ABSTRACT
To determine whether geese are susceptible to infection by avian leukosis virus (ALV), 702 serum samples from domestic and foreign goose breeds were screened for p27 antigen as well as being inoculated into DF-1 cell cultures to isolate ALV. Although 5.7% of samples were positive for p27 antigen, reactivity appeared to be non-specific because no ALV was detected in the corresponding DF-1 cultures. To further determine whether geese are susceptible to ALV-J isolated from chickens, ALV-J strain JS09GY7 was artificially inoculated into 10-day-old goose embryos, with one-day-old hatched goslings then screened for p27 antigen and the presence of ALV. In all cases, the results of both tests were negative. Liver tissues from the 1-day-old goslings were screened using a polymerase chain reaction-based assay, which failed to amplify ALV-J gene fragments from any of the samples. Further, no histopathological damage was observed in the liver tissues. ALV-J was further inoculated intraperitoneally into one-day-old goslings, with cloacal swabs samples and plasma samples then collected every 5 days for 30 days. All samples were again negative for the presence of p27 antigen and ALV, and liver tissues from the challenged geese showed no histopathological damage and were negative for the presence of ALV-J gene fragments. Furthermore, p27 antigen detection, PCR-based screening, and indirect immunofluorescence assays were all negative following the infection of goose embryo fibroblasts with ALV-J. Together, these results confirm that virulent chicken-derived ALV-J strains cannot infect geese, and that p27 antigen detection in goose serum is susceptible to non-specific interference.
Introduction
Avian leukosis is a transmissible benign or malignant neoplastic disease caused by avian leukosis virus (ALV). Symptoms include serious immunosuppression, growth retardation, and tumour-induced mortality. ALV infections have been reported worldwide and have caused severe economic losses to the poultry industry (Weiss & Vogt, Citation2011; Payne & Nair, Citation2012). ALVs are classified into 10 subgroups (A-J) on the basis of the envelope glycoprotein, which is responsible for specific viral interference patterns, virus neutralization, and host range (Payne & Nair, Citation2012). In 2012, a new ALV subgroup was identified and was subsequently designated subgroup K. Subgroups A, B, C, D, J, and K are exogenous viruses, while members of the ALV subgroups E, F, G, H, and I are endogenous viruses (Payne et al., Citation1991; McNally et al., Citation2010; Zhao et al., Citation2018).
Since the first report of avian leukosis virus subgroup J (ALV-J) in the United Kingdom in 1988, the virus has rapidly spread to many other countries, including China (Wang & Juan, Citation2002; Sun & Cui, Citation2007; Wu et al., Citation2010; Payne & Nair, Citation2012). At present, the host range of ALV-J is noticeably expanding (Zeghdoudi et al., Citation2017), with studies showing that all chicken varieties are now susceptible to ALV-J (Dimcheff et al., Citation2000; Li et al., Citation2018). ALV-J infection causes vascular and visceral neoplasms, decreased egg production, stunted growth, and increased mortality (Sun & Cui, Citation2007; Wang et al., Citation2013), resulting in huge economic losses. As such, ALV-J is considered a serious threat to the poultry industry.
Mutation and recombination within the ALV genome results in expansion of the host range (Payne, Howes et al., Citation1992). Previous molecular epidemiological studies have mainly focused on poultry, with recent reports of endogenous ALV-A, ALV-B, and ALV-J viruses in wild birds (Li et al., Citation2013). Studies have also confirmed that various ALV and gag gene fragments have been amplified from budgerigars and 26 species of Galliformes respectively (Payne, Gillespie et al., Citation1992). Shen et al. found that mutations within the genomes of ALV-J strains cause host range expansion (Shen et al., Citation2014). Recent studies have confirmed the presence of ALV-A and ALV-B infections in wild birds from Northeast China (Li et al., Citation2013), while in 2014, separate studies amplified ALV-E genes and isolated ALV-J from wild ducks in China (Hao et al., Citation2014; Zeng et al., Citation2014). Interestingly, in 2015, it was shown that there is no ALV-J receptor in goose embryo fibroblasts; however, receptor reconstruction resulted in ALV-J infection (Mei et al., Citation2015). Taken together, these findings suggest that ALV is evolving and becoming more complicated, making it a significant challenge for poultry experts and the poultry industry in China.
