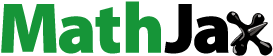
ABSTRACT
Intensification of broiler production has coincided with an increase in enteric disorders. Enteric syndromes of unknown aetiology are often associated with an increased feed conversion ratio and are given the general term “dysbiosis”. Despite the importance of dysbiosis, information on factors contributing to this condition are scarce. Therefore, the aim of this study was to describe dysbiosis in broilers (Ross 308) during one production round and to identify risk factors. Fifteen farms in Flanders (Belgium) were followed up, with visits at days 10, 17, 20, 24 and 28 of production. At every visit, 10 random birds were inspected for footpad lesions, hock burns and breast blisters. Also, coccidiosis and enteric abnormalities were scored after necropsy. A gut appearance score (GAS) was given based on 10 macroscopically visible parameters, where a higher GAS equalled more enteric abnormalities. Footpad lesions were seen in 14 farms and increased in prevalence with the age of the birds. Hock burns were seen less frequently, and no breast blisters were detected. Eimeria acervulina lesions were most frequently observed, followed by E. maxima and E. tenella lesions. The average GAS increased from day 10 until day 20. The strong correlations between the GAS at days 10, 17 and 20 indicate that prevalence of gut abnormalities at day 10 can be predictive for scores later on. A higher amount of intestinal defects was seen in older female birds, in the presence of a conceivable E. tenella infection and at farms with a higher productivity and sanitary status.
Gut lesions found in young broilers can predict further development of defects.
Caecal lesions showed the strongest correlation with GAS in a multivariate model.
RESEARCH HIGHLIGHTS
Introduction
A growing global population, rising incomes and urbanization are currently triggering an increasing demand for animal-based protein (Henchion et al., Citation2017). This demand is suitably met by broiler production, since broiler chickens are the most efficient of all terrestrial livestock in terms of the conversion of natural resources into animal products. Also, broilers have the lowest carbon footprint in meat production and no religious or cultural traditions restrict the consumption of poultry meat (Pimentel & Pimentel, Citation2003; Gerbens-Leenes et al., Citation2013; Mottet & Tempio, Citation2017).
Broiler production has undergone an exponential growth due to genetic selection and improvements in management and housing (Havenstein et al., Citation2003; Tallentire et al., Citation2016; Mottet & Tempio, Citation2017; Asche et al., Citation2018; Tickle et al., Citation2018). One drawback of this intensive production is the increased risk of transmission of different pathogens (Bailey, Citation2010). In addition, the removal of antimicrobial (AM) growth promotors (Dibner & Richards, Citation2005; Castanon, Citation2007) has further increased the incidence of enteric disorders in broilers. Furthermore, the high feed intake of modern broilers can give rise to enteritis of unknown aetiology that is characterized by intestinal inflammation and often referred to as dysbiosis (Bailey, Citation2010; Teirlynck et al., Citation2011; Collett, Citation2012; Tallentire et al., Citation2016).
It is proven that an alteration of the microbiota composition in the gut plays a role in this syndrome (Hughes et al., Citation2017). It is not always clear whether these disturbances are the cause or the consequence of intestinal disorders, but likely it goes both ways (Ducatelle et al., Citation2014; Antonissen et al., Citation2016). Also, an association with impaired intestinal barrier function and reduced nutrient digestibility has been proposed (van der Klis & Lensing, Citation2007; Ducatelle et al., Citation2018).
Undigested material will provide a substrate for specific bacterial populations to expand, while harmful bacteria can cause intestinal damage and thus affect digestibility (van der Klis & Lensing, Citation2007). Ducatelle et al. (Citation2018) referred to this condition as “the disruption of the gut microbiota composition accompanying intestinal inflammation”. The impairment of intestinal function is believed to be caused by a combination of non-infectious triggers (dietary changes, nutritional imbalance or management disorders) and infectious agents (subclinical coccidiosis or Clostridium perfringens-associated subclinical necrotic enteritis).
Dysbiosis usually affects broiler chickens at around 20–30 days of age. The main visible signs are abnormal droppings (sometimes containing undigested feed particles), an increased water intake, a decrease or stagnation in feed consumption and a concomitant increased feed conversion ratio (FCR; Wilson et al., Citation2005; Teirlynck et al., Citation2011). The elevated water intake and abnormal wet droppings can cause wet litter, which can potentially result in footpad lesions. Prolonged periods of contact with this wet litter might result in hock burn and breast blisters (Shepherd & Fairchild, Citation2010; De Jong et al., Citation2014). These types of contact dermatitis cause welfare issues in birds as it is considered to be painful (Michel et al., Citation2012). Moreover, the discomfort might lead to loss of appetite, lower slaughter weight and condemnations at the slaughter line (De Jong et al., Citation2014). Clinical signs tend to diminish at 4–5 weeks of age (Wilson et al., Citation2005; De Gussem, Citation2010; Teirlynck et al., Citation2011).
