ABSTRACT
Since the first report of chicken infectious anaemia virus (CIAV) in Vietnam in 2013, there have not been many studies focused on the detection of CIAV or the molecular characteristics of the virus. This study attempted to investigate the presence of CIAV in northern Vietnam by molecular-based methods. Regarding the spatial distribution of CIAV, the PCR-based results showed that CIAV was detected in 47 out of 64 farms (73.4%) and in all 10 investigated provinces. Of the 119 samples assayed by PCR, 74 (62.2%) tested positive for CIAV DNA. By arranging the samples into different categories, it was found that CIAV was detected at high rates (above 50%) based on all 4 evaluated criteria as follows: production type of chicken, housing system, flock size and age group. Different housing systems were significantly associated with the detection rates of CIAV (P = 0.003). By genetic analyses, all of the Vietnamese CIAVs were found to (i) lack substitutions related to attenuation substitutions, (ii) group separately from vaccine-like CIAVs and (iii) belong to genogroups G2 and G3 of CIAV. Because of the wide distribution of CIAV and because the virus was confirmed not to be vaccine-like viruses, it is suggested that further studies be conducted on the clinical form of chicken infectious anaemia, as well as the immunosuppressive effect of CIAV on chickens in Vietnam.
RESEARCH HIGHLIGHTS
Wide distribution of chicken infectious anaemia virus (CIAV) in northern Vietnam.
Vietnamese CIAVs belong to genogroups G2 and G3 of CIAV.
Introduction
Chicken infectious anaemia virus (CIAV), the only member of the genus Gyrovirus in the family Anelloviridae (Rosario et al., Citation2017), is a causative agent of chicken infectious anaemia disease (CIA) (Yuasa et al., Citation1979). The CIAV genome is a unisense, single-stranded, closed circular DNA of approximately 2,300 nucleotides in length (Noteborn et al., Citation1991; Meehan et al., Citation1992) and possesses three overlapping open reading frames (Noteborn et al., Citation1991). Of the three genes, VP1 (the most variable gene) encodes the capsid protein, VP2 encodes a protein that possesses dual-specificity protein phosphatase activity (Peters et al., Citation2002), and VP3 encodes a protein with apoptotic activity (Noteborn et al., Citation1994). The genes associated with the pathogenesis of CIAV are VP1 (Renshaw et al., Citation1996; Scott et al., Citation2001; Yamaguchi et al., Citation2001; Todd et al., Citation2002) and VP2 (Peters et al., Citation2006; Peters et al., Citation2007). Another indication of a genetic signature of attenuation was a 21-nucleotide insertion within the nontranscribed region of the CIAV genome (Meehan et al., Citation1997).
CIAV can infect chickens of all ages (Kaffashi et al., Citation2006) and is highly prevalent in chicken flocks (De Herdt et al., Citation2001). However, a clinical form of the disease usually occurs in chickens less than 3 weeks old (McNulty, Citation1991) and is characterized by anaemia and atrophy of the lymphoid organs (Yuasa et al., Citation1979; Goodwin et al., Citation1989; Buscaglia et al., Citation1994). In contrast, for a particular CIAV isolate (Toro et al., Citation1997) or in combination with immunosuppressive viruses (McNulty, Citation1991; Hu et al., Citation1993), the age resistance property of CIA could be altered.
CIAV is believed to be ubiquitous in all chicken producing countries. That was evidenced many years ago by a serological survey published in 1989 which found antibodies against CIAV in specific-pathogen-free chicken flocks in Europe, America, and Australia (McNulty et al., Citation1989). It was also supported by the fact that CIAV was isolated and continually detected in many countries in Europe (Todd et al., Citation1992; Olszewska-Tomczyk et al., Citation2016), America (van Santen et al., Citation2001; Simionatto et al., Citation2006), Africa (Oluwayelu et al., Citation2005; Snoeck et al., Citation2012), and Asia (Yuasa et al., Citation1979; Zhou et al., Citation1997; Natesan et al., Citation2006; Ducatez et al., Citation2008). For countries in Southeast Asia, CIAV and its related disease were confirmed in Malaysia (Chowdhury et al., Citation2002), Cambodia (Kye et al., Citation2013), and Thailand (Wanasawaeng et al., Citation2013). In Vietnam, the first published study describing CIAV reported that antibodies against CIAV were present in chicken sera sampled in 2013 (Trinh et al., Citation2015). The second report (Dao et al., Citation2018) described the prevalence of CIAV mainly in Hanoi. Because CIAV has not been thoroughly investigated in Vietnam, several questions remain to be answered, such as whether the clinical disease is present in the field, to what extent the disease exerts immunosuppressive effects, and what the genetic characteristics of CIAV are on a large scale. To answer these questions, this study was the first to investigate the presence of CIAV in northern Vietnam by PCR-based methods. Based on these results, phylogenetic and genetic analyses were performed to determine the genetic characteristics of the virus circulating in northern Vietnam.