At present, population purification is the most effective method to control avian leukosis. While researchers continue to develop new methods for ALV detection, enzyme-linked immunosorbent assay (ELISA)-based detection of p27 antigens from serum and cloacal swabs and meconium and albumen samples are still the most widely used methods of detection (Silva et al., Citation2007; Qian et al., Citation2015; Chen et al., Citation2018). While there are many reports showing a correlation between p27 antigen detection and positive virus isolation rates in different samples, the conclusions are not entirely consistent. The “gold standard” for ALV purification and detection is via isolation from DF-1 cells (Chai & Bates, Citation2006; Zeng et al., Citation2014; Qian et al., Citation2018). While the accuracy of this method is high, so too are the operating requirements, meaning that it is not widely used in poultry production. In this study, the prevalence of ALVs in both native and introduced geese was examined to better understand ALV infection of geese in China. Further, 10-day-old goose embryos, one-day-old geese, and goose embryo fibroblasts were artificially infected with a virulent ALV-J strain originally isolated from chickens, to determine whether a virulent ALV-J strain from a different poultry species could infect geese.
Materials and methods
Cells, viruses, and animals
The ALV-J strain used in this study (JS09GY7) was originally isolated from layer chickens with both haemangioma and myeloid leukosis (Qin et al., Citation2001). The virus was titrated in DF-1 cells to determine the median tissue culture infectious dose (TCID50). Goose embryo fibroblast (GEF) and DF-1 cells were maintained in Dulbecco’s modified Eagle’s medium (DMEM) supplemented with 5% (v/v) foetal bovine serum (FBS) and 100 U/ml of penicillin and streptomycin at 37°C in a 5% CO2 atmosphere. Chicken embryo fibroblasts (CEF) were prepared in our laboratory, as was specific monoclonal antibody targeting the p27 nucleocapsid protein of avian leukosis virus (5D3) (Khairy et al., Citation2017). Goat anti-rat IgG labelled with FITC was purchased from Sigma. The 10-day-old goose embryos and 1-day-old geese were supplied by the National Gene Bank of Waterfowl Resources (Jiangsu, China), while the ALV antigen test kit was purchased from IDEXX Laboratories, Inc. (Westbrook, ME, USA).
Sample collection and antigen detection
Between December 2017 and October 2018, 702 serum samples were collected from individual geese of the National Gene Bank of Waterfowl Resources (Jiangsu, China). Goose breeds sampled in this study included Taihu, Guangfeng Bailing, eastern Zhejiang white, Xupu, lotus white, Youjiang, and lion head. Blood samples were simultaneously collected from the 702 geese in tubes containing 4% sodium citrate anticoagulant. Serum samples were tested using IDEXX p27 antigen detection kit as per the manufacturer’s instructions, while blood samples were used for lymphocyte isolation. Information on the number of geese from each breed sampled in this study is provided in .
Table 1. ALV p27 antigen detection from serum samples and corresponding virus isolation results.
Cell culture and virus isolation
DF-1 cells were cultured in DMEM containing 5% FBS and antibiotics, and maintained in plates in an incubator at 37°C and 5% CO2. Plasma samples were prepared from the sterile goose blood samples. When the DF-1 monolayers reached 70% confluence, each well was inoculated with 60 µl of plasma suspension from the individual blood samples, and incubated at 37°C. At 2 h post-inoculation, the supernatant was discarded and the cells were washed with phosphate-buffered saline (PBS) to remove any remaining lymphocytes. A 200 µl volume of maintenance medium containing 1% FBS was then added to each well, and plates were returned to the incubator for a further 7 days. The cell monolayers were then subjected to one freeze–thaw cycle at −20 °C before the supernatants were collected and used for p27 antigen detection as per the manufacturer’s instructions.