The signs of dysbiosis are not pathognomonic, so diagnosis is challenging (Bailey, Citation2010) and the syndrome is often confused with other gastrointestinal diseases (De Gussem, Citation2010; Teirlynck et al., Citation2011). Because of the difficulties in making a correct diagnosis, AM treatments are often initiated based on vague clinical signs, causing enteric problems to be the main indication for treatment with antimicrobials (Persoons et al., Citation2012; Joosten et al., Citation2019). Unfortunately, these AM treatments are strong selectors for AM resistant bacteria (WHO, Citation2012; Chantziaras et al., Citation2014; Padiyara et al., Citation2018), so intestinal problems can hinder efforts made to reduce AM treatment and, subsequently, AM resistance.
Information on the factors triggering dysbiosis is limited (M’Sadeq et al., Citation2015; Kasab-Bachi et al., Citation2017). Therefore, the aim of this study was to describe the course of dysbiosis during one production round (42 days) in broiler chickens and to identify risk factors for the onset of disease. If these risk factors can be prevented, less enteric problems will occur and AM usage may be reduced, resulting in less development of AM resistance.
Materials and methods
Study design
This study was conducted at commercial farms as the aim of the project required birds to be kept in field conditions for extrapolation of the results. Housing and animal care was in accordance to EU Directive 2007/43/EC and the Report from the Commission to the European Parliament and the Council on the application of this Directive. Birds were solely euthanized for inspection of the intestinal tract and collection of intestinal samples, and the procedure was done in accordance to Annex I to the Council Regulation (EC) No 1099/2009 of 24 September 2009.
The study included 15 commercial broiler (Ross 308) farms in Flanders (Belgium). The farms were randomly selected from a list of the clients of a specialized poultry veterinary practice (Degudap, Belgium), and the farmers were asked for their voluntary collaboration. On every farm, one broiler house was monitored during one production round. Visits for all 15 farms took place between 15th May 2017 and 1st December 2017. If the farm had more than one house, the farmer was asked to indicate the house with the history of the most severe problems related to intestinal disorders. Each farm was visited and sampled five times, on days 10, 17, 20, 24 and 28 of production, in order to cover the situation before the onset of clinical signs. After this time point we did not expect any further high increase in the amount of intestinal abnormalities. The stocking density in all houses ranged between 38 and 42 kg/m².
At day 10 of production, an interview was conducted with each farmer to obtain information regarding the intestinal problems on the farm, focussing on the occurrence of dysbiosis. In addition, information was collected on biosecurity, AM use, performance, vaccination and management. Biosecurity was evaluated on the farms by completing the Biocheck.UGent™ questionnaire. This is a risk-based scoring system that was developed to quantify biosecurity in an objective manner (Gelaude et al., Citation2014). The questionnaire for broilers (version 2.1) comprises 79 questions, subdivided into categories for external and internal biosecurity. Detailed information on the different subcategories can be found on the website (https://www.biocheck.ugent.be). Information on vaccination was collected from the registration documents of the farm veterinarian. Antimicrobial use per 100 days was quantified using the treatment incidence, as described by Persoons et al. (Citation2012), and based on the used daily dose (UDDvet). The total amount of active substance administered equals the nominator, and the denominator represents the multiplication of: (1) UDDvet (the administered dose of an active substance per kg of bird per day); (2) the number of days a bird is at risk (the number of days a bird is possibly exposed to a treatment; thus, the average lifespan of the bird); and (3) the bodyweight of the birds at risk. The latter was set at 1 kg according to ESVAC guidelines (https://www.ema.europa.eu/documents/scientific-guideline/revised-european-surveillance-veterinary-antimicrobial-consumption-esvac-reflection-paper-collecting_en.pdf). The ratio was then multiplied by 100 to obtain the TIUDDvet per 100 days. Since the administered dose of active substance per kg per day appears in both the nominator and denominator, and the kg of birds treated and the kg of birds at risk are the same (group treatment on house level), the formula can be simplified, as follows:
Performance data were collected from the technical files after completion of the production round. At all sampling times (days 10, 17, 20, 24, and 28), 10 non-clinically diseased birds were selected from evenly distributed positions along the house, without considering gender. Each bird was weighed and inspected for footpad lesions, hock burns and breast blisters. These welfare indicators were scored 0 or 1; absent or present. Whenever the epithelial layer of foot, hock or breast showed a disruption, a score of 1 was assigned. The birds were euthanized by cervical dislocation by an experienced veterinarian and the gut appearance, as well as a coccidiosis score, was obtained at necropsy as described in the following sections. The sex of the birds was also determined.
Coccidiosis scoring
Coccidiosis scoring was performed according to the method of Johnson and Reid (Citation1970) on lesions caused by different Eimeria species: E. acervulina, E. maxima and E. tenella. Scores range from 0 to 4, with 0 being the absence of lesions and 4 being the presence of the most severe lesions.
