using the bacterial expression system. In addition, the peptide-based ELISA for the detection of ILTV showed promising results but their efficacy was found lower than the complete recombinant gD protein. Perhaps other epitopic regions of gD might be responsible for the generation of antibody which is recognized by full-length protein but by gDn and gDc peptides alone. Peptide-based ELISA has been developed for monkeypox virus (Dubois et al., Citation2012), HIV (Alcaro et al., Citation2003), infectious bronchitis virus (Ding et al., Citation2015), avian infectious bronchitis virus (Lougovskaia et al., Citation2002), Aleutian mink disease virus (Ma et al., Citation2016), and human H5N1 influenza viruses (Velumani et al., Citation2011) with varying level of efficacy. However, the studies showed some limitation in context of peptide to be cross-checked for the serum antibody titre by western blot. The benefits of recombinant gD protein to be used for ELISA include ease of production in large amounts for large scale screening. Our results showed that the gDn and gDc peptides are equally able to differentiate ILTV-infected and non-infected serum samples. Further, combination of synthetic gDn and gDc peptides (5 µg/well of each) was found comparable to the individual peptides. However, it is possible that other combinations/ratios of the gDn and gDc peptides can maximize the absorbance. Our surface probability study of gDn showed higher value as compared to gDc which could be the reason for the better absorbance of the gDn-based ELISA as compared to gDc.
Although the actual biological activity of gD being a glycoprotein in the bacterial expressed form may not be justifiable in the absence of post-translational modification, its use in the diagnostics may have an added advantage. The high yield of gD protein (800 mg/l) obtained from bacterial culture in this study suggests its efficient production. Our study shows that gD protein-based ELISA can be used for rapid and efficient screening of a large number of serum samples in field condition. The gD peptides designed in the current study were based on the conserved sequence of the gD protein among the ILTV strains. The present ELISA could be a more effective way to detect the antibody titre in the serum samples as compared to the earlier report since the peptide could specifically react to a selected pool of antibody and not the whole gD (Kanabagatte Basavarajappa, et al., Citation2015).
The sensitivity and specificity of the gD protein-based ELISA were 96.9% and 87.5%, respectively, relative to the commercial indirect ELISA (BioChek, USA). The close correlation obtained between single serum and standard ELISA titre showed similar trends on exposure to ILTV-positive serum. The developed ELISA appears to be comparable with the standard ELISA test to detect ILTV antibodies from field samples (). However, the test does not differentiate the antibodies coming from vaccinated and infected birds. The reported ELISA using gD protein would help to overcome some of the economic, technical, and statistical constraints of detecting ILTV infection in poultry. The recombinant gD protein-based single serum dilution ELISA for the detection of antibodies developed in this study was shown to be sensitive, specific, and accurate as compared to the available standard ELISA. The results demonstrate the potential benefit of a simple, specific ELISA for anti-gD antibodies that may have diagnostic value for the poultry industry. However, further optimization of the ELISA using more negative and positive serum samples would give a more accurate picture of the assay.
The assay may not differentiate vaccinated from infected birds when the flocks are vaccinated with live-attenuated vaccines. Inability to differentiate birds infected and vaccinated with wild-type and/or recombinant vaccine expressing gD is the major limitation of the present study. The study emphasizes the development of gD peptide-based screening of ILTV antibodies in the serum samples. Presumably, the assay could be useful to detect the disease condition in birds vaccinated with recombinant vaccine expressing glycoproteins gB and gI (Godoy et al., Citation2013). Moreover, the gG and gC gene-deleted live-attenuated vaccine showing encouraging results against ILTV infection could probably replace the traditional CEO and TCO vaccines (Devlin et al., Citation2007; Garcia et al., Citation2016). Furthermore, viral vectors expressing the immunogenic protein genes of ILTV were also explored thoroughly to generate vaccines (Kanabagatte Basavarajappa, et al., Citation2014; Zhao, et al., Citation2014; Gimeno et al., Citation2015). High sequence identity of the glycoproteins among different strains of ILTV is the major limitation in designing a unique peptide (Bagust, et al., Citation2000; Thureen & Keeler, Citation2006). Perhaps, identification of an alternative variant antigen could help to differentiate the wild-type strains of ILTV from the vaccine strain (Fuchs et al., Citation2007; Garcia, Citation2017). The study is a proof of concept for a peptide-based screening platform to detect the ILTV antibodies from the serum samples. The work could be further extended to find out a unique way to design the peptide in order to differentiate the wild-type strains of ILTV from the vaccine strain.