Infection of 10-day-old goose embryos with ALV-J
Thirty 10-day-old goose embryos were randomly divided into three groups: two experimental groups and one control group (n = 10 embryos per group). Embryos from the two experimental groups were then respectively inoculated with 104 and 105 TCID50 of ALV-J strain JS09GY7 via the yolk sac, while embryos from the control group were inoculated with 0.1 ml of PBS. A total of 25 goslings were obtained from the embryos. Sterile blood samples and cloacal swab samples were collected from each of the one-day-old goslings, with the blood samples used for virus isolation and p27 antigen testing as described under “Sample collection and antigen detection”, while the cloacal swab samples were stored at −80°C. For antigen detection, the cloacal swab samples were thawed and centrifuged at 5900 x g for 5 min to precipitate any impurities. Aliquots (100 µl) of the supernatant were then used for p27 antigen detection using the IDEXX p27 antigen detection kit. Five one-day-old goslings were then randomly selected from each group and sacrificed. Livers were recovered from the birds and used for histopathological analysis and PCR-based detection of ALV-J genes.
Infection of one-day-old geese with ALV-J
Thirty one-day-old goslings were randomly divided into three groups: two experimental groups (n = 12 per group) and one control group (n = 6). Geese in the experimental groups were inoculated intraperitoneally with 104 and 105 TCID50 of ALV-J strain JS09GY7, respectively, while geese in the control group were inoculated with 0.1 ml of PBS. Sterile blood samples and cloacal swab samples were collected every 5 days, with a total of six sampling points. The collected sterile blood samples were used for virus isolation and p27 antigen detection as described under “Cell culture and virus isolation”, while the cloacal swab samples were used for p27 antigen detection as described under “Infection of 10-day-old goose embryos with ALV-J”. At 30 days post-challenge, five geese were sacrificed from each group. Livers and spleens were removed from each bird and used for histopathological analysis and PCR-based detection of ALV-J genes.
Histopathology
Livers and spleens from the two challenge experiments were fixed in 10% neutral buffered formalin. Paraffin-embedded sections were cut to a thickness of 5 μm, stained with haematoxylin and eosin, and examined for histological lesions under a 200× light microscope.
PCR analysis of liver samples
Pro-viral DNA was extracted from goose liver samples using a Universal Genomic DNA Extraction Kit (TaKaRa, Dalian, China) and used as a template for PCR detection of ALV-J. Subsequently, the DNA was re-suspended in nuclease-free water and stored at −80°C. PCR primers H5 (5ʹ-GGATGAGGTGACTAAGAAAC-3ʹ) and H7 (5ʹ-CGACCAAAGGTAACACACG-3ʹ) were used to identify ALV-J via the amplification of a 545 bp fragment corresponding to the pol to gp85 region of the ALV-J genome (Smith et al., Citation1998). Thermal cycler conditions consisted of 95°C for 5 min; 35 cycles of 95°C for 30 s, 60°C for 30 s, and 72°C for 30 s; and 72°C for 10 min. PCR primers p27-F (5ʹ-GGATGAGGTGACTAAGAAAC-3ʹ) and p27-R (5ʹ-CGACCAAAGGTAACACACG-3ʹ) were also used for identification of ALV-J, amplifying a 750 bp fragment of p27 from the viral genome (Smith et al., Citation1998). Thermal cycler parameters consisted of 95°C for 3 min; 35 cycles of 94°C for 1 min, 52°C for 1 min, and 72°C for 50 s; and 72°C for 10 min. All primers were synthesized by Sangon Biotech Co. (Shanghai, China).
ALV-J infection of GEF cells
GEF cells were cultured in DMEM containing 5% FBS and maintained at 37°C and 5% CO2 in plates. When the monolayers reached 70% confluence, 104 TCID50 of ALV-J was inoculated into each. CEF cells inoculated with the same concentration of ALV-J were used as a positive control group, while GEF monolayers inoculated with 0.1 ml of PBS served as the negative control. At 2 h post-inoculation, the culture medium was replaced with maintenance medium containing 1% FBS and the plates were incubated for a further 7 days at 37°C. The cell monolayers were then used for p27 antigen detection using the IDEXX p27 antigen testing kit (as described under “Sample collection and antigen detection”), for PCR-based detection of ALV-J gene fragments (as described under “PCR analysis of liver samples”), or for immunofluorescence assays.