Gut appearance scoring
To determine the level of dysbiosis, the intestines were given a macroscopic gut appearance score (GAS), as described by De Gussem (Citation2010), and validated histologically by the method of Teirlynck et al. (Citation2011). Total scores range from 0, which is a normal appearance of the gastrointestinal tract, to a maximal score of 10. The scoring comprises 10 macroscopic parameters, each scored 0 when absent or 1 when present. The parameters are: (1) ballooning of the gut; (2) inflammation of the gut, visible as significant redness cranial or (3) caudal to Meckel’s diverticulum; (4) increased fragility of the gut cranial or (5) caudal to Meckel’s diverticulum; (6) abnormal tonus of the gut cranial or (7) caudal to Meckel’s diverticulum; (8) abnormal appearance of the contents in the lumen of the gut cranial or (9) caudal to Meckel’s diverticulum; and (10) undigested feed particles caudal to the ileocaecal junction.
Statistical analysis
Descriptive statistics were performed using IBM SPSS Statistics 25.0 (IBM, New York, NY, USA).
Factors contributing to a higher GAS were identified by analysing the data using a mixed proportional odds model due to the ordinal nature of the response variable. Models were fitted using the ordinal package and clmm2 function in R 3.5.1 (Christensen, Citation2018). Total GAS was set as the dependent variable, and gender, age, internal biosecurity score, external biosecurity score, total biosecurity score, footpad lesions, hock lesions, E. acervulina score, E. maxima score, E. tenella score, FCR and TIUDDvet were all evaluated as predictor variables in a univariate model. Continuous variables were scaled to have a mean of 0 and a standard deviation (SD) of 1 to prevent instability of the Hessian matrix due to differences in scale. First, an intercept only (null model) was fitted to the data. Next, the farm number was included as a random factor, taking into account the differences in baseline between farms and to correct for repeated measures in the same herd, and this was tested against the null model for significance using the likelihood ratio test. Secondly, the multivariate model was built by including all predictor variables that had a P-value of < 0.2 in the univariate analysis. The model was built in a stepwise backward procedure. The proportional odds assumption was verified visually using the Hmisc package (Harrel, Citation2018) for the final model.
Since we sampled different birds at multiple time points during one production round in the same house, we were able to evaluate the association between the GAS averages at the different time points using the Pearson correlation coefficient.
Results
Farm characteristics
The main farm characteristics are presented in . The working experience of each farmer with respect to poultry production varied from 3 to over 45 years. One-third of them worked as the only employee on the farm. Most farmers had more than one house (n = 14), and the total capacities ranged from 18,000 to 120,000 broilers. Of the 15 farmers, only four reported having few problems with intestinal disorders, whereas the other 11 reported recurring problems. Farms were visited on days 10 (9–11), 17 (15–18), 20 (20–21), 24 (24–25) and 28 (28–31) of the production round.
Table 1. To conceptualize the included farms, the main characteristics are represented here. The biosecurity level, FCR and TIUDDvet were investigated as possible risk factors for dysbiosis, as information on the factors contributing to dysbiosis are scarce.
The total score for each farm’s external biosecurity, according to the Biocheck.UGent™ questionnaire, ranged from 43% to 73%, with 100% being the maximum score. Overall, the following subcategories of external biosecurity (all measures taken to prevent the introduction of disease) scored the lowest: supply of (farm specific) materials ( = 45%) and feed and water supply (
= 50%). Most farms had adequate infrastructure and vermin control (
= 81%). For internal biosecurity, which is concerning all measures preventing the spread of diseases, the subcategory materials and measures between compartments scored the lowest (
= 45%). Disease management was already well implemented, with an average score of 80%. The FCR ranged from 1.52 to 1.73 (
= 1.60), which is in line with national averages that range from 1.54 to 1.65 (Pluimveeloket, Citation2019). One farm was able to complete an entire production round without the use of antimicrobials (TIUDDvet of 0%), while the highest user had a TIUDDvet of 38.10%, meaning that antimicrobials were administered to the birds during 38.10% of the production period. The average TIUDDvet of all farms was 16.39% (SD ± 9.34%). With a production cycle of 42 days, this means that the birds were treated with antimicrobials for almost 7 days, on average.
Nine out of 15 farms were using an anticoccidial in the feed at some time during the production round. Some used prebiotics and probiotics for prevention of intestinal disorders (sometimes in combination with an anticoccidial).
The presence of footpad lesions was seen in 14 out of 15 farms. The lesions became more prevalent with increasing age. On three farms, lesions could already be detected in 10-day-old chicks. Hock lesions were present in some cases on day 20, but most were found from day 24 onwards. On one farm, hock lesions were already present on day 17. Breast blisters were not observed during this study.
Coccidiosis score
Lesions of the different Eimeria species were scored on the five successive visits, as shown in . The E. acervulina lesion scores varied between 0 and 4, depending on the production day, whereas no scores greater than 3 were found for E. maxima. Eimeria tenella had the lowest scores on average, ranging from 0 to 2. For all Eimeria species, the severity of the lesions increased over time.
Gut appearance score
During the study, no bird had a total GAS of 8, 9 or 10. Based on all 10 parameters, the following were seen most frequently: undigested feed particles (72.9%), abnormal appearance of intestinal contents cranial (65.3%) and inflammation cranial (54.7%). By contrast, increased fragility cranial (1.1%) and caudal (1.2%) and abnormal tonus caudal (3.1%) were present in only a few cases.
