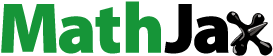
ABSTRACT
Dermanyssus gallinae (De Geer, 1778) is a major problem for the poultry industry worldwide, as it negatively affects virtually all kinds of rearing systems. Therefore, the control of infestation has become a routine process, and its economic cost is constantly increasing. Until now, most of the control strategies have relied on the use of synthetic chemical drugs, but their efficacy is often questioned by the emergence and diffusion of resistant mite populations. With this in mind, the efficacy of λ-cyhalothrin, amitraz, and phoxim has been verified by testing them against 86 mite populations collected from the same number of poultry farms in Italy from 2008 to 2015. Assays were performed according to the filter paper method using the recommended, half, quarter, double and quadruple doses. The results showed that phoxim and amitraz were the most effective acaricides (median efficacies 80.35% and 80.83%, respectively), but amitraz exhibited a sharp fall in its efficacy during 2011 and 2012, while phoxim maintained its high effectiveness up to 2015, when it dropped. The overall median efficacy of λ-cyhalothrin was 58.33%. The data also highlighted the importance of the use of the right concentration, as an increase in dosage was not always useful against resistant populations, while its reduction also diminished efficacy, simultaneously increasing the risk for the development of resistance.
Introduction
The poultry red mite (PRM) Dermanyssus gallinae (De Geer, 1778 ) (Mesostigmata: Dermanyssidae) is a haematophagous mite universally considered a major ectoparasite of poultry, whose infestations are reported from all over the world (Sigognault-Flochlay et al., Citation2017). Recently, infestations by PRM have also been recorded in North America, despite the fact that this area was often considered to be affected by Ornithonyssus sylviarum rather than by D. gallinae (Tomley & Sparagano, Citation2018). In Europe, where the presence of PRM has been recorded for decades, the large majority of poultry farms of laying hens and breeders is infested by D. gallinae, independent of the production systems (enriched cages, barns, free-range, organic or backyard) (Sparagano et al., Citation2009).
Poultry red mites usually produce detrimental effects on health and welfare of infested flocks with an increase in mortality whenever infestation level becomes very high (Kilpinen et al., Citation2005; Mul et al., Citation2009). In recent years, human infestations by D. gallinae have been more frequently reported. Beside the well-established risk that it represents for poultry operators (Cafiero et al., Citation2011), urban cases are being increasingly recorded (Cafiero et al., Citation2008, Citation2018; Navarrete-Dechent & Uribe, Citation2018).
Further concerns are raised by the association between D. gallinae and a number of pathogens, such as Erysipelothrix rhusiopathiae (Chirico et al., Citation2003), Chlamydia psittaci (Circella et al., Citation2011), Tsukamurella spp. (Hubert et al., Citation2017), Coxiella burnetii and Borrelia burgdorferi (Raele et al., Citation2018). Moreover, it has been found involved in the transmission of Salmonella enterica subsp. enterica ser. Enteritidis (Valiente Moro et al., Citation2007) and Gallinarum (Pugliese et al., Citation2018).
Consequently, D. gallinae also has a heavy impact on the poultry system from an economic point of view. It has been estimated that the infestation costs 0.60 € per hen per year, a sum that includes 0.45 € for productivity loss and 0.15 € for treatments (Sigognault-Flochlay et al., Citation2017).
In fact, the management of PRM infestation has become a routine process in intensive poultry farms. Synthetic acaricides are still the most used drugs for the control of the PRM infestation, despite the fact that several alternative methods have been developed in recent years (reviewed in Sparagano et al., Citation2014). However, very few treatments are authorized for administration in poultry farms. Among these, phoxim, an organophosphate, and fluralaner, an isoxazoline-substituted benzamide derivative, are the only substances authorized for use in the presence of animals in most European countries, along with Spinosad (Sigognault-Flochlay et al., Citation2017; Brauneis et al., Citation2018), a mixture of active metabolites produced by the actinobacterium Saccharopolyspora spinosa (Kirst, Citation2010). However, the scandal of summer 2017, when the illegal use of fipronil in poultry farms was uncovered in several European countries (Schuetze, Citation2017), brought to the public opinion the illicit use of unallowed substances, despite the fact that the scientific community already suspected that such practices had already been adopted. In fact, residues of pyrethroids and carbaryl were repeatedly detected in chickens (Marangi et al., Citation2012). Pyrethrins and pyrethroids have been widely used against pests in pets and industrial animal farms for many decades (Beugnet & Franc, Citation2012), but another source of concern was the potential use of amitraz, a substance belonging to the class of the formamidines, which is still intensively used in veterinary medicine for the treatment of ectoparasitoses in cattle, swine, sheep, and dog (Padula, Ponzinibbio, Picco, & Seoane, Citation2012).