Our results show that peptide ELISA can be used as a screening tool to identify ILTV infection in poultry. The recombinant gD protein-based assay specificity can be validated by the western blot. The bacterial expressed recombinant gD protein is very useful for large scale screening by providing continuous access to an inexpensive reagent. It will be interesting to explore the possibility of using immunogenic proteins of ILTV, other than gD and gB, for diagnosis.
Supplemental Material
Download JPEG Image (594.7 KB)Acknowledgements
We are grateful to Dr Monika Koul for critical review of the manuscript. We are thankful to Dr K Puro, ICAR-NEH for help in chicken experiments.
Disclosure statement
No potential conflict of interest was reported by the authors.
ORCID
Vishnu Kumar http://orcid.org/0000-0001-5787-9632
Nitin Chaudhary http://orcid.org/0000-0003-3542-8157
Sachin Kumar http://orcid.org/0000-0002-8526-6013
Additional information
Funding
References
- Agnew-Crumpton, R., Vaz, P.K., Devlin, J.M., O'Rourke, D., Blacker-Smith, H.P., Konsak-Ilievski, B., & Noormohammadi, A.H. (2016). Spread of the newly emerging infectious laryngotracheitis viruses in Australia. Infection Genetics Evolution, 43, 67–73. doi:10.1016/j.meegid.2016.05.023
- Alcaro, M.C., Peroni, E., Rovero, P. & Papini, A.M. (2003). Synthetic peptides in the diagnosis of HIV infection. Current Protein & Peptide Science, 4, 285–290. doi: 10.2174/1389203033487117
- Bagust, T.J., Jones, R.C. & Guy, J.S. (2000). Avian infectious laryngotracheitis. Revue Scientifique et Technique de l'OIE, 19, 483–492. doi: 10.20506/rst.19.2.1229
- Chacon, J.L. & Ferreira, A.J.P. (2009). Differentiation of field isolates and vaccine strains of infectious laryngotracheitis virus by DNA sequencing. Vaccine, 27, 6731–6738. doi: 10.1016/j.vaccine.2009.08.083
- Connolly, S.A., Jackson, J.O., Jardetzky, T.S. & Longnecker, R. (2011). Fusing structure and function: a structural view of the herpesvirus entry machinery. Nature Reviews Microbiology, 9, 369–381. doi: 10.1038/nrmicro2548
- Cruickshank, J.G., Berry, D.M. & Hay, B. (1963). The fine structure of infectious laryngotracheitis virus. Virology, 20, 376–378. doi: 10.1016/0042-6822(63)90129-6
- Datta D, K.V., Kumar, S., Nagaraj, R. & Chaudhary, N. (2019). Hydrogel formation by an aromatic analogue of a β-amyloid fragment, Aβ16–22: a scaffold for 3D cell culture. ACS Omega, 4, 620–627. doi: 10.1021/acsomega.8b02771
- Davidson, I., Raibshtein, I., Altori, A. & Elkin, N. (2016). Infectious laryngotracheitis virus (ILTV) vaccine intake evaluation by detection of virus amplification in feather pulps of vaccinated chickens. Vaccine, 34, 1630–1633. doi: 10.1016/j.vaccine.2016.01.006
- Devlin, J.M., Browning, G.F., Hartley, C.A. & Gilkerson, J.R. (2007). Glycoprotein G deficient infectious laryngotracheitis virus is a candidate attenuated vaccine. Vaccine, 25, 3561–3566. doi: 10.1016/j.vaccine.2007.01.080
- Di Giovine, P., Settembre, E.C., Bhargava, A.K., Luftig, M.A., Lou, H. & Cohen, G.H. (2011). Structure of herpes simplex virus glycoprotein D bound to the human receptor nectin-1. PLoS Pathogens, 7, e1002277. doi: 10.1371/journal.ppat.1002277
- Ding, M.D., Wang, H.N., Cao, H.P., Fan, W.Q., Ma, B.C. & Xu, P.W. (2015). Development of a multi-epitope antigen of S protein-based ELISA for antibodies detection against infectious bronchitis virus. Bioscience, Biotechnology, and Biochemistry, 79, 1287–1295. doi: 10.1080/09168451.2015.1025692
- Dormitorio, T.V., Giambrone, J.J. & Macklin, K.S. (2013). Detection and isolation of infectious laryngotracheitis virus on a broiler farm after a disease outbreak. Avian Diseases, 57, 803–807. doi:10.1637/10544-032913-ResNote.1
- Dubois, M.E., Hammarlund, E. & Slifka, M.K. (2012). Optimization of peptide-based ELISA for serological diagnostics: a retrospective study of human monkeypox infection. Vector-Borne and Zoonotic Diseases, 12, 400–409. doi: 10.1089/vbz.2011.0779
- Dunaevskaia, G.N. (1988). Pains and the lumbosacral area. Fel'dsher I Akusherka, 53, 33–37.