During the course of the production round, the average GAS tended to increase from day 10 ( = 2.0) to day 20 (
= 3.0). After day 20, the scores tended to stabilize (
= 3.2 and 3.1 on days 24 and 28, respectively) (). However, the overall scores remained low.
Figure 2. Development of the average GAS per farm over time (n = 15). The number of lesions increased until production day 20 and then stabilized after that time point. However, the scores remained low.
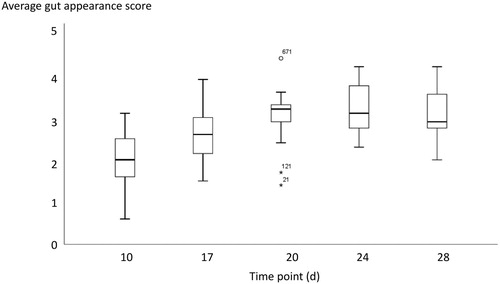
The correlation between the average GAS at different time points is presented in . A significant positive correlation was observed between the scores of days 10 and 17 and between the scores of days 10 and 20. After day 20, the strength of the correlations declined.
Table 2. Correlations between the GAS on the different days of production (days 10, 17, 20, 24 and 28), indicating that the overall score at day 10 can be predictive for scores found at days 17 and 20.
Between the average coccidiosis score (all three species together) and the GAS, a significant correlation was found on day 10 (r² = 0.385) and day 20 (r² = 0.409). These findings were mainly due to the strong significant correlation between the GAS and caecal lesions, indicative for E. tenella, at day 10 (r² = 0.617).
Proportional odds model
The output of the model is summarized in . Gender, E. tenella score, age and internal biosecurity scores were positively associated with the GAS. None of the other parameters, evaluated as predictor variables, showed a significant association with the GAS.
Table 3. Summary of the parameters of the final model. Gender, age, internal biosecurity score and Eimeria tenella score were included as fixed effects and farm number as a random factor. All four parameters appear to be possible risk factors for the onset of dysbiosis.
Macroscopic scoring system
The macroscopic GAS is used widely in practice to determine the level of dysbiosis. However, in the present study, some parameters used in the GAS were found rarely (ballooning, increased fragility cranial and caudal, and abnormal tonus caudal). The discriminating value of these parameters was assessed by removing them from the scoring system. The correlation between the original score obtained with all 10 parameters and the score with only six parameters was 0.951 (P-value < 0.001). In addition, the effect of removal of the parameter undigested feed particles, which is highly dependent on the composition and grain size of the feed, was examined. The correlation between the GAS determined based on five parameters and the GAS determined based on 10 parameters was 0.828 (P-value < 0.001).
Discussion
As enteric disorders are the main indication for AM use in broilers, knowledge about the factors contributing to these problems is important. Within this study, the presence of footpad and hock lesions increased with the age of the birds. As older birds have a higher weight and are resting more, the contact time with the litter increases. Above that, none of the welfare indicators came out of our model as a risk factor for gut abnormalities. As these indicators are a consequence of dysbiosis rather than a result, this was to be expected. The fact that we did not detect breast blisters might indicate that the litter in all participating farms was still acceptable until day 28.
The current literature indicates that enteric syndromes occur most prominently in broilers at around 3 weeks of age (Wilson et al., Citation2005; De Gussem, Citation2010; Teirlynck et al., Citation2011). Nonetheless, in the present study, we found abnormalities already present at day 10 of production. As we scored footpad and hock lesions only as being absent or present, and not differentiating lesion scores from light to severe, finding three farms where footpad lesions were already present at day 10 could be a coincidence. Above that, those farms had older houses (25, 31 and 33 years old). One house had a damaged roof, causing the ventilation to be disturbed. This could potentially influence the level of moisture in the litter, affecting the foot condition of the birds. However, no clear explanation for this observation could be found. The farm where hock lesions were already present on day 17 had the highest prevalence of hock lesions throughout the production round. An increased level of footpad lesions was found in this farm as well. This suggests that the litter quality in this farm was impaired.
The total GAS at day 10 showed a strong positive correlation with the scores at day 17 and with the scores at day 20. Although the parameters responsible for the total GAS can differ between the different time points, the total score increased over time from day 10 to 20, with a stabilization after day 20. These results suggest that gut abnormalities found at a young age can give an indication regarding the future development of lesions and, thereby, the course of enteric disorders. However, one must consider the total GAS, and not determine the presence of dysbiosis on the development of one specific parameter as dysbiosis is described by all 10 parameters.
Age, female gender, the presence of E. tenella lesions and a better internal biosecurity level can all be considered as risk factors for a high GAS, according to our model. The effect of age was expected, since enteric disorders become a greater problem later on in the production round, as was also evident in our descriptive results. This age relationship is partially in line with previous reports, where an increase in the severity of the GAS was seen until 4–5 weeks of age (Wilson et al., Citation2005; De Gussem, Citation2010; Teirlynck et al., Citation2011).