Immunofluorescence assay
Replicates of the ALV-J-infected GEF cells were used for immunofluorescence assays using 5D3, a specific monoclonal antibody targeting the p27 nucleocapsid protein of ALV. Culture medium was removed from each well and the monolayers were washed twice with PBS before being fixed with an acetone: ethanol (3:2) mixture at −20°C for 10 min and then allowed to dry naturally. Monoclonal antibody 5D3 (1: 200 dilution) was added to each well and plates were incubated at 37°C for 45 min. Cells were washed three times with PBS for 5 min each time before the addition of 1:200-diluted FITC-labelled goat anti-rat IgG. Plates were incubated at 37°C for 45 min and then washed three times with PBS for 5 min each time. Finally, 50 μl of glycerol were added to each well, and the cells were observed using an inverted fluorescence microscope.
Results
Non-specific binding of ALV p27 antigen in serum samples
In this study, 702 serum samples from geese belonging to seven different breeds were screened for the presence of ALV using a p27 antigen-based ELISA assay. A total of 40 serum samples (40/702, 5.7%) across all seven breeds tested positive for the p27 antigen. To determine whether this was a true reflection of the ALV infection rate, whole-blood samples from the 702 geese were used for virus isolation and culture. Interestingly, ALV was not isolated from any of the samples (0/702, 0%). Results from the two assays are shown in .
ALV-J cannot vertically infect 10-day-old goose embryos
To determine whether geese can be infected with ALV, a vertical infection assay was carried out using a virulent ALV-J strain originally isolated from chickens. The yolk sacs of 10-day-old goose embryos were inoculated with ALV-J. Virus isolation and detection assays were then carried out on the resulting one-day-old goslings. The cloacal swab samples from all goslings were negative for p27 antigen, and no virus was isolated from any of the goose plasma samples. In addition, no obvious microscopic lesions were observed in the liver sections of geese challenged with ALV-J, with all liver tissues morphologically similar to those of the negative control (). PCR results for all liver tissues were also negative. Together, these results indicated that chicken-derived ALV-J could not be vertically transmitted to 10-day-old goose embryos.
Figure 1. Liver sections of one-day-old goslings hatched following infection with ALV-J at the 10-day-old embryo stage. Embryos were infected with (nc) PBS, (a) 104 TCID50 of ALV-J, or (b) 105 TCID50 of ALV-J. All tissues were stained with haematoxylin and eosin. Scale bar = 50 μm. Magnification, ×200.
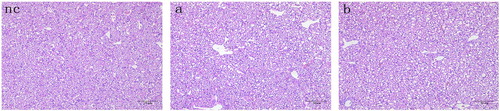
ALV-J cannot infect one-day-old goslings
To further determine whether ALV-J can infect goslings, one-day-old goslings were intraperitoneally inoculated with ALV-J. Virus isolation and detection were then conducted periodically. All resulting cloacal swab samples were negative for the p27 antigen, and ALV was not isolated from any of the plasma samples ().
Table 2. ALV p27 antigen detection from cloacal swab samples and corresponding virus isolation results.
Following ALV-J infection, the feeding habits, mental state, growth, and development of goslings were all normal. Further, histopathological analysis showed that there were no obvious microscopic lesions in the livers and spleens of infected geese, and these organs were morphologically similar to the corresponding organs from the negative controls (). PCR results were also negative for all of the collected liver tissues (). These results indicated that chicken-derived ALV-J was not infectious and had no pathogenic effects in one-day-old goslings.
Figure 2. Chicken-derived ALV-J cannot infect one-day-old goslings. (A) Liver sections from 30-day-old geese infected with ALV-J at one-day-old. (B) Spleen sections from 30-day-old geese infected with ALV-J at one-day-old. All tissues were stained with haematoxylin and eosin. Scale bar = 50 μm. Magnification, ×200. Geese were inoculated with PBS (nc), 104 TCID50 of ALV-J (a), or 105 TCID50 of ALV-J (b).