- Eisenberg, R.J., Atanasiu, D., Cairns, T.M., Gallagher, J.R., Krummenacher, C. & Cohen, G.H. (2012). Herpes virus fusion and entry: a story with many characters. Viruses, 4, 800–832. doi: 10.3390/v4050800
- Fields, G.B. & Noble, R.L. (1990). Solid phase peptide synthesis utilizing 9-fluorenylmethoxycarbonyl amino acids. International Journal of Peptide and Protein Research, 35, 161–214. doi: 10.1111/j.1399-3011.1990.tb00939.x
- Fuchs, W., Veits, J., Helferich, D., Granzow, H., Teifke, J.P. & Mettenleiter, T.C. (2007). Molecular biology of avian infectious laryngotracheitis virus. Veterinary Research, 38, 261–279. doi: 10.1051/vetres:200657
- Garcia, M., Spatz, S. & Guy, J. (2013). Infectious laryngotracheitis. In D. Swayne (Ed.), Diseases of Poultry (Vol. 13, pp. 161–179). Ames, IA: John Wiley & Sons, Inc.
- Garcia, M. (2017). Current and future vaccines and vaccination strategies against infectious laryngotracheitis (ILT) respiratory disease of poultry. Veterinary Microbiology, 206, 157–162. doi: 10.1016/j.vetmic.2016.12.023
- Garcia, M., Spatz, S.J., Cheng, Y., Riblet, S.M., Volkening, J.D. & Schneiders, G.H. (2016). Attenuation and protection efficacy of ORF C gene-deleted recombinant of infectious laryngotracheitis virus. Journal of General Virology, 97, 2352–2362. doi: 10.1099/jgv.0.000521
- Gimeno, I.M., Cortes, A.L., Faiz, N.M., Hernandez-Ortiz, B.A., Guy, J.S. & Hunt, H.D. (2015). Evaluation of the protection efficacy of a serotype 1 Marek's disease virus-vectored bivalent vaccine against infectious laryngotracheitis and Marek's disease. Avian Diseases, 59, 255–262. doi: 10.1637/10966-103014-Reg
- Godoy, A., Icard, A., Martinez, M., Mashchenko, A., Garcia, M. & El-Attrachea, J. (2013). Detection of infectious laryngotracheitis virus antibodies by glycoprotein-specific ELISAs in chickens vaccinated with viral vector vaccines. Avian Diseases, 57, 432–436. doi: 10.1637/10345-090312-Reg.1
- Gowthaman, V., Koul, M. & Kumar, S. (2016). Avian infectious laryngotracheitis: a neglected poultry health threat in India. Vaccine, 34, 4276–4277. doi: 10.1016/j.vaccine.2016.07.002
- Guy, J.S. & Bagust, T.J. (2003). Laryngotracheitis. Diseases of Poultry, 11, 121–134.