Materials and methods
Sample collection
In Vietnam, chickens are reared in all six ecological regions from North to South ((A, B)). Estimated for 2018, the total chicken population was approximately 316 million birds. The chicken population in the North accounted for approximately 48.4% of the whole country (statistical data for the year 2018 on Agriculture, Forestry and Fishing, General Statistics Office of Vietnam, https://www.gso.gov.vn/default_en.aspx?tabid=778, last accessed on April 20, 2019). To enhance the likelihood of detecting CIAV, this study focused on 10 northern provinces with high chicken densities ((B,C)). The chosen farms (n = 64: 11 in 2016, 36 in 2017 and 17 in 2018) were selected according to convenience and willingness to participate. No farms used the CIAV vaccine.
Figure 1. The distribution of CIAV according to geographical locations. (A) Map of six ecological regions from North to South of Vietnam. (B) Chart showing the percentage of chickens in each ecological region. (C) Spatial distribution of CIAV at sampling sites: 10 northern provinces are highlighted. The stacked column indicates the proportion of CIAV-positive (red) and negative (blue) tests to the total samples collected in each province. The distribution of the CIAV genogroup in each province is indicated by ★ (genogroup G2 and G3) and ● (genogroup G3).
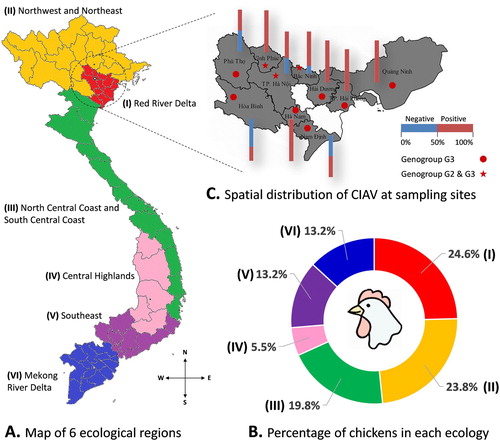
All samples analyzed were from commercial layer farms (n = 76) and broiler farms (n = 43). The vaccination programme of the parent flocks against CIAV was not known. Fifteen collected chicken breeds were grouped into egg-type (four exotic breeds) and meat-type (11 native breeds that grow to slaughter weight in a minimum of 12 weeks). For sample collection, as this study focused on pathogen detection, sick birds (depression, ruffled feathers, etc.) of three age groups (1–4, 5–15, and ≥ 16 weeks) were selected. Because chickens only display age resistance to the disease (McNulty, Citation1991) but not to the infection, the arrangement of samples in the three abovementioned age groups reflects the production cycles. At necropsy, lymphoid and internal organs from each bird were pooled. These organs were the Harderian gland, thymus, bursa of Fabricius, caecal tonsil, bone marrow, heart, liver, spleen and kidney.
Nucleic acid extraction
Total DNA was extracted from 10% tissue homogenate using an automated magnetic bead-based extraction system (taco™ Nucleic Acid Automated Extraction System). The commercial kit used was the taco™ DNA/RNA Extraction Kit (atc-d/rna, GeneReach Biotechnology Corp., Taiwan). All steps were done per the manufacturer’s instructions.
PCR-based detection and sequencing
Extracted nucleic acid was screened by PCR for CIAV genomic DNA as described previously (van Santen et al., Citation2001; Amer et al., Citation2011). Subsequently, all CIAV-positive samples were whole-genome sequenced by the Sanger method (1st BASE, Malaysia) using three pairs of primers as described elsewhere (Li et al., Citation2016). As a result, three complete genomes and 55 partial VP1 genes covering the hypervariant region were obtained by assembling overlapping fragments using BioEdit 7.0.5.3 (Hall, Citation1999). All 58 CIAV strains sequenced in this study were deposited in GenBank under the accession numbers MH536104- MH536106 and MF611928- MF611982.
Phylogenetic analysis and genetic characterization
According to previous publications (Ducatez et al., Citation2008; Kye et al., Citation2013; Ou et al., Citation2018), the phylogenetic relationships between CIAV strains were reconstructed by the neighbor-joining method implemented in MEGA6 (Tamura et al., Citation2013), using the Kimura-2 parameter as a model of nucleotide substitutions and 1,000 bootstrap replications. Reference CIAV strains of genogroups G1–G3 (Olszewska-Tomczyk et al., Citation2016) and a recently proposed genogroup G4 (Ou et al., Citation2018), were included for phylogenetic classification. The complete list of sequences used in this study can be found in supplementary Table S1.
Variations of VP1 gene were assessed via 12 amino acid sites which (i) played roles in replication and pathogenicity the virus (Renshaw et al., Citation1996; Scott et al., Citation2001; Yamaguchi et al., Citation2001; Todd et al., Citation2002) and (ii) were under positive selection (Wang et al., Citation2009). Patterns of amino acid substitutions were visualized by WebLogo programme (Crooks et al., Citation2004). The overall height of each stack (measured in bits) indicated the sequence conservation at that position. In each position, only the most frequent residues were shown. The height of symbols within the stack indicated the relative frequency of each amino acid at that position.
Statistical analysis
Data were entered into Microsoft Excel 2010 for basic calculation. To assess whether the detection rate of CIAV differed across production type of chicken, housing system, flock size or age group, analysis of variance (one-way ANOVA) was applied. In cases where the assumption of homogeneity of variance was violated, the Kruskal–Wallis test (one-way ANOVA on ranks) was used instead. All data analyses were performed using IBM SPSS for Windows, version 22 (IBM Corp., 2013, Armonk, USA). A P-value of 0.05 or less was considered to be significant.