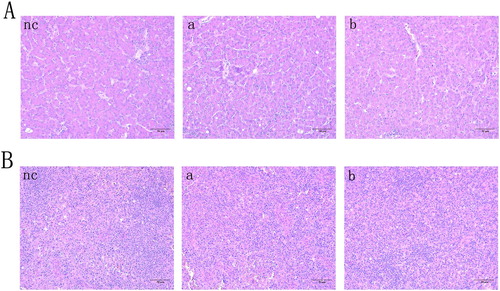
Figure 3. PCR-based detection of ALV-J genes in tissues from inoculated geese. (A) PCR-based screening for gp85 in liver tissues. (B) PCR-based screening for p27 in liver tissues. Lane M, 1 kb DNA marker; lane 1, positive control; lane 2, negative control; lane 3, tissues from geese infected with 104 TCID50 of ALV-J; lane 4, tissues from geese infected with 105 TCID50 of ALV-J
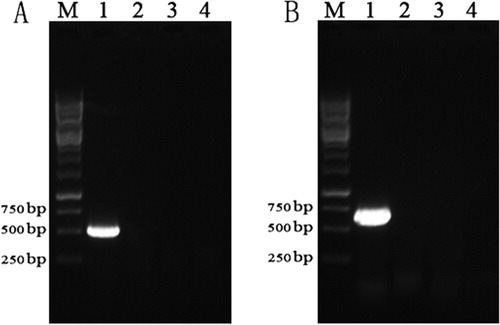
ALV-J cannot infect GEF cells
We then investigated whether the chicken-derived ALV-J strain could infect GEF cells. CEF cells were infected as a positive control, and p27 antigen detection was carried out at 7 days post-inoculation. ELISA assays were negative for all infected GEF cells, while the CEF control cells returned a positive result. PCR-based analysis of the infected cells failed to amplify a product from the GEF cells, while amplicons of the expected sizes were obtained from the control CEF cells. Following fixation of infected GEF and CEF cells, immunofluorescence assays were conducted using monoclonal antibody 5D3 and FITC-labelled goat anti-rat IgG. No fluorescence was observed in the ALV-J-infected GEF cells, while specific green fluorescence was observed in the control CEF cells (). These results suggested that chicken-derived ALV-J could not infect GEF cells.
Discussion
Avian leukosis virus is responsible for significant economic losses in poultry industries worldwide and has thus attracted much attention in both the popular and scientific press. At present, there are neither effective vaccines nor effective treatments for avian leukosis, and population purification is still the most effective treatment method. The key principle of eradication is to detect and remove any infected chicks to avoid early horizontal spread of infection. This measure relies on the detection of ALV, for which many molecular and immunological methods have been developed in recent years (Kim & Brown, Citation2004; Yun et al., Citation2013; Gao et al., Citation2014). The isolation of ALV from plasma and other samples using DF-1 cells is still recognized as the gold standard for isolating exogenous ALV (Chai & Bates, Citation2006), while ELISA-based detection of p27 antigen is the main tool for mass screening of samples at large-scale poultry farms (Silva et al., Citation2007). In this study, 702 serum samples and corresponding whole-blood samples were collected from geese belonging to seven different breeds. All serum samples were screened using an ELISA-based method targeting the p27 antigen. Positive results were obtained across all goose species, with an overall infection rate of 5.7%. However, the results of virus isolation and detection from the blood samples using DF-1 cells were negative in all cases. These findings suggested a fairly high false-positive rate from the serum detection method, which might be related to the inability of serum testing to distinguish between endogenous and exogenous viruses. Because of this non-specific detection in the poultry serum testing, we suggest that conclusions regarding ALV infection of animals should not be based solely on p27 antigen testing of serum samples.