Miles et al. (Citation2006) showed that female broilers had a shorter gastrointestinal tract and shorter villi in the duodenum, which created a smaller surface area for absorption. Lumpkins et al. (Citation2008) and Zhao et al. (Citation2013) found a difference in the gut microbiota composition between male and female birds that was comparable to the results found in mice. In the latter species, a different microbiome was linked to a different immunological response. The abundance of different Lactobacillus species and E. coli in the caeca differed between sexes (Torok et al., Citation2013). The factors responsible for this gender difference are not yet understood, but it could explain a higher susceptibility of female broilers to digestive disorders and why our results showed that gender is a risk factor for a higher GAS. The higher susceptibility of female animals is not simply related to growth-rate as Lumpkins et al. (Citation2008) already found differences (at 3 days of age) in microbiota composition between males and females before differences in growth rate occurred (day 21). Future studies are needed to give better insights into these gender differences in microbiota composition and might potentially lead to the development of gender-specific diets for optimizing gut health.
During this study, only a small amount of lesions was found in the caeca, thus resulting in low E. tenella scores according to the method of Johnson and Reid. As low scores are not pathognomonic for an E. tenella infection, and lesions can be caused by different pathogens, we might have overestimated the amount of lesions caused by coccidiosis. Moreover, we did not select birds who were visually diseased. However, due to the location of the lesions and the presence of small petechiae, we believe these lesions to be somewhat typical for an E. tenella infestation.
From the proportional odds model we did find a high correlation between the lesions in the caeca and the GAS. As E. tenella is known to infect the caeca which are, to a large extent, the site where microbial fermentation takes place in chickens, with an estimated 1011 bacteria per gram in the caecal content (Barnes et al., Citation1972), damage to the caecal epithelium can allow invasion of bacteria into the mucosa, triggering a Toll-like receptor-mediated inflammatory response (Macdonald et al., Citation2017). Inflammation, in turn, will disturb the caecal microbiome, leading to a vicious cycle of inflammation and dysbiosis. Alterations in microbial composition and associated host effects taking place in the caeca may affect the entire intestine, since numerous neural and endocrine links exist between the lower and upper intestinal tract (Lynagh et al., Citation2000; Laurent et al., Citation2001; Hu et al., Citation2010).
The overall biosecurity scores found during this study were slightly below national averages as presented on the Biocheck.UGent™ website (http://www.biocheck.ugent.be/worldwide.php, visited 28/10/2019). Especially for internal biosecurity, national averages are higher than the ones derived from this study. We found the total score for all internal biosecurity parameters to have a positive association with the GAS. As this was unexpected, further research is needed to gain more information on the influence of each question of the questionnaire individually on the GAS. Also, the results depend largely on the interpretation of the farmers while taking the questionnaire, as not all measures could be checked on-site. Since farms with a better biosecurity level can have better production results (Collineau et al., Citation2017), and since faster-growing birds are more susceptible to specific intestinal pathologies, such as necrotic enteritis (Dierick et al., Citation2019), the positive association observed between the better internal biosecurity score and the GAS during this study may be confounded by the performance. Previous studies have suggested that birds raised in highly hygienic conditions can have an altered microbiota composition due to a decreased exposure to different bacteria early in life, and this can subsequently affect the normal development of the immune system (Bailey, Citation2010; Mulder et al., Citation2011; Stanley et al., Citation2013). This means that, although a high biosecurity status and good hygiene are favourable for preventing the onset of disease, these conditions may have a negative effect on the immune maturation of young birds. Further research is required to confirm this possibility.
The findings of this study should, however, be handled with the necessary precautions as we included only 15 farms. The risk factors found in our research should be confirmed by a controlled field study.
Antimicrobial therapy causes a clear alteration of the gut microbiota composition, depending on the type of AM administered (Danzeisen et al., Citation2011; Roberts et al., Citation2015; Díaz Carrasco et al., Citation2018; Kogut, Citation2018; Mehdi et al., Citation2018). Despite having a clear effect on specific bacterial pathogens, AM use can cause a change in the microbiota composition and a resulting imbalance between commensal and (opportunistic) pathogenic bacteria, potentially causing intestinal inflammation and gut pathology. In the current study, however, we could not find any association between the use of antimicrobials and the GAS. This might reflect the influence of other factors (e.g. diet, stocking density, gender or age), which cause a healthy microbial balance to be maintained.
The macroscopic GAS system is used ubiquitously in daily practice to determine the level of dysbiosis. According to De Gussem (Citation2010), the tool is efficient and has limited economic costs. However, in this study, some of the macroscopic parameters showed little variation, having only a limited effect on the results of this study. The parameter undigested feed particles is believed to be highly dependent on the composition and grain size of the feed provided. Again, the results of the study were barely influenced after removal of this parameter. These findings suggest a simplification of the current tool. Also, performing the gut appearance scoring needs experience and can be partly subjective. If the current tool could be replaced by quantification methods that use host or microbial biomarkers and that adopt easy-to-use tools on faecal material (Ducatelle et al., Citation2018; Goossens et al., Citation2018), objectivity can be enhanced. This could lead to improved AM stewardship, ultimately contributing to less development of AM resistance.
Disclosure statement
No potential conflict of interest was reported by the authors.