Results
Detection of CIAV in northern provinces
This study took samples from 10 provinces, seven of which belonged to the Red River Delta, where chickens were raised at a high density and accounted for about 24.6% of the total number of chickens in Vietnam ((B)). As shown in (C), CIAV was detected in all 10 investigated provinces. There were 47 out of 64 (73.4%) commercial chicken farms that were CIAV-positive. Of the 119 samples assayed by PCR, 74 (62.2%) tested positive for CIAV DNA. The CIAV-positive samples were arranged and analyzed under different criteria as follows. Of the production types ((A)), CIAV was detected in both meat- and egg-types. The positive rates were higher in the meat-type (69.8%) than in the egg-type (57.9%). However, the difference was not significant (P = 0.201). It was revealed that the housing system ((B)) clearly affected the occurrence of CIAV. A higher magnitude of CIAV-positive rates was recorded in the free-range system (80.5%) than in the intensive production system (52.6%) (P = 0.003).
Figure 2. The detection rates of CIAV according to the type of chicken (A), housing system (B), flock size (C) and age group (D). CIAV positive rates (in percentage, black dots) and 95% confidence intervals (bars). A P-value at the top-left corner of each panel, which was 0.05 or less, indicated a statistically significant difference between group means.
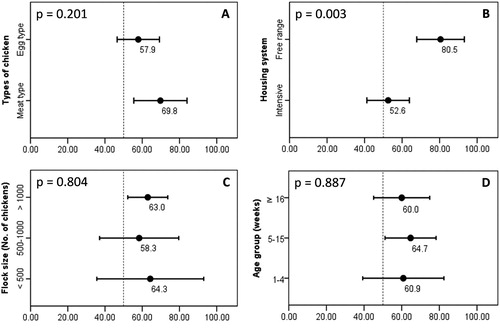
The epidemiological features of CIAV infections were further elucidated by analyzing positive samples according to the flock size and age group of chickens. At the flock level ((C)), the positive rates for small (< 500 birds), medium (500–1000 birds) and large (> 1000 birds) flocks were 64.3%, 58.3%, and 63.0%, respectively. Because of overlapping 95% confidence intervals, the CIAV-positive rates among flock scales were not significantly different (P = 0.804). Considering the ages of chickens, (D) clearly shows that chickens of all ages were CIAV-positive. The positive rates for chickens in the periods of 1–4 weeks, 5–15 weeks and ≥ 16 weeks were 60.9%, 64.7%, and 60.0%, respectively. By one-way ANOVA, the null hypothesis of an equal mean positive rate among the three age groups was not rejected (P = 0.887), implying that CIAV circulating in northern Vietnam did not have a significant trend toward any age group.
Phylogenetic classification of Vietnamese CIAVs
The phylogenetic trees of CIAV reconstructed from complete genomes (n = 162), complete VP1 genes (n = 395) and partial VP1 genes (n = 450) are depicted in . The details of the phylogenetic trees are given in supplementary Figures S1–S3. In all cases, CIAV was consistently divided into three genogroups, G1 to G3, with high bootstrap support values (100%). All Vietnamese CIAVs (including sequences of three strains generated in this study, filled arrows, (A-C)) fell only within genogroups G2 and G3. Based on the phylogenetic tree inferred from complete genomes, one field strain of genogroup G3 collected in this study (G17.33.3, MH536104) was within a cluster containing vaccine strains (Nobilis P4, Del-Ros) as well as high passage strains (Cux-1 passages 310, SMSC-1 passages 60, 123, etc.). However, when the number of sequences increased (n = 395 for complete VP1 gene, n = 450 for partial VP1 gene), the relationships between strains were more precisely defined. As a result, none of Vietnamese strains (collected in 2013, 2016 and 2017) were within the cluster containing CIAV vaccine strains ((B,C)).
Figure 3. Phylogenetic trees of CIAV inference based on the complete genome (A), complete VP1 gene (B) and partial VP1 gene (C). The viruses were clearly divided into three genogroups from G1 to G3. The trees were outgroup-rooted by genogroup G1. Vietnamese CIAVs are coloured in violet and are indicated by arrows according to year of isolation: 2013 (empty arrow), 2016 (arrow head), 2017 (filled arrow). Asterisks (*) indicate long branches of four strains with extreme amino acid substitutions at sites 194–225 (EF552227), 249–275 (EF552229, EF552230), and 416–449 (HM134240) of VP1. The branch of vaccine and high passage CIAV strains is indicated by a vertical double-headed arrow. The scale under each tree measures changes in the unit of the number of base substitutions per site.