In developed countries, the major chicken breeding companies have successfully eradicated and controlled the spread of ALV; however, in developing countries, ALV is still a significant threat (Thapa et al., Citation2004; Chang et al., Citation2013). In recent years, the host range of ALV has expanded (Shen et al., Citation2014; Zeng et al., Citation2014). In addition to the infection of broilers, layers, and local chicken breeds (Li et al., Citation2018; Meng et al., Citation2018), gene fragments corresponding to subgroup A/B and J ALVs have been amplified from wild birds (Li et al., Citation2013), while ALV-J strains have been isolated from wild ducks (Zeng et al., Citation2014). At present, ALV subgroup J strains are the most harmful among the ALVs, infecting several breeds of chickens and having an unprecedented impact on the poultry industry in China. Based on the infection pathway of ALV, we inoculated a chicken-derived ALV-J strain into the yolk sacs of 10-day-old goose embryos and into one-day-old goslings by intraperitoneal injection. Any resulting infection was detected via p27 antigen screening and virus isolation using DF-1 cells. Importantly, all samples were negative for ALV-J infection. To further screen for ALV-J infection, we attempted to amplify viral gene fragments from the samples but again obtained negative results from all samples. Additionally, no virus could be isolated from infected goose liver tissues and there were no visible lesions or histopathological changes in the infected organs. These results confirmed that the chicken-derived ALV-J strain could not replicate in goose embryos and could not infect one-day-old goslings. Finally, we carried out in vitro infection analyses of GEF cells inoculated with the chicken-derived ALV-J strain. p27 antigen detection, PCR analysis, and indirect immunofluorescence assays all showed that the GEF cells were not infected with ALV-J, further Break word as confirming if possible that the virulent ALV-J strain could not infect geese.
In developing countries, ALV infection of poultry has become more complicated. ALV-J strains have evolved rapidly, resulting in a change in host range from meat-type chickens to layer flocks and many other local chicken breeds in China. This study provides an epidemiological survey of ALV in different goose breeds, which will help to clarify the infection status of waterfowl in China and provide a reference for early prevention and control of ALV. Our results showed that, although different goose breeds tested positive for ALV using antigen-based testing, no virus was isolated. At the same time, we found that ALV-J, which is the most prevalent and harmful ALV subgroup among poultry, could not infect geese. These results suggest that, although ALV serotypes are numerous and harmful in poultry, poultry ALV strains cannot infect waterfowl, which provides important epidemiological data for the prevention and control of waterfowl leukosis and is highly significant for commercial production of waterfowl.
Ethical statement
All bird experimental infections were performed in accordance with the Regulations for the Administration of Affairs Concerning Experimental Animals, and approved by the Animal Care and Use Committee of Yangzhou University (approval ID: SYXK [Su] 2007-0005, 21 September 2016).
Disclosure statement
No potential conflict of interest was reported by the authors.
Additional information
Funding
References