ORCID
Filip Van Immerseel http://orcid.org/0000-0003-0455-3350
Additional information
Funding
Notes
* Study conducted at the Veterinary Epidemiology Unit, Department of Reproduction, Obstetrics and Herd Health, Faculty of Veterinary Medicine, Ghent University, Salisburylaan 133, 9820 Merelbeke, Belgium.
References
- Antonissen, G., Eeckhaut, V., Van Driessche, K., Onrust, L., Haesebrouck, F., Ducatelle, R., Moore, R.J. & Van Immerseel, F. (2016). Microbial shifts associated with necrotic enteritis. Avian Pathology, 45, 308–312. doi: 10.1080/03079457.2016.1152625
- Asche, F., Cojocaru, A.L. & Roth, B. (2018). The development of large scale aquaculture production: a comparison of the supply chains for chicken and salmon. Aquaculture, 493, 446–455. doi: 10.1016/j.aquaculture.2016.10.031
- Bailey, R.A. (2010). Intestinal microbiota and the pathogenesis of dysbacteriosis in broiler chickens (PhD thesis). Norwich: Institute of Food Research, Norwich Research Park.
- Barnes, E.M., Mead, G.C., Barnuml, D.A. & Harry, E.G. (1972). The intestinal flora of the chicken in the period 2 to 6 weeks of age, with particular reference to the anaerobic bacteria. British Poultry Science, 13, 311–326. doi: 10.1080/00071667208415953
- Castanon, J.I.R. (2007). History of the use of antibiotic as growth promoters in European poultry feeds. Poultry Science, 86, 2466–2471. doi: 10.3382/ps.2007-00249
- Chantziaras, I., Dewulf, J., Boyen, F., Callens, B. & Butaye, P. (2014). Correlation between veterinary antimicrobial use and antimicrobial resistance in food-producing animals: a report on seven countries. Journal of Antimicrobial Chemotherapy, 69, 827–834. doi: 10.1093/jac/dkt443
- Christensen, R.H.B. (2018). Ordinal-regression models for ordinal data. R package version 2018. 4–19.
- Collett, S.R. (2012). Nutrition and wet litter problems in poultry. Animal Feed Science and Technology, 173, 65–75. doi: 10.1016/j.anifeedsci.2011.12.013
- Collineau, L., Rojo-Gimeno, C., Léger, A., Backhans, A., Loesken, S., Nielsen, E.O., Postma, M., Emanuelson, U., Beilage, E.G., Sjölund, M., Wauters, E., Stärk, K.D.C., Dewulf, J., Belloc, C. & Krebs, S. (2017). Herd-specific interventions to reduce antimicrobial usage in pig production without jeopardising technical and economic performance. Preventive Veterinary Medicine, 144, 167–178. doi: 10.1016/j.prevetmed.2017.05.023
- Danzeisen, J.L., Kim, H.B., Isaacson, R.E., Tu, Z.J. & Johnson, T.J. (2011). Modulations of the chicken cecal microbiome and metagenome in response to anticoccidial and growth promoter treatment. PLoS One, 6, 1–14. doi: 10.1371/journal.pone.0027949
- De Gussem, M. (2010). Macroscopic scoring system for bacterial enteritis in broiler chickens and turkeys. WVPA Meeting 01/04/2010.
- De Jong, I.C., Gunnink, H. & Van Harn, J. (2014). Wet litter not only induces footpad dermatitis but also reduces overall welfare, technical performance, and carcass yield in broiler chickens. Journal of Applied Poultry Research, 23, 51–58. doi: 10.3382/japr.2013-00803
- Díaz Carrasco, J.M., Redondo, E.A., Pin Viso, N.D., Redondo, L.M., Farber, M.D. & Fernández Miyakawa, M.E. (2018). Tannins and bacitracin differentially modulate gut microbiota of broiler chickens. BioMed Research International, 2018. doi: 10.1155/2018/1879168
- Dibner, J.J. & Richards, J.D. (2005). Antibiotic growth promoters in agriculture: history and mode of action. Poultry Science, 84, 634–643. doi: 10.1093/ps/84.4.634
- Dierick, E., Hirvonen, O.P., Haesebrouck, F., Ducatelle, R., Van Immerseel, F. & Goossens, E. (2019). Rapid growth predisposes broilers to necrotic enteritis. Avian Pathology, 48, 416–422. doi: 10.1080/03079457.2019.1614147
- Ducatelle, R., Eeckhaut, V., Haesebrouck, F. & Van Immerseel, F. (2014). A review on prebiotics and probiotics for the control of dysbiosis: present status and future perspectives. Animal, 9, 43–48. doi: 10.1017/S1751731114002584
- Ducatelle, R., Goossens, E., De Meyer, F., Eeckhaut, V., Antonissen, G., Haesebrouck, F. & Van Immerseel, F. (2018). Biomarkers for monitoring intestinal health in poultry: present status and future perspectives. Veterinary Research, 49. doi: 10.1186/s13567-018-0538-6
- Gelaude, P., Schlepers, M., Verlinden, M., Laanen, M. & Dewulf, J. (2014). Biocheck.UGent: a quantitative tool to measure biosecurity at broiler farms and the relationship with technical performances and antimicrobial use. Poultry Science, 93, 2740–2751. doi: 10.3382/ps.2014-04002
- Gerbens-Leenes, P.W., Mekonnen, M.M. & Hoekstra, A.Y. (2013). The water footprint of poultry, pork and beef: a comparative study in different countries and production systems. Water Resources and Industry, 1–2, 25–36. doi: 10.1016/j.wri.2013.03.001
- Goossens, E., Debyser, G., Callens, C., De Gussem, M., Dedeurwaerder, A., Devreese, B., Haesebrouck, F., Flügel, M., Pelzer, S., Thiemann, F., Ducatelle, R. & Van Immerseel, F. (2018). Elevated faecal ovotransferrin concentrations are indicative for intestinal barrier failure in broiler chickens. Veterinary Research, 49. doi: 10.1186/s13567-018-0548-4
- Harrel, F.E. (2018). Hmisc: Harrell miscellaneous. R package version 4.1–1.