- Hidalgo, H. (2003). Infectious laryngotracheitis: a review. Revista Brasileira de Ciência Avícola, 5, 157–168. doi: 10.1590/S1516-635X2003000300001
- Hopp, T.P. & Woods, K.R. (1981). Prediction of protein antigenic determinants from amino acid sequences. Proceedings of the National Academy of Sciences, 78, 3824–3828. doi: 10.1073/pnas.78.6.3824
- Hughes, C.S., Williams, R.A., Gaskell, R.M., Jordan, F.T., Bradbury, J.M. & Bennett, M. (1991). Latency and reactivation of infectious laryngotracheitis vaccine virus. Archives of Virology, 121, 213–218. doi: 10.1007/BF01316755
- Johnson, M.A., Prideaux, C.T., Kongsuwan, K., Sheppard, M. & Fahey, K.J. (1991). Gallid herpesvirus 1 (infectious laryngotracheitis virus): cloning and physical maps of the SA-2 strain. Archives of Virology, 119, 181–198. doi: 10.1007/BF01310669
- Kanabagatte Basavarajappa, M., Kumar, S., Khattar, S.K., Gebreluul, G.T., Paldurai, A. & Samal, S.K. (2014). A recombinant Newcastle disease virus (NDV) expressing infectious laryngotracheitis virus (ILTV) surface glycoprotein D protects against highly virulent ILTV and NDV challenges in chickens. Vaccine, 32, 3555–3563. doi: 10.1016/j.vaccine.2014.04.068
- Kanabagatte Basavarajappa, M., Song, H., Lamichhane, C. & Samal, S.K. (2015). Glycoprotein-based enzyme-linked immunosorbent assays for serodiagnosis of infectious laryngotracheitis. Journal of Clinical Microbiology, 53, 1727–1730. doi: 10.1128/JCM.02540-14
- Kirkpatrick, N.C., Mahmoudian, A., O'Rourke, D. & Noormohammadi, A.H. (2006). Differentiation of infectious laryngotracheitis virus isolates by restriction fragment length polymorphic analysis of polymerase chain reaction products amplified from multiple genes. Avian Diseases, 50, 28–34. doi: 10.1637/7414-072205R.1
- Kotiw, M., Wilks, C.R. & May, J.T. (1995). The effect of serial in vivo passage on the expression of virulence and DNA stability of an infectious laryngotracheitis virus strain of low virulence. Veterinary Microbiology, 45, 71–80. doi: 10.1016/0378-1135(94)00115-D
- Kumar, R., Barman, N.N., Khatoon, E. & Kumar, S. (2016). Development of single dilution immunoassay to detect E2 protein specific classical swine fever virus antibody. Veterinary Immunology and Immunopathology, 172, 50–54. doi: 10.1016/j.vetimm.2016.03.004
- Lazear, E., Whitbeck, J.C., Ponce-de-Leon, M., Cairns, T.M., Willis, S.H. & Zuo, Y. (2012). Antibody-induced conformational changes in herpes simplex virus glycoprotein gD reveal new targets for virus neutralization. Journal of Virology, 86, 1563–1576. doi: 10.1128/JVI.06480-11
- Leib, D.A., Bradbury, J.M., Hart, C.A. & McCarthy, K. (1987). Genome isomerism in two alphaherpesviruses: herpesvirus saimiri-1 (herpesvirus tamarinus) and avian infectious laryngotracheitis virus. Brief report. Archives of Virology, 93, 287–294. doi: 10.1007/BF01310982
- Lougovskaia, N.N., Lougovskoi, A.A., Bochkov, Y.A., Batchenko, G.V., Mudrak, N.S. & Drygin, V.V. (2002). Detection and estimation of avian infectious bronchitis virus antigen by a novel indirect liquid-phase blocking enzyme-linked immunosorbent assay using chicken and rabbit affinity purified immunoglobulins. Avian Pathology, 31, 549–557. doi: 10.1080/0307945021000024571
- Ma, F., Zhang, L., Wang, Y., Lu, R., Hu, B. & Lv, S. (2016). Development of a peptide ELISA for the diagnosis of Aleutian mink disease. PLoS One, 11(11), e0165793. doi:10.1371/journal.pone.0165793.
- Menendez, K.R., Garcia, M., Spatz, S. & Tablante, N.L. (2014). Molecular epidemiology of infectious laryngotracheitis: A review. Avian Pathology, 43, 108–117. doi:10.1080/03079457.2014.886004
- Neff, C., Sudler, C. & Hoop, R.K. (2008). Characterization of western European field isolates and vaccine strains of avian infectious laryngotracheitis virus by restriction fragment length polymorphism and sequence analysis. Avian Diseases, 52, 278–283. doi:10.1637/8168-110107-Reg.1
- Ojkic, D., Swinton, J., Vallieres, M., Martin, E., Shapiro, J. & Sanei, B. (2006). Characterization of field isolates of infectious laryngotracheitis virus from Ontario. Avian Pathology, 35, 286–292. doi: 10.1080/03079450600815481
- Parra, S., Nunez, L., Astolfi-Ferreira, C. & Ferreira, J. (2015). Occurrence of infectious laryngotracheitis virus (ILTV) in 2009-2013 in the State of São Paulo-Brazil. Revista Brasileira de Ciência Avícola, 17, 117–120.
- Pereira, L. (1994). Function of glycoprotein B homologues of the family herpesviridae. Infectious Agents and Disease, 3, 9–28.
- Ramadass, P., Parthiban, M., Thiagarajan, V., Chandrasekar, M., Vidhya, M. & Raj, G.D. (2008). Development of single serum dilution ELISA for detection of infectious bursal disease virus antibodies. Journal of Veterinary, 78, 23–30.