Apart from the obvious implications in terms of food contamination and public health, the abuse or misuse of acaricides may also enhance the emergence and diffusion of resistant mite populations (FAO, Citation2012). In fact, drug-resistant populations of D. gallinae are being detected even more frequently, posing a further problem to farmers and, directly or indirectly, to all stakeholders of the poultry system (Sigognault-Flochlay et al., Citation2017). The World Health Organization defined the pest resistance as “an inherited characteristic that imparts an increased tolerance to a pesticide, or a group of pesticides, such that the resistant individuals survive a concentration of the compound(s) that would normally be lethal to the species” (WHO Expert Committee on Vector Biology and Control, Citation1992). Nowadays, the emergence and spread of resistant pest populations is still a major problem worldwide (Sparks & Nauen, Citation2015), which impacts on wide areas of human and veterinary medicine and agriculture. In this scenario, D. gallinae does not represent an exception, as the presence of PRM populations resistant to one or more acaricides has been reported from 1985 (Zeman & Železný, Citation1985) up to the present time (Thomas et al., Citation2018).
In the light of those considerations, the present study analysed the trend in the acaricide susceptibility of PRM populations collected from industrial poultry farms in Italy during an eight-year period. In particular, susceptibility to λ-cyhalothrin (a pyrethroid), amitraz and phoxim was evaluated in order to verify possible fluctuations in the efficacy of acaricide drugs authorized and unauthorized for administration in the poultry farms.
Materials and methods
Mite populations
Mites were collected from industrial poultry farms all across Italy from 2008–2015. The samples were anonymized and sent to the facilities of the Avian Disease Unit of the Department of Veterinary Medicine of the University of Bari. All samples were shipped in refrigerated boxes and, upon arrival, mites were starved at room temperature in 5% CO2 for 5 days. After starvation, the viability of mites was visually inspected and groups with less than approximately 50% of live mites were excluded from the investigation. Overall, 86 mite populations, collected from the same number of poultry farms, were tested in this study ().
Table 1. Number of Dermanyssus gallinae populations analyzed by year.
Before performing assays, 10 mites from each sample were randomly selected and morphologically inspected to confirm the D. gallinae identification according to the keys of Varma (Citation1993) and Baker (Citation1999).
Efficacy test
Mites were tested against λ-cyhalothrin (Oxyfly® 10 CS, Novartis Animal Health, Basel, Switzerland), amitraz (TakTik125®, Farmaceutici Gellini, Aprilia, Italy) and phoxim (ByeMite®, Bayer Animal Health, Leverkusen, Germany).
The drug efficacy was assessed by the filter paper technique as described by Thind & Muggleton (Citation1998) with slight modifications. Specifically, two pieces of filter paper were impregnated with 200 µl of acaricide solution; then 20 mites were collected and distributed by using a small brush on the filter paper. The first piece of paper was covered by the second, and they were both tightened between two plastic enclosing layers, sealed with vinylic glue. Once prepared, these “cells” were incubated at 20°C and 60% relative humidity for 24 h; afterward, they were opened and live and dead mites were counted by the aid of a stereomicroscope. Moribund mites were considered inactive, and therefore dead. Control cells were assembled with water instead of acaricide solution. Each assay and the relative control test was performed in triplicate.
Mites were exposed to five different concentrations of acaricides. The field concentration (1×) was defined according to the pesticide label. Namely, 1× λ-cyhalothrin was 0.5 g/l, and 1× phoxim was 2 g/l. Since amitraz was not labelled for poultry, and different concentrations were reported for bovine (0.25 g/l), swine (0.5 g/l) and sheep (0.5 g/l), the higher dosage was considered (0.5 g/l). Considering that 7 cm2 of paper were impregnated with 200 µL of solution, 1x concentration corresponded to 0.14 g/m2 for λ-cyhalothrin and amitraz, and 0.57 g/m2 for phoxim.
The other tested concentrations were: two-fold (2x, specifically 1 g/l λ-cyhalothrin, 1 g/l amitraz and 4 g/l phoxim), four-fold (4x, specifically 2 g/l λ-cyhalothrin, 2 g/l amitraz and 8 g/l phoxim), half (0.5×, specifically 0.25 g/l λ-cyhalothrin, 0.25 g/l amitraz and 1 g/l phoxim), and one quarter (0.25×, specifically 0.125 g/l λ-cyhalothrin, 0.125 g/l amitraz and 0.5 g/l phoxim) of the field concentration. Those specific concentrations were chosen because of their proximity to those potentially applied in the field.
For each group of three replicates, the mean and the 95% confidence interval (CI) were calculated. Whenever the CI was greater than 2% of the mean value, the group of data was discarded.
The percent efficacy was calculated according to Abbott's formula (Abbott, Citation1925), modified as follows to consider the mean of the three replicates.When mortality was greater than 20% in the control group, the test was rejected (WHO, Citation2009).
Statistical analysis
The efficacy data were grouped and compared by acaricide, by year, by four-year period and by concentration. Furthermore, five efficacy classes (EC), namely 0–20%, 21–40%, 41–60%, 61–80%, and 81–100%, were established and mite populations were allocated to them according to the interval where their efficacy fell.
Populations included in the 81–100% and 61–80% EC were considered highly susceptible and susceptible, respectively, those included within the 41–60% EC were considered intermediate, those falling within 0–20% and 21–40% EC were considered highly and moderately resistant, respectively.
The Shapiro–Wilk test was applied to all groups of data to ascertain their normal distribution, with α-level for rejecting the null hypothesis equal to 0.05. Since the great majority of data showed a non-normal distribution, medians and their respective 95% CI were calculated. Comparisons among groups were performed by using the nonparametric, two-tailed Mann–Whitney U test.