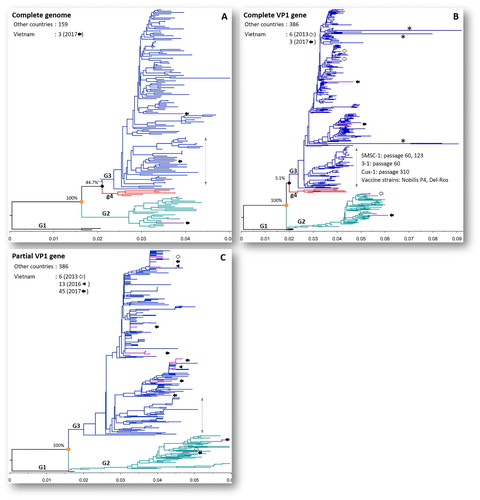
Genomic characterization of Vietnamese CIAVs
At the time of the study, only coding sequences of Vietnamese CIAVs were available from GenBank. As a result, the comparative genomics were done between three Vietnamese CIAVs (this study) and the viruses of three genogroups. At the genomic level, all three field strains consistently showed the most variable regions were within the VP1 gene. This gene contained two regions (asterisks, (A-C)) having genetic similarity of less than 95% (dashed lines) in comparison with strains from all three genogroups. An additional area of low genetic similarity (star, (A)) was observed in the noncoding region when three Vietnamese strains were compared with genogroup G1.
Figure 4. Whole genome comparative analysis of genetic similarity between 3 Vietnamese field strains and three genogroups of CIAV. The comparisons between genogroup 1 to genogroup 3 are shown from A to C. Each point plotted is the percentage genetic similarity within a 200-nt-wide sliding window centered on the position plotted with a step size of 20 nt and Kimura 2-parameter. Regions with genetic similarity less than 95% (dashed line) are indicated by star and asterisk symbols. The lower region in each panel shows the gene structure of CIAV corresponding to nucleotide positions in the plot map.
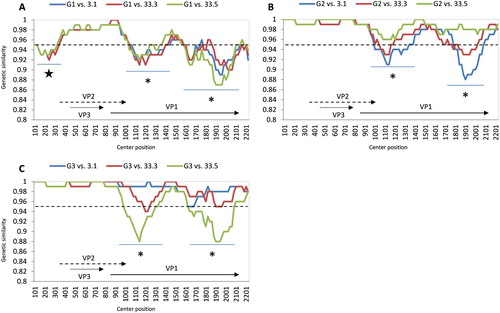
At gene level, variations of the VP1 gene were assessed via 12 amino acid sites, which played roles in replication and pathogenicity of the virus, and were under positive selection (see discussion below). It is apparent in (A, C) that the amino acid motif is different between genogroups G2 and G3. This was also reflected between genogroups G2 and G3 of Vietnamese CIAV strains ((B, D)). Within genogroup G3, the sequence logos revealed five positions (arrows, (C, E)) in which certain residues favoured specific positions in field CIAV strains and in the vaccine and high passage strains (shown in (A-C)). Accordingly, it was observed that all of the Vietnamese genogroup G3 viruses resembled the sequence pattern of field CIAV strains ((D)). Several amino acid substitutions, experimentally demonstrated to be associated with lower pathogenicity, were not observed in the Vietnamese strains, such as 394H or a complete pattern of 75I- 89T- 125L- 141L- 144E (supplementary Table S2). An indication of a genetic signature of attenuation (21-nucleotide insertion within the nontranscribed region of the CIAV genome) was not observed in any of three completed genomes of Vietnamese CIAVs (data not shown).
Figure 5. Sequence logo plot of important amino acid positions of the VP1 protein. The plots were created using WebLogo3 for genogroup 2 (n = 63, A) and genogroup 3 (n = 319, C, E). For comparison, amino acid sequence logos were also constructed for Vietnamese CIAVs of genogroup 2 (n = 3, B) and genogroup 3 (n = 6, D). For genogroup 3, the logo plot was separately generated for the sequences belonging (E) and not belonging (C, D) to the branch of vaccine and high passage CIAV strains (n = 74 and n = 245, respectively). Arrows indicate amino acid sites exhibiting different variations between these branches. The overall height of each stack (measured in bits) indicates the sequence conservation at that position. The height of symbols within the stack indicates the relative frequency of each amino acid at that position. Amino acids are coloured according to their hydrophobicity: blue, green and black for hydrophilic, neutral and hydrophobic, respectively. The numbers on the horizontal axis are the amino acid positions of the VP1 protein.
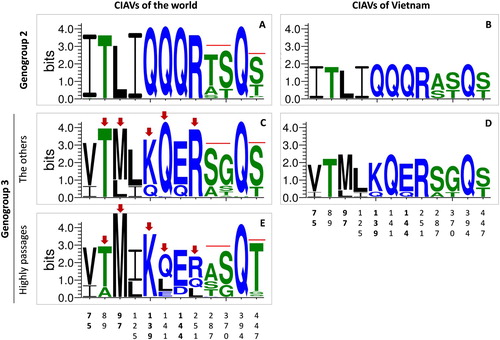
Discussion
Although CIAV appears to be ubiquitous in all major chicken-producing countries (Dhama et al., Citation2008), to date, there have been few studies investigating the virus and the disease in Vietnam. In this study, the presence of CIAV in northern Vietnam was investigated in different ways. First, regarding the spatial distribution of the virus, it was revealed that CIAV was present in all 10 sampled provinces ((C)) and in 47 out of 64 examined farms (73.4%). In Vietnam, poultry are raised in every ecological region ((B)). The poultry production system contains millions of small- to large-scale households. From that perspective, 64 investigated farms in this study represented a small sample. Fortunately, the sampling sites were in areas with high density (about 3000 chickens per km2) that accounted for about 24.6% of the total number of chickens in Vietnam ((B)). This sampling was representative of the source population with regard to pathogen detection in general, and CIAV in particular. Combined with two previous studies that detected CIAV in Hanoi and Hanam Provinces (Trinh et al., Citation2015; Dao et al., Citation2018), it could be inferred that CIAV is distributed widely in almost all the main provinces where a high density of chicken farms are located. This wide distribution is further supported by the finding that CIAV was detected at high rates of above 50% based on all four evaluated criteria: production type of chicken ((A)), housing system ((B)), flock size ((C)) and age group ((D)). The high detection rate of CIAV in the northern provinces of Vietnam is comparable with the rates reported in several other countries. With the PCR method, 58.4% flocks in Iran were positive for CIAV (Gholami-Ahangaran et al., Citation2013). In Turkey, CIAV was detected in 80.0% of flocks (Hadimli et al., Citation2008).