- Chai, N. & Bates, P. (2006). Na+/H+ exchanger type 1 is a receptor for pathogenic subgroup J avian leukosis virus. Proceedings of the National Academy of Sciences of the United States of America, 103, 5531–5536. doi: 10.1073/pnas.0509785103
- Chang, S.W., Hsu, M.F. & Wang, C.H. (2013). Gene detection, virus isolation, and sequence analysis of avian leukosis viruses in Taiwan country chickens. Avian Diseases, 57, 172–177. doi: 10.1637/10387-092612-Reg.1
- Chen, J., Zhao, Z., Chen, Y., Zhang, J., Yan, L., Zheng, X., Liao, M. & Cao, W. (2018). Development and application of a SYBR green real-time PCR for detection of the emerging avian leukosis virus subgroup K. Poultry Science, 97, 2568–2574. doi: 10.3382/ps/pey086
- Dimcheff, D.E., Drovetski, S.V., Krishnan, M. & Mindell, D.P. (2000). Cospeciation and horizontal transmission of avian sarcoma and leukosis virus gag genes in galliform birds. Journal of Virology, 74, 3984–3995. doi: 10.1128/JVI.74.9.3984-3995.2000
- Gao, Q., Yun, B., Wang, Q., Jiang, L., Zhu, H., Gao, Y., Qin, L., Wang, Y., Qi, X., Gao, H. & Wang, X. (2014). Development and application of a multiplex PCR method for rapid differential detection of subgroup A, B, and J avian leukosis viruses. Journal of Clinical Microbiology, 52, 37–44. doi: 10.1128/JCM.02200-13
- Hao, R., Han, C., Liu, L. & Zeng, X. (2014). First finding of subgroup-E avian leukosis virus from wild ducks in China. Veterinary Microbiology, 173, 366–370. doi: 10.1016/j.vetmic.2014.08.019
- Khairy, W.O.A., Wang, L., Tian, X., Ye, J., Qian, K., Shao, H. & Qin, A. (2017). Identification of a novel linear B-cell epitope in the p27 of avian leukosis virus. Virus Research, 238, 253–257. doi: 10.1016/j.virusres.2017.07.007
- Kim, Y. & Brown, T.P. (2004). Development of quantitative competitive-reverse transcriptase-polymerase chain reaction for detection and quantitation of avian leukosis virus subgroup J. Journal of Veterinary Diagnostic Investigation, 16, 191–196. doi: 10.1177/104063870401600303
- Li, D., Qin, L., Gao, H., Yang, B., Liu, W., Qi, X., Wang, Y., Zeng, X., Liu, S., Wang, X. & Gao, Y. (2013). Avian leukosis virus subgroup A and B infection in wild birds of Northeast China. Veterinary Microbiology, 163, 257–263. doi: 10.1016/j.vetmic.2013.01.020
- Li, J., Meng, F., Li, W., Wang, Y., Chang, S., Zhao, P. & Cui, Z. (2018). Characterization of avian leukosis virus subgroup J isolated between 1999 and 2013 in China. Poultry Science, 97, 3532–3539. doi: 10.3382/ps/pey241
- McNally, M.M., Wahlin, K.J. & Canto-Soler, M.V. (2010). Endogenous expression of ASLV viral proteins in specific pathogen free chicken embryos: relevance for the developmental biology research field. BMC Developmental Biology, 10, 106. doi: 10.1186/1471-213X-10-106
- Mei, M., Ye, J., Qin, A., Wang, L., Hu, X., Qian, K. & Shao, H. (2015). Identification of novel viral receptors with cell line expressing viral receptor-binding protein. Scientific Reports, 5, 7935. doi: 10.1038/srep07935
- Meng, F., Li, Q., Zhang, Y., Zhang, Z., Tian, S., Cui, Z., Chang, S. & Zhao, P. (2018). Characterization of subgroup J avian leukosis virus isolated from Chinese indigenous chickens. Virology Journal, 15, 33. doi: 10.1186/s12985-018-0947-1
- Payne, L.N., Brown, S.R., Bumstead, N., Howes, K., Frazier, J.A. & Thouless, M.E. (1991). A novel subgroup of exogenous avian leukosis virus in chickens. Journal of General Virology, 72, 801–807. doi: 10.1099/0022-1317-72-4-801
- Payne, L.N., Gillespie, A.M. & Howes, K. (1992). Myeloid leukaemogenicity and transmission of the HPRS-103 strain of avian leukosis virus. Leukemia, 6, 1167–1176.