- Havenstein, G.B., Ferket, P.R. & Qureshi, M.A. (2003). Growth, livability, and feed conversion of 1957 versus 2001 broilers when fed representative 1957 and 2001 broiler diets. Poultry Science, 82, 1500–1508. doi: 10.1093/ps/82.10.1500
- Henchion, M., Hayes, M., Mullen, A.M., Fenelon, M. & Tiwari, B. (2017). Future protein supply and demand: strategies and factors influencing a sustainable equilibrium. Foods, 6. doi: 10.3390/foods6070053
- Hu, X.F., Guo, Y.M., Huang, B.Y., Bun, S., Zhang, L.B., Li, J.H., Liu, D., Long, F.Y., Yang, X. & Jiao, P. (2010). The effect of glucagon-like peptide 2 injection on performance, small intestinal morphology, and nutrient transporter expression of stressed broiler chickens. Poultry Science, 89, 1967–1974. doi: 10.3382/ps.2009-00547
- Hughes, E.R., Winter, M.G., Duerkop, B.A., Spiga, L., de Carvalho, F., Zhu, T., Gillis, W., Büttner, C.C., Smoot, L., Behrendt, M.P., Cherry, C.L., Santos, S., Hooper, R.L., & Winter, L.V. & E, S. (2017). Microbial respiration and formate oxidation as metabolic signatures of inflammation-associated dysbiosis. Cell Host & Microbe, 21, 208–219. doi: 10.1016/j.chom.2017.01.005
- Johnson, J. & Reid, W.M. (1970). Anticoccidial drugs: lesion scoring techniques in battery and floor-pen experiments with chickens. Experimental Parasitology, 28, 30–36. doi: 10.1016/0014-4894(70)90063-9
- Joosten, P., Sarrazin, S., Van Gompel, L., Luiken, R.E.C., Mevius, D.J., Wagenaar, J.A., Heederik, D.J.J. & Dewulf, J. (2019). Quantitative and qualitative analysis of antimicrobial usage at farm and flock level on 181 broiler farms in nine European countries. Journal of Antimicrobial Chemotherapy, 74, 798–806. doi: 10.1093/jac/dky498
- Kasab-Bachi, H., Arruda, A.G., Roberts, T.E. & Wilson, J.B. (2017). The use of large databases to inform the development of an intestinal scoring system for the poultry industry. Preventive Veterinary Medicine, 146, 130–135. doi: 10.1016/j.prevetmed.2017.07.012
- Kogut, M.H. (2018). The effect of microbiome modulation on the intestinal health of poultry. Animal Feed Science and Technology, 250, 32–40. doi: 10.1016/j.anifeedsci.2018.10.008
- Laurent, F., Mancassola, R., Lacroix, S., Menezes, R. & Naciri, M. (2001). Analysis of chicken mucosal immune response to Eimeria tenella and Eimeria maxima infection by quantitative reverse transcription-PCR. Infection and Immunity, 69, 2527–2534. doi: 10.1128/IAI.69.4.2527-2534.2001
- Lumpkins, B.S., Batal, A.B. & Lee, M. (2008). The effect of gender on the bacterial community in the gastrointestinal tract of broilers. Poultry Science, 87, 964–967. doi: 10.3382/ps.2007-00287
- Lynagh, G.R., Bailey, M. & Kaiser, P. (2000). Interleukin-6 is produced during both murine and avian Eimeria infections. Veterinary Immunology and Immunopathology, 76, 89–102. doi: 10.1016/S0165-2427(00)00203-8
- Macdonald, S.E., Nolan, M.J., Harman, K., Boulton, K., Hume, A., Tomley, F.M., Stabler, R.A. & Blake, D.P. (2017). Effects of Eimeria tenella infection on chicken caecal microbiome diversity, exploring variation associated with severity of pathology. PLoS One, 12. doi: 10.1371/journal.pone.0184890
- Mehdi, Y., Létourneau-Montminy, M.-P., Gaucher, M.-L., Chorfi, Y., Suresh, G., Rouissi, T., Brar, S.K., Côté, C., Ramirez, A.A. & Godbout, S. (2018). Use of antibiotics in broiler production: global impacts and alternatives. Animal Nutrition, 4, 170–178. doi: 10.1016/j.aninu.2018.03.002
- Michel, V., Prampart, E., Mirabito, L., Allain, V., Arnould, C., Huonnic, D., Le Bouquin, S. & Albaric, O. (2012). Histologically-validated footpad dermatitis scoring system for use in chicken processing plants. British Poultry Science, 53, 275–281. doi: 10.1080/00071668.2012.695336