By arranging the samples into different categories (), it was found that only the housing system had significant relevance to the detection rates of CIAV. CIAV is known for its outstanding stability against disinfectants, especially in the form associated with tissue material or faeces (Yuasa, Citation1992). Thus, elimination of the virus from poultry facilities is not straightforward. This property, combined with the fact that most CIAV infections are subclinical (McNulty, Citation1991), helps to explain why the virus was detected at high rates in both indoor and outdoor housing systems ((B)). However, chickens kept in outdoor housing are clearly more frequently CIAV-positive than those kept in indoor housing (P = 0.003). This finding might be observed due to a higher probability of exposure to potential sources of disease. Consequently, chickens in free-range systems are more vulnerable to infections, of which avian influenza virus is a well-known example (Miao et al., Citation2005; Biswas et al., Citation2009).
Other epidemiological features of CIAV infections in northern Vietnam were: (i) the occurrence in all growth stages of the chicken and (ii) the lack of a significant trend toward an individual age group ((D)). It is known that the active or passive immune response against CIAV induced by vaccination or maternal antibodies is not sterilizing immunity and that the virus can establish a persistent infection for months in chickens (Hoop, Citation1992; Cardona et al., Citation2000; Brentano et al., Citation2005). Additionally, chickens are known to display age resistance to the disease (McNulty, Citation1991) but not to the infection, which was supported by the fact that CIAV replicated at similar levels in 1-day-old and 6-week-old chickens (Kaffashi et al., Citation2006). Combined with the high degree of resistance of CIAV to chemical inactivation (Yuasa, Citation1992) and wide distribution at the farm level ((A-C)), it is understandable why the virus was detected at an average rate of 62.2%, irrespective of age group. This finding was different from a study that showed a decreasing prevalence of CIAV with increasing age of birds (Wani et al., Citation2013). That finding was based on testing samples from chicken flocks suspected of CIA with a history of anaemia. A possible explanation for the discrepancy between the findings of the two studies is that this study investigated the presence of CIAV across age groups, rather than in individual ages; thus, the density of age-associated prevalence might be diluted.
When a large number of sequences was included in the phylogenetic analysis, the relationships between strains were more precisely defined. As a result, this study approached the classification of CIAV strains by analyzing a large number of genome sequences (n = 162) and VP1 sequences (n = 395 or 450 for complete and partial genes, respectively). The tree topologies ((A,B)) revealed a newly proposed genogroup of CIAV, G4 (Ou et al., Citation2018). However, that split was supported by low bootstrap values (less than 50% in both genome- and VP1 gene-based phylogenies). As illustrated in (A-C), all of the Vietnamese CIAVs collected in 2013, 2016 and 2017 were grouped within genogroups G2 and G3. Supported by the branching pattern ((B-C)) and the amino acid motif of the VP1 protein ((D), supplementary Table S2), none of the Vietnamese CIAV strains clustered within the branch of high passage and vaccine strains. This result implied that all CIAVs found in this study were field strains. That differed from the situation in Taiwan (Ou et al., Citation2018) and Poland (Olszewska-Tomczyk et al., Citation2016) where vaccine-like isolates were detected. This result can be explained by the fact that live CIAV vaccination is not a common practice in Vietnam; for example, upon interview, all 64 farm owners investigated in this study said that they did not hear about the CIAV vaccine elsewhere. Due to the wide distribution of CIAVs ((C)), it is suggested that further studies should be conducted to investigate the clinical form of chicken infectious anaemia. In this study, variations of the VP1 gene were observed at 8 out of 12 amino acid sites ((B-D)), which (i) played roles in replication and pathogenicity of the virus (Renshaw et al., Citation1996; Scott et al., Citation2001; Yamaguchi et al., Citation2001; Todd et al., Citation2002), and (ii) were under positive selection (Wang et al., Citation2009). Additionally, as CIAV field isolates might differ in their in vivo pathogenicity (Rimondi et al., Citation2014), further experiments should be conducted to test the pathogenicity of Vietnamese CIAV strains and to investigate to what extent the infection exerts immunosuppressive effects.
Supplemental Material
Download Zip (10.6 MB)Acknowledgements
This study was supported by the Vietnam-Belgium Project, AI Program with Vietnam National University of Agriculture 2014–2019 (grant number 04/DAVB). We are grateful to all farmers who granted access to their farms and their kind cooperation.