The ECs were compared using two-tailed Fisher's exact test. In both cases, differences were considered significant when P < 0.05.
All statistical analyses were performed in R (R Core Team, Citation2018).
Results
Efficacy of λ-cyhalothrin, amitraz, and phoxim against poultry red mite
Considering the entire period of the survey and the field concentration (), the λ-cyhalothrin was found to be the least effective drug, with an overall median efficacy of 58.33% (CI: 48.33–68.33%). The efficacies of amitraz and phoxim were significantly (P < 0.001, Table S8) higher when compared to λ-cyhalothrin, as they killed 80.33% (CI: 71.25–88.33%) and 80.35% (CI:75.00–91.67%) of mites, respectively, without any significant (P = 0.523, Table S8) difference between them. Such a trend was confirmed by analyzing the ECs (), because the distribution for amitraz and phoxim was right-skewed toward the higher efficacy classes, while the central value for λ-cyhalothrin fell into the central class (41–60%) (Figure S1).
Table 2. Percent efficacy of the acaricide drugs at field concentration, by year.
Table 3. Distribution of mite populations according to the efficacy of acaricides at field concentration (0.5 g/l λ-cyhalothrin, 0.5 g/l amitraz, 2 g/l phoxim).
Fisher's exact test confirmed the significance of the differences in the distribution (P < 0.001 and P = 0.001, respectively).
When the four-year periods were considered, the efficacies of λ-cyhalothrin and amitraz were significantly lower (P = 0.002 and P = 0.001, respectively, Table S8) in the tested mite populations during the 2012–2015 period with respect to the previous period (). The EC distribution reflected this trend, as a marked shift was observed from susceptibility to high and moderate resistance to λ-cyhalothrin and amitraz (P = 0.020 and P = 0.003, respectively, , Table S8, Figure S2). Neither median efficacy, nor EC distribution changed significantly for phoxim (P = 0.436 and P = 0.283 between 2008–2011 and 2012–2015, respectively).
Those facts indicated that phoxim and amitraz efficacies against the tested populations were comparable during the first four-year period (P = 0.293, Table S8), but not during 2012–2015 (P = 0.020, Table S8). Considering the EC distribution for phoxim, no evident changes were observed, as the number of resistant and susceptible populations remained roughly the same (P = 0.283, , Table S8).
Annual trends in drug efficacy
The analysis of annual trends added new elements (). While in 2008 and 2009 no amitraz-resistant populations were detected, 4.55% of the mite populations tested in 2010 were moderately resistant (). In the same years, the median efficacy of phoxim did not vary significantly () but, in 2010, 4.17% of the tested mite populations were moderately resistant and 8.33% were highly resistant (Table 3).
Figure 1. Trends of the median percent efficacy of λ-cyhalothrin (white bars), amitraz (dotted bars), and phoxim (grey bars) at the field concentration (0.5 g/l λ-cyhalothrin, 0.5 g/l amitraz, 2 g/l phoxim) by year.
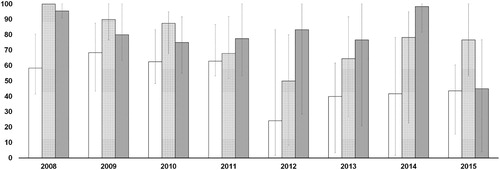
During 2011, efficacy of amitraz against tested populations decreased from 87.50% (CI: 67.50–95%) to 67.92% (CI: 51.67–91.84%) with sufficient statistical relevance (P = 0.051, , Table S8). Median efficacy of both λ-cyhalothrin and phoxim did not vary significantly in 2011 with respect to 2010 (P = 0.696 and P = 0.774, respectively, , Table S8), and neither did their EC distribution (P = 0.829 and P = 1, respectively, , Table S8).
The mite populations tested during 2012 were more resistant to λ-cyhalothrin and amitraz, making the efficacy of these drugs decrease (P = 0.015 and P = 0.023, respectively, , Table S8). Specifically, 83.33% of the tested mite populations were moderately to highly resistant to λ-cyhalothrin, and 42.86% of them were resistant (moderately or highly) to amitraz. No mite population was found highly susceptible to the latter acaricide (). In contrast, the median of the phoxim killing rate did not vary significantly (P = 0.574, Table S8) in 2012 compared to 2011. No population was found highly resistant to phoxim.
During 2013, the recorded efficacies of the λ-cyhalothrin and amitraz numerically improved. In particular, the latter returned, in terms of killing rate, at a comparable level with phoxim (P = 0.575, Table S8).
Mite populations collected during 2014 were neither intermediate, nor resistant to phoxim, and 88.89% were highly susceptible and 11.11% susceptible (). Considering that the efficacy of λ-cyhalothrin and amitraz did not vary significantly with respect to the previous year (P = 0.689 and P = 0.859, Table S8), phoxim was the most effective drug (P = 0.005 against λ-cyhalothrin and P = 0.025 against amitraz, , Table S8) against the tested populations.
However, during 2015, the phoxim efficacy significantly (P = 0.007) fell to 45% (CI: 3.89–76.67%). Only 40% of the tested mite populations were susceptible or highly susceptible to the acaricide, with a significant (P = 0.023, Table S8) discrepancy with the data of the previous year. Remarkably, no significant difference was observed between phoxim efficacy and λ-cyhalothrin efficacy (P = 1.000, Table S8).