- Robinson, A., Bartlett, R.C. & Mazens, M.F. (1985). Antimicrobial synergy testing based on antibiotic levels, minimal bactericidal concentration, and serum bactericidal activity. American Journal of Clinical Pathology, 84, 328–333. doi: 10.1093/ajcp/84.3.328
- Roizman, B. (1982). The family herpesviridae: General description, taxonomy, and classification. In B. Roizman (Ed.), The Herpesviruses (pp. 1–23). Boston, MA: Springer US.
- Rouse, B.T. & Kaistha, S.D. (2006). A tale of 2 alpha-herpesviruses: lessons for vaccinologists. Clinical Infectious Diseases, 42, 810–817. doi: 10.1086/500141
- Snyder, D.B., Marquardt, W.W. & Russek, E. (1983). Rapid serological profiling by enzyme-linked immunosorbent assay, II. Comparison of computational methods for measuring antibody titer in a single serum dilution. Avian Diseases, 27, 474–484. doi: 10.2307/1590173
- Spear, P.G., Eisenberg, R.J. & Cohen, G.H. (2000). Three classes of cell surface receptors for alphaherpesvirus entry. Virology, 275, 1–8. doi:10.1006/viro.2000.0529
- Stanberry, L.R., Spruance, S.L., Cunningham, A.L., Bernstein, D.I., Mindel, A. & Sacks, S. (2002). Glycoprotein-D-adjuvant vaccine to prevent genital herpes. New England Journal of Medicine, 347, 1652–1661. doi: 10.1056/NEJMoa011915
- Sun, P., Ju, H., Liu, Z., Ning, Q., Zhang, J. & Zhao, X. (2013). Bioinformatics resources and tools for conformational B-cell epitope prediction. Computational and Mathematical Methods in Medicine, 2013, 943636.
- Thureen, D.R. & Keeler, C.L. (2006). Psittacid herpesvirus 1 and infectious laryngotracheitis virus: Comparative genome sequence analysis of two avian alphaherpesviruses. Journal of Virology, 80, 7863–7872. doi: 10.1128/JVI.00134-06
- Velumani, S., Ho, H.T., He, F., Musthaq, S., Prabakaran, M. & Kwang, J. (2011). A novel peptide ELISA for universal detection of antibodies to human H5N1 influenza viruses. PLoS One, 6, e20737. doi: 10.1371/journal.pone.0020737
- Vita, R., Zarebski, L., Greenbaum, J.A., Emami, H., Hoof, I. & Salimi, N. (2010). The immune epitope database 2.0. Nucleic Acids Research, 38, D854–D862. doi: 10.1093/nar/gkp1004
- Watrach, A.M., Hanson, L.E. & Watrach, M.A. (1963). The structure of infectious laryngotracheitis virus. Virology, 21, 601–608. doi: 10.1016/0042-6822(63)90233-2
- Yan, Z., Li, S., Xie, Q., Chen, F. & Bi, Y. (2016). Characterization of field strains of infectious laryngotracheitis virus in China by restriction fragment length polymorphism and sequence analysis. Journal of Veterinary Diagnostic Investigation, 28, 46–49. doi:10.1177/1040638715618230
- York, J.J. & Fahey, K.J. (1988). Diagnosis of infectious laryngotracheitis using a monoclonal antibody ELISA. Avian Pathology, 17, 173–182. doi: 10.1080/03079458808436436
- York, J.J., Fahey, K.J. & Bagust, T.J. (1983). Development and evaluation of an ELISA for the detection of antibody to infectious laryngotracheitis virus in chickens. Avian Diseases, 27, 409–421. doi: 10.2307/1590167
- York, J.J., Sonza, S., Brandon, M.R. & Fahey, K.J. (1990). Antigens of infectious laryngotracheitis herpesvirus defined by monoclonal antibodies. Archives of Virology, 115, 147–162. doi: 10.1007/BF01310527
- York, J.J., Sonza, S. & Fahey, K.J. (1987). Immunogenic glycoproteins of infectious laryngotracheitis herpesvirus. Virology, 161, 340–347. doi: 10.1016/0042-6822(87)90126-7
- Zhao, W., Spatz, S., Zhang, Z., Wen, G., Garcia, M. & Zsak, L. (2014). Newcastle disease virus (NDV) recombinants expressing infectious laryngotracheitis virus (ILTV) glycoproteins gB and gD protect chickens against ILTV and NDV challenges. Journal of Virology, 88, 8397–8406. doi: 10.1128/JVI.01321-14