Disclosure statement
No potential conflict of interest was reported by the authors.
ORCID
Le Thi My Huynh http://orcid.org/0000-0002-4257-3507
Additional information
Funding
References
- Amer, H.M., Elzahed, H.M., Elabiare, E.A., Badawy, A.A. & Yousef, A.A. (2011). An optimized polymerase chain reaction assay to identify avian virus vaccine contamination with Chicken anemia virus. Journal of Veterinary Diagnostic Investigation, 23, 34–40. doi: 10.1177/104063871102300105
- Biswas, P.K., Christensen, J.P., Ahmed, S.S., Das, A., Rahman, M.H., Barua, H., Giasuddin, M., Hannan, A.S., Habib, M.A. & Debnath, N.C. (2009). Risk for infection with highly pathogenic avian influenza virus (H5N1) in backyard chickens, Bangladesh. Emerging Infectious Diseases, 15, 1931–1936. doi: 10.3201/eid1512.090643
- Brentano, L., Lazzarin, S., Bassi, S.S., Klein, T.A. & Schat, K.A. (2005). Detection of chicken anemia virus in the gonads and in the progeny of broiler breeder hens with high neutralizing antibody titers. Veterinary Microbiology, 105, 65–73. doi: 10.1016/j.vetmic.2004.09.019
- Buscaglia, C., Crosetti, C.F. & Nervi, P. (1994). Identification of chicken infectious anaemia, isolation of the virus and reproduction of the disease in Argentina. Avian Pathology, 23, 297–304. doi: 10.1080/03079459408418997
- Cardona, C.J., Oswald, W.B. & Schat, K.A. (2000). Distribution of chicken anaemia virus in the reproductive tissues of specific-pathogen-free chickens. Journal of General Virology, 81, 2067–2075. doi: 10.1099/0022-1317-81-8-2067
- Chowdhury, S.M., Omar, A.R., Aini, I., Hair-Bejo, M., Jamaluddin, A.A., Kono, Y., Darus, A., & Yatim H.M. (2002). Isolation, identification and characterization of chicken anaemia virus in Malaysia. The Journal of Biochemistry, Molecular Biology and Biophysics, 6, 249–255. doi: 10.1080/10258140290022310
- Crooks, G.E., Hon, G., Chandonia, J.M. & Brenner, S.E. (2004). Weblogo: a sequence logo generator. Genome Research, 14, 1188–1190. doi: 10.1101/gr.849004
- Dao, D., Cao, T., Vu, T., Nguyen, V. & Huynh, T. (2018). Prevalence of chicken infectious anemia virus (CIAV) circulating in Hanoi and surrounding provinces. Vietnam Journal of Agricultural Sciences, 16, 36–45.
- De Herdt, P., Van den Bosch, G., Ducatelle, R., Uyttebroek, E. & Schrier, C. (2001). Epidemiology and significance of chicken infectious anemia virus infections in broilers and broiler parents under nonvaccinated European circumstances. Avian Diseases, 45, 706–708. doi: 10.2307/1592916
- Dhama, K., Mahendran, M., Somvanshi, R. & Chawak, M.M. (2008). Chicken infectious anaemia virus: An immunosuppressive pathogen of poultry-A review. Indian Journal of Veterinary Pathology, 32, 158–167.
- Ducatez, M.F., Chen, H., Guan, Y. & Muller, C.P. (2008). Molecular epidemiology of chicken anemia virus (CAV) in southeastern Chinese live birds markets. Avian Diseases, 52, 68–73. doi: 10.1637/8049-070407-Reg
- Gholami-Ahangaran, M., Fathi-Hafshejani, E. & Seyed-Hosseini, R. (2013). Seromolecular study of chicken infectious anemia in chickens, ostriches, and turkeys in Iran. The Journal of Applied Poultry Research, 22, 404–409. doi: 10.3382/japr.2012-00567
- Goodwin, M.A., Brown, J., Miller, S.L., Smeltzer, M.A., Steffens, W.L. & Waltman, W.D. (1989). Infectious anemia caused by a parvovirus-like virus in Georgia broilers. Avian Diseases, 33, 438–445. doi: 10.2307/1591102
- Hadimli, H.H., Erganis, O., Guler, L. & Ucan, U.S. (2008). Investigation of chicken infectious anemia virus infection by PCR and ELISA in chicken flocks. Turkish Journal of Veterinary and Animal Sciences, 32, 79–84.
- Hall, T.A. (1999). Bioedit: A user-friendly biological sequence alignment editor and analysis program for Windows 95/98/NT. Nucleic Acids Symposium Series, 41, 95–98.