- Miles, R.D., Butcher, G.D., Henry, P.R. & Littell, R.C. (2006). Effect of antibiotic growth promoters on broiler performance, intestinal growth parameters and quantitative morphology. Poultry Science, 85, 476–485. doi: 10.1093/ps/85.3.476
- Mottet, A. & Tempio, G. (2017). Global poultry production: current state and future outlook and challenges. World’s Poultry Science Journal, 73, 245–256. doi: 10.1017/S0043933917000071
- M’Sadeq, S.A., Wu, S., Swick, R.A. & Choct, M. (2015). Towards the control of necrotic enteritis in broiler chickens with in-feed antibiotics phasing-out worldwide. Animal Nutrition, 1, 1–11. doi: 10.1016/j.aninu.2015.02.004
- Mulder, I.E., Schmidt, B., Lewis, M., Delday, M., Stokes, C.R., Bailey, M., Aminov, R.I., Gill, B.P., Pluske, J.R., Mayer, C.-D. & Kelly, D. (2011). Restricting microbial exposure in early life negates the immune benefits associated with gut colonization in environments of high microbial diversity. PLoS One, 6. doi: 10.1371/journal.pone.0028279
- Padiyara, P., Inoue, H. & Sprenger, M. (2018). Global governance mechanisms to address antimicrobial resistance. Infectious Diseases: Research and Treatment, 11, 1–4.
- Persoons, D., Dewulf, J., Smet, A., Herman, L., Heyndrickx, M., Martel, A., Catry, B., Butaye, P. & Haesebrouck, F. (2012). Antimicrobial use in Belgian broiler production. Preventive Veterinary Medicine, 105, 320–325. doi: 10.1016/j.prevetmed.2012.02.020
- Pimentel, D. & Pimentel, M. (2003). Sustainability of meat-based and plant-based diets and the environment. American Journal of Clinical Nutrition, 78, 660–663. doi: 10.1093/ajcn/78.3.660S
- Pluimveeloket. (2019). Voederconversie vleeskuikens. Retrieved from https://www.pluimveeloket.be/kenniscentrum.
- Roberts, T., Wilson, J., Guthrie, A., Cookson, K., Vancraeynest, D., Schaeffer, J., Moody, R. & Clark, S. (2015). New issues and science in broiler chicken intestinal health: intestinal microbial composition, shifts, and impacts. World’s Poultry Science Journal, 71, 259–270. doi: 10.1017/S0043933915000276
- Shepherd, E.M. & Fairchild, B.D. (2010). Footpad dermatitis in poultry. Poultry Science, 89, 2043–2051. doi: 10.3382/ps.2010-00770
- Stanley, D., Geier, M.S., Hughes, R.J., Denman, S.E. & Moore, R.J. (2013). Highly variable microbiota development in the chicken gastrointestinal tract. PLoS One, 8. doi: 10.1371/journal.pone.0084290
- Tallentire, C.W., Leinonen, I. & Kyriazakis, I. (2016). Breeding for efficiency in the broiler chicken: a review. Agronomy for Sustainable Development, 36. doi: 10.1007/s13593-016-0398-2
- Teirlynck, E., De Gussem, M., Dewulf, J., Haesebrouck, F. & Ducatelle, R. (2011). Morphometric evaluation of “dysbacteriosis” in broilers. Avian Pathology, 40, 139–144. doi: 10.1080/03079457.2010.543414
- Tickle, P.G., Hutchinson, J.R. & Codd, J.R. (2018). Energy allocation and behaviour in the growing broiler chicken. Scientific Reports, 8. doi: 10.1038/s41598-018-22604-2
- Torok, V.A., Dyson, C., Mckay, A. & Ophel-Keller, K. (2013). Quantitative molecular assays for evaluating changes in broiler gut microbiota linked with diet and performance. Animal Production Science, 53, 1260–1268. doi: 10.1071/AN12272
- van der Klis, J. D. & Lensing, M. (2007). Wet litter problems relate to host-microbiota interactions. World Poultry, 23, 20–22.
- WHO. (2012). The evolving threat of antimicrobial resistance: options for action. World Health Organization.
- Wilson, J., Tice, G., Brash, M.L. & Hilaire, S.S. (2005). Manifestations of Clostridium perfringens and related bacterial enteritides in broiler chicken. World’s Poultry Science Journal, 61, 435–449. doi: 10.1079/WPS200566
- Zhao, L., Wang, G., Siegel, P., He, C., Wang, H., Zhao, W., Zhai, Z., Tian, F., Zhao, J., Zhang, H., Sun, Z., Chen, W., Zhang, Y. & Meng, H. (2013). Quantitative genetic background of the host influences gut microbiomes in chickens. Scientific Reports, 3. doi: 10.1038/srep01163