Drug efficacy in relation to the concentration
The relation between the concentration of drugs and their efficacy was variable. Considering all the tested mite populations, the two- and four-fold increases of λ-cyhalothrin (Table S1) concentration did not significantly improve its efficacy (P = 0.288 and P = 0.212, respectively, Table S8), against the tested populations, as well as the two-fold reduction (P = 0.207, Table S8). Only when diluted four times, λ-cyhalothrin was significantly less effective (P = 0.004, Table S8).
In contrast, the concentration considerably affected amitraz activity (Table S2), as its killing rate increased to median values of 90.00% (CI: 85.00–95.00%) and 96.67% (CI: 91.67–98.33%) when the field concentration was doubled and quadrupled, respectively, and it decreased to 66.67% (CI: 56.67–75%) and 60.00% (CI: 48.33–70.00%) when concentration was reduced two- and four-fold, respectively. The statistical significance of such differences was always high (P = 0.049, P < 0.001, P = 0.004 and P < 0.001, respectively, Table S8). However, in 2012, when amitraz efficacy dropped to the minimum point, the increase in concentration did not increase mortality rate of mites, as medians were 31.67% (CI: 5.00–90.00%) and 33.33% (CI: 16.97–91.67%) at 2× and 4× concentrations, respectively (P = 0.443 and P = 0.609, Tables S2 and S8).
Conversely, only the quadruplication of phoxim concentration significantly (P = 0.039, Table S8) improved its efficacy (Table S3) from 80.35% (CI: 75.00–91.67%) to 90% (CI: 81.67–98.33%). When phoxim concentration was reduced to 0.5× and 0.25×, its efficacy was significantly reduced to 72.50% (CI: 61.67–80.00%) and 62.91%, (CI: 56.67–72.50%) with P = 0.025 and P < 0.001, respectively (Table S8). Also in this case, in 2015, when the tested populations were more resistant to phoxim, no significant efficacy improvement was observed when concentration was doubled and quadrupled (P = 0.421 and P = 0.841, respectively, Tables S3 and S8).
By analyzing the four-year periods, no remarkable association was found between susceptibility trends and variations in concentration. However, it is noteworthy that, during the period 2012–2015, when phoxim activity was the highest among the three tested drugs, the efficacies of 2× and 4× amitraz concentrations were comparable to 1× phoxim (P = 0.535 and P = 0.103, Tables S2, S3 and S8).
Discussion
The results from this investigation underlined that the detection of resistant mite populations was a common issue that pertains to all tested acaricides. In fact, two out of the three tested drugs, namely λ-cyhalothrin and amitraz, exhibited a significant decrease in efficacy against the populations collected during the four-year period 2012–2015, especially compared with those from 2008–2011. Phoxim effects were more constant in time, but highly resistant populations were detected in 2015.
The most remarkable contrast was observed for amitraz, which killed more than 80% of mites in 61.82% of populations during the first four-year period but inactivated less than 80% of mites in 74% of populations during the next four years.
The decrease in efficacy of λ-cyhalothrin was equally sizeable, but less evident because of the low susceptibility of the tested populations since the first years of investigation. On the other hand, the phoxim killing rate remained mainly constant in the two four-year periods even considering the drop observed in 2015.
There are some hypotheses for those trends. It is well recognized that the emergence and spread of resistant populations are largely due to the abuse or misuse of drugs, which exert a selective pressure that promotes the survival of resistant individuals (FAO, Citation2012; Coles & Dryden, Citation2014). It is no accident that λ-cyhalothrin exhibited the lowest efficacy towards PRM, as it belongs to the pyrethroid family, one of the first insecticide classes. Pyrethroids, such as λ-cyhalothrin and bifenthrin, were largely used in poultry farms to fight the house fly Musca domestica L. (Abbas et al., Citation2016), and this may have contributed to selection of resistant individuals of D. gallinae, too. This could account for the early rise of resistant D. gallinae populations, which was firstly documented more than 20 years ago (Beugnet et al., Citation1997) and it has been repeatedly reported from mites collected in poultry farms (Nordenfors et al., Citation2001; Thomas et al., Citation2018). A similar trend was observed for O. sylviarum populations collected in the field in California (Mullens et al., Citation2017).