- Payne, L.N., Howes, K., Gillespie, A.M. & Smith, L.M. (1992). Host range of Rous sarcoma virus pseudotype RSV(HPRS-103) in 12 avian species: support for a new avian retrovirus envelope subgroup, designated J. Journal of General Virology, 73, 2995–2997. doi: 10.1099/0022-1317-73-11-2995
- Payne, L.N. & Nair, V. (2012). The long view: 40 years of avian leukosis research. Avian Pathology, 41, 11–19. doi: 10.1080/03079457.2011.646237
- Qian, K., Liang, Y.Z., Yin, L.P., Shao, H.X., Ye, J.Q. & Qin, A.J. (2015). Development and evaluation of an immunochromatographic strip for rapid detection of capsid protein antigen p27 of avian leukosis virus. Journal of Virological Methods, 221, 115–118. doi: 10.1016/j.jviromet.2015.04.033
- Qian, K., Tian, X., Shao, H., Ye, J., Yao, Y., Nair, V. & Qin, A. (2018). Identification of novel B-cell epitope in gp85 of subgroup J avian leukosis virus and its application in diagnosis of disease. BMC Veterinary Research, 14, 295. doi: 10.1186/s12917-018-1622-x
- Qin, A., Lee, L.F., Fadly, A., Hunt, H. & Cui, Z. (2001). Development and characterization of monoclonal antibodies to subgroup J avian leukosis virus. Avian Diseases, 45, 938–945. doi: 10.2307/1592872
- Shen, Y., Cai, L., Wang, Y., Wei, R., He, M., Wang, S. & Cheng, Z. (2014). Genetic mutations of avian leukosis virus subgroup J strains extended their host range. Journal of General Virology, 95, 691–699. doi: 10.1099/vir.0.059915-0
- Silva, R.F., Fadly, A.M. & Taylor, S.P. (2007). Development of a polymerase chain reaction to differentiate avian leukosis virus (ALV) subgroups: detection of an ALV contaminant in commercial Marek's disease vaccines. Avian Diseases, 51, 663–667. doi: 10.1637/0005-2086(2007)51[663:DOAPCR]2.0.CO;2
- Smith, L.M., Brown, S.R., Howes, K., McLeod, S., Arshad, S.S., Barron, G.S., Venugopal, K., McKay, J.C. & Payne, L.N. (1998). Development and application of polymerase chain reaction (PCR) tests for the detection of subgroup J avian leukosis virus. Virus Research, 54, 87–98. doi: 10.1016/S0168-1702(98)00022-7
- Sun, S. & Cui, Z. (2007). Epidemiological and pathological studies of subgroup J avian leukosis virus infections in Chinese local “yellow” chickens. Avian Pathology, 36, 221–226. doi: 10.1080/03079450701332345
- Thapa, B.R., Omar, A.R., Arshad, S.S. & Hair-Bejo, M. (2004). Detection of avian leukosis virus subgroup J in chicken flocks from Malaysia and their molecular characterization. Avian Pathology, 33, 359–363. doi: 10.1080/0307945042000220435
- Wang, C.H. & Juan, Y.W. (2002). Occurrence of subgroup J avian leukosis virus in Taiwan. Avian Pathology, 31, 435–439. doi: 10.1080/0307945021000005806
- Wang, G., Jiang, Y., Yu, L., Wang, Y., Zhao, X. & Cheng, Z. (2013). Avian leukosis virus subgroup J associated with the outbreak of erythroblastosis in chickens in China. Virology Journal, 10, 92. doi: 10.1186/1743-422X-10-92
- Weiss, R.A. & Vogt, P.K. (2011). 100 years of Rous sarcoma virus. Journal of Experimental Medicine, 208, 2351–2355. doi: 10.1084/jem.20112160
- Wu, X., Qian, K., Qin, A., Shen, H., Wang, P., Jin, W. & Eltahir, Y.M. (2010). Recombinant avian leukosis viruses of subgroup J isolated from field infected commercial layer chickens with hemangioma and myeloid leukosis possess an insertion in the E element. Veterinary Research Communications, 34, 619–632. doi: 10.1007/s11259-010-9436-8
- Yun, B., Li, D., Zhu, H., Liu, W., Qin, L., Liu, Z., Wu, G., Wang, Y., Qi, X., Gao, H., Wang, X. & Gao, Y. (2013). Development of an antigen-capture ELISA for the detection of avian leukosis virus p27 antigen. Journal of Virological Methods, 187, 278–283. doi: 10.1016/j.jviromet.2012.11.027
- Zeghdoudi, M., Aoun, L., Merdaci, L. & Bouzidi, N. (2017). Epidemiological features and pathological study of avian leukosis in turkeys’ flocks. Veterinary World, 10, 1135–1138. doi: 10.14202/vetworld.2017.1135-1138
- Zeng, X., Liu, L., Hao, R. & Han, C. (2014). Detection and molecular characterization of J subgroup avian leukosis virus in wild ducks in China. PLoS One, 9, e94980. doi: 10.1371/journal.pone.0094980
- Zhao, Z., Rao, M., Liao, M. & Cao, W. (2018). Phylogenetic analysis and pathogenicity assessment of the emerging recombinant subgroup K of avian leukosis virus in South China. Viruses, 10, 194. doi: 10.3390/v10040194