- Hoop, R.K. (1992). Persistence and vertical transmission of chicken anaemia agent in experimentally infected laying hens. Avian Pathology, 21, 493–501. doi: 10.1080/03079459208418867
- Hu, L.B., Lucio, B. & Schat, K.A. (1993). Abrogation of age-related resistance to chicken infectious anemia by embryonal bursectomy. Avian Diseases, 37, 157–169. doi: 10.2307/1591469
- Kaffashi, A., Noormohammadi, A.H., Allott, M.L. & Browning, G.F. (2006). Viral load in 1-day-old and 6-week-old chickens infected with chicken anaemia virus by the intraocular route. Avian Pathology, 35, 471–474. doi: 10.1080/03079450601028837
- Kye, S.J., Kim, J.Y., Seul, H.J., Kim, S., Kim, S.E., Lee, H.S., Sorn, S. & Choi, K.S. (2013). Phylogenetic analysis and genetic characterization of chicken anemia virus isolates from Cambodia. Poultry Science, 92, 2681–2686. doi: 10.3382/ps.2013-03204
- Li, Y., Wang, Y., Fang, L., Fu, J., Cui, S., Zhao, Y., Cui, Z., Chang, S. & Zhao, P. (2016). Genomic analysis of the chicken infectious anemia virus in a specific pathogen-free chicken population in China. Biomed Research International, 2016, 1–5.
- McNulty, M.S. (1991). Chicken anaemia agent: a review. Avian Pathology, 20, 187–203. doi: 10.1080/03079459108418756
- McNulty, M.S., Connor, T.J. & McNeilly, F. (1989). A survey of specific pathogen-free chicken flocks for antibodies to chicken anaemia agent, avian nephritis virus and group a rotavirus. Avian Pathology, 18, 215–220. doi: 10.1080/03079458908418596
- Meehan, B.M., Todd, D., Creelan, J.L., Connor, T.J. & McNulty, M.S. (1997). Investigation of the attenuation exhibited by a molecularly cloned chicken anemia virus isolate by utilizing a chimeric virus approach. Journal of Virology, 71, 8362–8367.
- Meehan, B.M., Todd, D., Creelan, J.L., Earle, J.A., Hoey, E.M. & McNulty, M.S. (1992). Characterization of viral DNAs from cells infected with chicken anaemia agent: sequence analysis of the cloned replicative form and transfection capabilities of cloned genome fragments. Archives of Virology, 124, 301–319. doi: 10.1007/BF01309811
- Miao, Z.H., Glatz, P.C. & Ru, Y.J. (2005). Free-range poultry production - a review. Asian-Australasian Journal of Animal Sciences, 18, 113–132. doi: 10.5713/ajas.2005.113
- Natesan, S., Kataria, J.M., Dhama, K., Rahul, S. & Bhardwaj, N. (2006). Biological and molecular characterization of chicken anaemia virus isolates of Indian origin. Virus Research, 118, 78–86. doi: 10.1016/j.virusres.2005.11.017
- Noteborn, M.H., de Boer, G.F., van Roozelaar, D.J., Karreman, C., Kranenburg, O., Vos, J.G., Jeurissen, S.H., Hoeben, R.C., Zantema, A. & Koch, G. (1991). Characterization of cloned chicken anemia virus DNA that contains all elements for the infectious replication cycle. Journal of Virology, 65, 3131–3139.
- Noteborn, M.H., Todd, D., Verschueren, C.A., de Gauw, H.W., Curran, W.L., Veldkamp, S., Douglas, A.J., McNulty, M.S. & Koch, G. (1994). A single chicken anemia virus protein induces apoptosis. Journal of Virology, 68, 346–351.
- Olszewska-Tomczyk, M., Swieton, E., Minta, Z. & Smietanka, K. (2016). Occurrence and phylogenetic studies of chicken anemia virus from Polish broiler flocks. Avian Diseases, 60, 70–74. doi: 10.1637/11277-091415-ResNote.1
- Oluwayelu, D.O., Todd, D., Ball, N.W., Scott, A.N., Oladele, O.A., Emikpe, B.O., Fagbohun, O.A., Owoade, A.A. & Olaleye, O.D. (2005). Isolation and preliminary characterization of chicken anemia virus from chickens in Nigeria. Avian Diseases, 49, 446–450. doi: 10.1637/7339-020705R.1
- Ou, S.C., Lin, H.L., Liu, P.C., Huang, H.J., Lee, M.S., Lien, Y.Y. & Tsai, Y.L. (2018). Epidemiology and molecular characterization of chicken anaemia virus from commercial and native chickens in Taiwan. Transboundary and Emerging Diseases, 0, 1–9.
- Peters, M.A., Crabb, B.S., Tivendale, K.A. & Browning, G.F. (2007). Attenuation of chicken anemia virus by site-directed mutagenesis of VP2. Journal of General Virology, 88, 2168–2175. doi: 10.1099/vir.0.82904-0
- Peters, M.A., Crabb, B.S., Washington, E.A. & Browning, G.F. (2006). Site-directed mutagenesis of the VP2 gene of chicken anemia virus affects virus replication, cytopathology and host-cell MHC class I expression. Journal of General Virology, 87, 823–831. doi: 10.1099/vir.0.81468-0
- Peters, M.A., Jackson, D.C., Crabb, B.S. & Browning, G.F. (2002). Chicken anemia virus VP2 is a novel dual specificity protein phosphatase. Journal of Biological Chemistry, 277, 39566–39573. doi: 10.1074/jbc.M201752200
- Renshaw, R.W., Soine, C., Weinkle, T., O'Connell, P.H., Ohashi, K., Watson, S., Lucio, B., Harrington, S. & Schat, K.A. (1996). A hypervariable region in VP1 of chicken infectious anemia virus mediates rate of spread and cell tropism in tissue culture. Journal of Virology, 70, 8872–8878.