Data from amitraz are more controversial. An early report found PRM quite tolerant to amitraz (Fletcher & Axtell, Citation1991), while Marangi et al. (Citation2009) found amitraz sufficiently effective to kill 100% of mites belonging to different field populations. Similarly, data from this study evidenced very low resistance levels up to 2011, but, during 2011 and 2012, the proportion of resistant groups increased, whereas susceptible and highly susceptible populations declined. This may indicate that D. gallinae recurrently came into contact with amitraz between the end of the 2000s and the beginning of the 2010s, perhaps because of its illicit use as an acaricide in poultry farms. The unauthorized administration of amitraz in poultry has often been suspected but, to our knowledge, no investigation was carried out to verify it, at least in the years covered by this investigation. Indirect evidence may be inferred by the detection of resistance in cattle ticks Boophilus microplus (Li et al., Citation2004). Amitraz is largely used in cattle and a reduction in susceptibility of their ectoparasites is expected. Conversely, if amitraz was not applied in poultry, it would be unusual to find resistant populations, as it has been reported that amitraz resistance is uncommon in absence of selective pressure (Jonsson & Hope, Citation2007). As a partial confirmation of such a hypothesis, there is the recent detection of amitraz residues in two samples of eggs from Italy (Reich & Triacchini, Citation2018). In the same report, fipronil was also detected. Previously, residues deriving from other acaricides, such as carbamates, organophosphates, and pyrethroids, were found in poultry products (Ivey et al., Citation1984; Szerletics-Túri et al., Citation2000; Marangi et al., Citation2012). On aggregate, those data suggest that the illegal use of unapproved pesticides could have sometimes been practiced by farmers, thus contributing to the emergence of resistant D. gallinae populations. It should be emphasized that the only acaricides authorized for use in Italy in presence of hens are phoxim (since 2009), spinosad (since 2011) and, more recently, fluralaner. Other drugs, such as amitraz and fipronil, are not labelled for being applied in poultry, due to their toxicity and their residual activity. Additionally, authorization for the use of carbaryl, employed in the past, was withdrawn in 2007, but the retrieval of residuals of amitraz or carbaryl (Marangi et al., Citation2012) in aviary products from Italy led us to hypothesize that some farmers were still using it during the years covered by this investigation.
In the light of those considerations, it is tempting to speculate that the sudden decline of amitraz efficacy during 2011 and 2012 might be consequent to a selection process caused by repeated contact with amitraz, probably inappropriately dispensed. In fact, it is reasonable to assume that dosing, application mode, and administration schedule might have been improperly, or at least empirically, devised when unauthorized drugs were handled.
Conversely, out of the three tested drugs, phoxim was the only one authorized for use in the presence of animals in poultry farms of Italy, and its operating procedures were adequately conceived and set up, especially in terms of dosage and administration schedule. This fact may have helped to keep low the proportion of resistant groups of D. gallinae, insomuch that less susceptible populations have recently been detected (Thomas et al., Citation2018). In this investigation, phoxim-resistant populations were only found in 2015, and it is possible that factors other than contacts with acaricides should be intervened.
A list of elements affecting the susceptibility of pests was scrutinized by the Food and Agriculture Organization of the United Nations (FAO, Citation2012), which grouped them into three major categories: biological (i.e. population size, reproductive potential, dispersal, pesticide metabolism, number of target sites of pesticides, host range, etc.), genetic (occurrence of resistance genes, resistance mechanisms, fitness of resistant individuals, cross-resistance, past selection, etc.) and operational (activity spectrum of the pesticide, pesticide application rate, application coverage, treatment frequency, etc.). By matching those elements to the known biological and physiological features of D. gallinae, it is clear that the potential for resistance development is very high, as PRM is characterized by a very high population size, high reproductive potential, great dispersal capability, and a relatively wide potential host range (George et al., Citation2015). On the other hand, most of the substances tested in this study are active against a specific target site, as well as many commercially available drugs. Specifically, pyrethroids and DDT target the sodium channels, carbamates, and organophosphates are directed against acetylcholinesterase (David et al., Citation2013), and amitraz binds the octopamine receptor (Beugnet & Franc, Citation2012). Potentially, their specificity is an important factor for the emergence of resistant mites.
Considering that those factors cannot be directly modified, proper management acquires great relevance to the control of the infestation. Among operational aspects, the pesticide application rate is one of the most important. According to FAO (Citation2012), if pesticides are used following the label instructions, then the risk of resistance development is lower because heterozygotes (assuming that the potential resistant genes are incompletely dominant) are killed, while they might survive if the application rate is below the recommended dose. On the other hand, if the pesticide is applied at higher doses than required, few homozygous resistant individuals may survive and reproduce, biasing the selection process toward the more resistant mites and thus producing less susceptible populations. The herein collected data about the relationship between drug efficacy and concentration strongly suggest that resistant populations were not affected even at higher concentrations, allowing us to infer that they might be composed of homozygous individuals, resistant to high levels of drugs.
In particular, the activity of λ-cyhalothrin, whose efficacy was generally low, was not affected by its concentration, as it was only more effective against D. gallinae populations when its concentration was increased four times. In contrast, amitraz was much more influenced by the concentration, as resistant populations were usually killed when it was administered at double or quadruple concentration. It is noteworthy that, in 2012, when most of the tested populations were resistant or, at least, intermediate, the rise in concentration was not as equally effective, probably because they had yet developed high resistance levels. Similar considerations may be replicated for phoxim. Its efficacy was found to be directly related to its dosage, despite the label concentration usually being effective enough to kill more than 80% of mites. However, even for phoxim, the data for 2015 showed that no significant effects were obtained by increasing the concentration, as highly resistant populations were developed.
Apart from this exception, it is clear that the label concentration of phoxim was actually the most effective, underlining the pivotal role of the preliminary studies aimed to assess the right dose to be administered, insomuch that every variation in concentration becomes useless or even counterproductive.
All considered, it appears evident that only integrated management (Tomley & Sparagano, Citation2018) makes possible effective control of D. gallinae infestation, also contributing to maintaining a low risk of emergence of resistant mite populations. This approach is aimed to find the correct equilibrium among all factors that act in a poultry farm by, among other, implementing good hygiene practices, avoiding overcrowding, carefully controlling the environmental conditions (i.e. light, humidity and temperature) and adopting a pest control strategy that would alternate more than one synthetic drug and other natural acaricides. A major limitation is the small number of available and authorized substances that could be used against D. gallinae in poultry farms. Fortunately, research is providing some encouraging results. For example, fluralaner was recently authorized for use in the presence of animals (Brauneis et al., Citation2018), and interesting data are emerging from tests with the neem oil, an essential oil that has been found to be active against D. gallinae (Camarda et al., Citation2018). Therefore, the range of available products is widening, and this may encourage stakeholders to adopt well-differentiated strategies for fighting D. gallinae, in an effort to reduce infestation, prevent the emergence of resistance, and even protect the environment by keeping to a minimum the introduction of hazardous substances.
Supplemental Material
Download Zip (250.3 KB)Acknowledgement
The study was carried out within the EU COST Action FA1404 “Improving current understanding and research for sustainable control of the poultry red mite Dermanyssus gallinae (COREMI)”.
Disclosure statement
No potential conflict of interest was reported by the authors.
ORCID
Nicola Pugliese http://orcid.org/0000-0001-9342-9516
Elena Circella http://orcid.org/0000-0001-9625-2920
Antonio Camarda http://orcid.org/0000-0002-3961-585X
References
- Abbas, N., Shah, R.M., Shad, S.A., Iqbat, N. & Razaq, M. (2016). Biological trait analysis and stability of lambda-cyhalothrin resistance in the house fly, Musca domestica L. (Diptera: Muscidae). Parasitology Research, 115, 2073–2080. doi: 10.1007/s00436-016-4952-2
- Abbott, W.S. (1925). A method of computing the effectiveness of an insecticide. Journal of Economic Entomology, 18, 265–267. doi: 10.1093/jee/18.2.265a
- Baker, A.S. (1999). Mites and ticks of domestic animals: an identification guide and information source. London: The Natural History Museum, The Stationery Office.
- Beugnet, F., Chauve, C., Gauthey, M. & Beert, L. (1997). Resistance of the red poultry mite to pyrethroids in France. Veterinary Record, 140, 577–579. doi: 10.1136/vr.140.22.577
- Beugnet, F. & Franc, M. (2012). Insecticide and acaricide molecules and/or combinations to prevent pet infestation by ectoparasites. Trends in Parasitology, 28, 267–279. doi: 10.1016/j.pt.2012.04.004
- Brauneis, M.D., Zoller, H., Williams, H., Zschiesche, E. & Heckeroth, A.R. (2018). The acaricidal speed of kill of orally administered fluralaner against poultry red mites (Dermanyssus gallinae) on laying hens and its impact on mite reproductions. Parasites & Vectors, 10, 594. doi: 10.1186/s13071-017-2534-5
- Cafiero, M.A., Camarda, A., Circella, E., Santagada, G., Schino, G. & Lomuto, M. (2008). Pseudoscabies caused by Dermanyssus gallinae in Italian city dwellers: a new setting for an old dermatitis. Journal of the European Academy of Dermatology and Venereology, 22, 1382–1383. doi: 10.1111/j.1468-3083.2008.02645.x
- Cafiero, M.A., Galante, D., Camarda, A., Giangaspero, A. & Sparagano, O. (2011). Why dermanyssosis should be listed as an occupational hazard. Occupational and Environmental Medicine, 68, 628. doi: 10.1136/oemed-2011-100002
- Cafiero, M.A., Viviano, E., Lomuto, M., Raele, D.A., Galante, D. & Castelli, E. (2018). Dermatitis due to Mesostigmatic mites (Dermanyssus gallinae, Ornithonyssus [O.] bacoti, O. bursa, O. sylviarum) in residential settings. Journal of the German Society of Dermatology, 16, 904–906.
- Camarda, A., Pugliese, N., Bevilacqua, A., Circella, E., Gradoni, L., George, D., Sparagano, O. & Giangaspero, A. (2018). Efficacy of a novel neem oil formulation (RP03TM) to control the poultry red mite Dermanyssus gallinae. Medical and Veterinary Entomology, 32, 290–297. doi: 10.1111/mve.12296
- Chirico, J., Eriksson, H., Fossum, O. & Jansson, D. (2003). The poultry red mite, Dermanyssus gallinae, a potential vector of Erysipelothrix rhusiopathiae causing erysipelas in hens. Medical and Veterinary Entomology, 17, 232–234. doi: 10.1046/j.1365-2915.2003.00428.x
- Circella, E., Pugliese, N., Todisco, G., Cafiero, M.A., Sparagano, O.A. & Camarda, A. (2011). Chlamydia psittaci infection in canaries heavily infested by Dermanyssus gallinae. Experimental and Applied Acarology, 55, 329–338. doi: 10.1007/s10493-011-9478-9
- Coles, T.B. & Dryden, M.W. (2014). Insecticide/acaricide resistance in fleas and ticks infesting dogs and cats. Parasites & Vectors, 7, 8. doi: 10.1186/1756-3305-7-8
- David, J.-P., Ismail, H.M., Chandor-Proust, A. & Paine, M.J.I. (2013). Role of cytochrome P450s in insecticide resistance: impact on the control of mosquito-borne diseases and use of insecticides on Earth. Philosophical Transactions of the Royal Society of London. Series B Biological Sciences, 368, 20120429. doi: 10.1098/rstb.2012.0429
- European Food Safety Authority (EFSA), Reich, H. & Triacchini, G.A. (2018). Occurrence of residues of fipronil and other acaricides in chicken eggs and poultry muscle/fat. EFSA Journal, 16, 5164.