- Rimondi, A., Pinto, S., Olivera, V., Dibarbora, M., Perez-Filgueira, M., Craig, M.I. & Pereda, A. (2014). Comparative histopathological and immunological study of two field strains of chicken anemia virus. Veterinary Research, 45, 102. doi: 10.1186/s13567-014-0102-y
- Rosario, K., Breitbart, M., Harrach, B., Segales, J., Delwart, E., Biagini, P. & Varsani, A. (2017). Revisiting the taxonomy of the family Circoviridae: establishment of the genus Cyclovirus and removal of the genus Gyrovirus. Archives of Virology, 162, 1447–1463. doi: 10.1007/s00705-017-3247-y
- Scott, A.N., McNulty, M.S. & Todd, D. (2001). Characterisation of a chicken anaemia virus variant population that resists neutralisation with a group-specific monoclonal antibody. Archives of Virology, 146, 713–728. doi: 10.1007/s007050170141
- Simionatto, S., Lima-Rosa, C.A., Binneck, E., Ravazzolo, A.P. & Canal, C.W. (2006). Characterization and phylogenetic analysis of Brazilian chicken anaemia virus. Virus Genes, 33, 5–10. doi: 10.1007/s11262-005-0033-9
- Snoeck, C.J., Komoyo, G.F., Mbee, B.P., Nakoune, E., Le Faou, A., Okwen, M.P. & Muller, C.P. (2012). Epidemiology of chicken anemia virus in Central African Republic and Cameroon. Virology Journal, 9, 189. doi: 10.1186/1743-422X-9-189
- Tamura, K., Stecher, G., Peterson, D., Filipski, A. & Kumar, S. (2013). MEGA6: Molecular Evolutionary Genetics Analysis version 6.0. Molecular Biology and Evolution, 30, 2725–2729. doi: 10.1093/molbev/mst197
- Todd, D., Mawhinney, K.A. & McNulty, M.S. (1992). Detection and differentiation of chicken anemia virus isolates by using the polymerase chain reaction. Journal of Clinical Microbiology, 30, 1661–1666.
- Todd, D., Scott, A.N., Ball, N.W., Borghmans, B.J. & Adair, B.M. (2002). Molecular basis of the attenuation exhibited by molecularly cloned highly passaged chicken anemia virus isolates. Journal of Virology, 76, 8472–8474. doi: 10.1128/JVI.76.16.8472-8474.2002
- Toro, H., Ramirez, A.M. & Larenas, J. (1997). Pathogenicity of chicken anaemia virus (isolate 10343) for young and older chickens. Avian Pathology, 26, 485–499. doi: 10.1080/03079459708419229
- Trinh, D.Q., Ogawa, H., Bui, V.N., Nguyen, T.T., Gronsang, D., Baatartsogt, T., Kizito, M.K., AboElkhair, M., Yamaguchi, S., Nguyen, V.K. & Imai, K. (2015). Development of a blocking latex agglutination test for the detection of antibodies to chicken anemia virus. Journal of Virological Methods, 221, 74–80. doi: 10.1016/j.jviromet.2015.04.027
- van Santen, V.L., Li, L., Hoerr, F.J. & Lauerman, L.H. (2001). Genetic characterization of chicken anemia virus from commercial broiler chickens in Alabama. Avian Diseases, 45, 373–388. doi: 10.2307/1592977
- Wanasawaeng, W., Buatong, J., Chaichote, S. & Chansiripornchai, N. (2013). Molecular characterization of chicken infectious anemia virus outbreaks during 2008-2011 in Thailand. The Thai Journal of Veterinary Medicine, 43, 497–502.
- Wang, D., Fan, W., Han, G.Z. & He, C.Q. (2009). The selection pressure analysis of chicken anemia virus structural protein gene VP1. Virus Genes, 38, 259–262. doi: 10.1007/s11262-008-0316-z
- Wani, M.Y., Dhama, K., Barathidasan, R., Gowthaman, V., Tiwari, R., Bhatt, P., Mahajan, N.K., Chawak, M.M., Singh, S.D. & Kataria, J.M. (2013). Molecular detection and epidemiology of chicken infectious anaemia virus in India. South Asian Journal of Experimental Biology, 3, 145–151.
- Yamaguchi, S., Imada, T., Kaji, N., Mase, M., Tsukamoto, K., Tanimura, N., Yuasa, N. (2001). Identification of a genetic determinant of pathogenicity in chicken anaemia virus. Journal of General Virology, 82, 1233–1238. doi: 10.1099/0022-1317-82-5-1233
- Yuasa, N. (1992). Effect of chemicals on the infectivity of chicken anaemia virus. Avian Pathology, 21, 315–319. doi: 10.1080/03079459208418846
- Yuasa, N., Taniguchi, T. & Yoshida, I. (1979). Isolation and some characteristics of an agent inducing anemia in chicks. Avian Diseases, 23, 366. doi: 10.2307/1589567
- Zhou, W., Shen, B., Yang, B., Han, S., Wei, L., Xiao, B., Zhou, J. (1997). Isolation and identification of chicken infectious anemia virus in China. Avian Diseases, 41, 361–364. doi: 10.2307/1592190