- Fletcher, M.G. & Axtell, R.C. (1991). Susceptibilities of northern fowl mite, Ornithonyssus sylviarum (Acarina: Macronyssidae), and chicken mite, Dermanyssus gallinae (Acarina: Dermanyssidae), to selected acaricides. Experimental and Applied Acarology, 13, 137–142. doi: 10.1007/BF01193664
- Food and Agriculture Organization of the United Nations (FAO). (2012). International code of conduct on the distribution and use of pesticides. Guidelines on prevention and management of pesticide resistance. Retrieved from http://www.fao.org/fileadmin/templates/agphome/documents/Pests_Pesticides/Code/FAO_RMG_Sept_12.pdf.
- George, D.R., Finn, R.D., Graham, K.M., Mul, M.F., Maurer, V., Moro, C. V. & Sparagano, O.A.E. (2015). Should the poultry red mite Dermanyssus gallinae be of wider concern for veterinary and medical science? Parasites & Vectors, 8, 178. doi: 10.1186/s13071-015-0768-7
- Hubert, J., Erban, T., Kopecky, J., Sopko, B., Nesvorna, M., Lichovnikova, M., Schicht, S., Strube, C. & Sparagano, O. (2017). Comparison of microbiomes between red poultry mite populations (Dermanyssus gallinae): predominance of Bartonella-like bacteria. Microbial Ecology, 74, 947–960. doi: 10.1007/s00248-017-0993-z
- Ivey, M.C., Wayne Ivie, G., Devaney, G. & Beerwinkle, K.R. (1984). Residues of carbaryl and two of its metabolites in eggs of laying hens treated with Sevin® for Northern fowl mite control by dipping. Poultry Science, 63, 61–65. doi: 10.3382/ps.0630061
- Jonsson, N.N. & Hope, M. (2007). Progress in the epidemiology and diagnosis of amitraz resistance in the cattle tick Boophilus microplus. Veterinary Parasitology, 146, 193–198. doi: 10.1016/j.vetpar.2007.03.006
- Kilpinen, O., Roepstorff, A., Permin, A., Nørgaard-Nielsen, G., Lawson, L.G. & Simonsen, H.B. (2005). Influence of Dermanyssus gallinae and Ascaridia galli infections on behaviour and health of laying hens (Gallus gallus domesticus). British Poultry Science, 46, 26–34. doi: 10.1080/00071660400023839
- Kirst, H.A. (2010). The spinosyn family of insecticides: realizing the potential of natural products research. The Journal of Antibiotics, 63, 101–111. doi: 10.1038/ja.2010.5
- Li, A.Y., Davey, R.B., Miller, R.J. & George, J.H. (2004). Detection and characterization of amitraz resistance in the Southern cattle tick, Boophilus microplus (Acari: Ixodidae). Journal of Medical Entomology, 41, 193–200. doi: 10.1603/0022-2585-41.2.193
- Marangi, M., Cafiero, M.A., Capelli, G., Camarda, A., Sparagano, O.A.E. & Giangaspero, A. (2009). Evaluation of the poultry red mite, Dermanyssus gallinae (Acari: Dermanyssidae) susceptibility to some acaricides in field populations from Italy. Experimental and Applied Acarology, 48, 11–18. doi: 10.1007/s10493-008-9224-0
- Marangi, M., Morelli, V., Pati, S., Camarda, A., Cafiero, M.A., Giangaspero, A., & Kaltenboeck, B. (2012). Acaricide residues in laying hens naturally infested by red mite Dermanyssus gallinae. PLoS One, 7, e31795. doi: 10.1371/journal.pone.0031795
- Mul, M., Van Niekerk, T., Chirico, J., Maurer, V., Kilpinen, O., Sparagano, O., Thind, B., Zoons, J., Moore, D., Bell, B., Gjevre, A.G. & Chauve, C. (2009). Control methods for Dermanyssus gallinae in systems for laying hens: results of an international seminar. World’s Poultry Science Journal, 65, 589–600. doi: 10.1017/S0043933909000403
- Mullens, B.A., Murillo, A.C., Zoller, H., Heckeroth, A.R., Jirjis, F. & Flochlay-Sigognault, A. (2017). Comparative in vitro evaluation of contact activity of fluralaner, spinosad, phoxim, propoxur, permethrin and deltamethrin against the northern fowl mite, Ornithonyssus sylviarum. Parasites & Vectors, 10, 358. doi: 10.1186/s13071-017-2289-z
- Navarrete-Dechent, C. & Uribe, P. (2018). A case of gamasidosis caused by Dermanyssus gallinae, misdiagnosed as delusional parasitosis. Clinical and Experimental Dermatology, 43, 950–952. doi: 10.1111/ced.13687
- Nordenfors, H., Höglund, J., Tauson, R. & Chirico, J. (2001). Effect of permethrin impregnated plastic strips on Dermanyssus gallinae in loose-housing system for laying hens. Veterinary Parasitology, 102, 121–131. doi: 10.1016/S0304-4017(01)00528-3
- Padula, G., Ponzinibbio, M.V., Picco, S. & Seoane, A. (2012). Assessment of the adverse effects of the acaricide amitraz: in vitro evaluation of genotoxicity. Toxicology Mechanisms and Methods, 22, 657–661. doi: 10.3109/15376516.2012.666683
- Pugliese, N., Circella, E., Marino, M., De Virgilio, C., Cocciolo, G., Lozito, P., Cafiero, M.A. & Camarda, A. (2018). Circulation dynamics of Salmonella enterica subsp. enterica ser. Gallinarum biovar Gallinarum in a poultry farm infested by Dermanyssus gallinae. Medical and Veterinary Entomology, in press.
- Raele, D.A., Galante, D., Pugliese, N., La Salandra, M., Lomuto, M. & Cafiero, M.A. (2018). First report of Coxiella burnetii and Borrelia burgdorferi sensu lato in poultry red mites, Dermanyssus gallinae (Mesostigmata, Acari), related to urban outbreaks of dermatitis in Italy. New Microbes and New Infections, 22, 103–109. doi: 10.1016/j.nmni.2018.01.004
- R Core Team. (2018). R: a language and environment for statistical computing. Vienna: R Foundation for Statistical Computing. Retrieved from https://www.R-project.org
- Schuetze, C.F. (2017). Tainted eggs prompt scare in Europe. The New York Times. Retrieved from https://www.nytimes.com/2017/08/07/world/europe/eggs-belgium-germany-netherlands.html
- Sigognault-Flochlay, A., Thomas, E. & Sparagano, O. (2017). Poultry red mite (Dermanyssus gallinae) infestation: a broad impact parasitological disease that still remains a significant challenge for the egg-laying industry in Europe. Parasites & Vectors, 10, 357. doi: 10.1186/s13071-017-2292-4
- Sparagano, O., Pavlićević, A., Murano, T., Camarda, A., Sahibi, H., Kilpinen, O., Mul, M., van Emous, R., le Bouquin, S., Hoel, K. & Cafiero, M.A. (2009). Prevalence and key figures for the poultry red mite Dermanyssus gallinae infestations in poultry farm systems. Experimental and Applied Acarology, 48, 3–10. doi: 10.1007/s10493-008-9233-z
- Sparagano, O.A.E., George, D.R., Harrington, D.W.J. & Giangaspero, A. (2014). Significance and control of the poultry red mite, Dermanyssus gallinae. Annual Review of Entomology, 59, 447–466. doi: 10.1146/annurev-ento-011613-162101
- Sparks, T.C. & Nauen, R. (2015). IRAC: mode of action classification and insecticide resistance management. Pesticide Biochemistry and Physiology, 121, 122–128. doi: 10.1016/j.pestbp.2014.11.014
- Szerletics-Túri, M., Soós, K. & Végh, E. (2000). Determination of residues of pyrethroids and organophosphorous ectoparasiticides in foods of animal origin. Acta Veterinaria Hungarica, 48, 139–149.
- Thind, B.B. & Muggleton, J. (1998). A new bioassay method for the detection of resistance to pesticides in the stored product mite Acarus siro (Acari: Acaridae). Experimental and Applied Acarology, 22, 543–552. doi: 10.1023/A:1006037807498
- Thomas, E., Zoller, H., Liebisch, G., Alves, L.F.A., Vettorato, L., Chiummo, R.M. & Sigognault-Flochlay, A. (2018). In vitro activity of fluralaner and other commonly used acaricides against Dermanyssus gallinae isolates from Europe and Brazil. Parasites & Vectors, 11, 361. doi: 10.1186/s13071-018-2956-8
- Tomley, F.M. & Sparagano, O. (2018). Spotlight on avian pathology: red mite, a serious emergent problem in layer hens. Avian Pathology, 47, 533–535. doi: 10.1080/03079457.2018.1490493
- Valiente Moro, C., Chauve, C. & Zenner, L. (2007). Experimental infection of Salmonella Enteritidis by the poultry red mite, Dermanyssus gallinae. Veterinary Parasitology, 146, 329–336. doi: 10.1016/j.vetpar.2007.02.024
- Varma, M.G.R. (1993). Ticks and mites (Acari). In R.P. Lane & R.W. Crosskey (Eds.), Medical Insects and Arachnids (pp. 597–658). Cambridge: University Press.
- WHO. (2009). Guidelines for efficacy testing of insecticides for indoor and outdoor ground-applied space spray applications. Geneva: World Health Organization.
- WHO Expert Committee on Vector Biology and Control. (1992). Vector resistance to pesticides: fifteenth report of the WHO Expert Committee on Vector Biology and control. Geneva: World Health Organization.
- Zeman, P. & Železný, J. (1985). The susceptibility of the poultry red mite, Dermanyssus gallinae (De Geer, 1778), to some acaricides under laboratory conditions. Experimental and Applied Acarology, 1, 17–22. doi: 10.1007/BF01262196