ABSTRACT
This paper describes the characterization of a new infectious bronchitis virus (IBV) strain D181, that rapidly evolved from a low-level incidental finding in 2017 to become the second most isolated IBV strain in Dutch layers and breeders in 2018, as well as being found in samples from Germany and Belgium. Based on the sequence of the S gene and the results of cross-neutralization tests, D181 can be considered as a new serotype and the second lineage within genotype II (GII-2). The experimental infection of SPF hens confirmed the ability of D181 to cause a drop in egg production, and immunohistochemistry showed presence of the virus in the trachea, lung and conjunctiva at 5 days post inoculation and in the caecal tonsils at 5 and 8 days post inoculation. In silico analysis of several widely used PCR primers indicated that primer sets adapted for GII might be needed to detect D181, as many general S1 primers might miss it.
Introduction
Infectious bronchitis virus (IBV), a highly contagious and rapidly spreading gammacoronavirus, continues to have a high impact on poultry production world-wide (De Wit, Cook et al., Citation2011). Like other single-stranded RNA viruses, IBV has a high rate of acquiring genetic mutations, leading to new viral strains with potentially modified antigenicity or enhanced pathogenicity, potentially posing challenges for the preventive immunization of poultry flocks (Jackwood et al., Citation2005; De Wit et al., Citation2018; Marandino et al., Citation2019). Because developing a new vaccine for each emerging strain of IBV is unfeasible, cross-protection conferred by existing vaccines should always be evaluated.
This paper describes the characterization of a new IBV strain named D181, that rapidly evolved from a low-level incidental finding in 2017 to become the second most isolated IBV strain in Dutch layers and breeders in 2018, as well as being found in samples from Germany and Belgium (Rapportage monitoring diergezondheid Pluimvee, Citation2018). The rapid emergence of this strain suggests an antigenic advantage compared to earlier strains as the affected layer and breeder flocks had all been primed using live vaccines of the Massachusetts, 793B and QX serotypes and boosted with an inactivated vaccine with at least an M41 antigen. For this reason, virus cross-neutralization tests were performed using strains and antisera of seven serotypes, plus the D181 strain and its antiserum, to determine the antigenic relationship of D181 with other IBV strains. We further report on the results of sequencing the S gene (S1 part), and the clinical signs including the drops in egg production observed both after experimental infection in laying SPF hens as well as in field cases.
Materials and methods
Survey of affected farms
In 2018 GD Animal Health detected IBV D181 in submitted samples (21×) and chickens (27×) of 30 layer farms, 16 breeder farms and two broiler farms. Owners of each of the 27 farms from which IBV D181-positive chickens had been sent in for post mortem examination to GD Animal Health were contacted and asked to partake in a survey. The 14 owners who agreed were asked for details of the clinical signs in the period leading up to and following the post mortem submission, including changes in mortality, egg production, eggshell abnormalities, respiratory signs and intake of feed or water.
RT–PCR and partial S1 sequencing
For routine testing, viral RNA was extracted using the High Pure Viral Nucleic Acid kit (Roche Applied Science, Penzberg, Germany) according to the manufacturer’s instructions. Two RT–PCR primer sets were used, a universal IBV RT–PCR generating a fragment of about 350 base pairs of the S1 gene with primers XCE1+ and XCE3– (Cavanagh et al., Citation1999), and a genotype-specific RT–PCR for D1466-like strains using forward primer 5′-TACRggMAATTTTACTgATgg-3′ and reverse primer 5′-CTgACTgCTTACAAgAACC-3′. Both RT–PCRs were performed on a VeritiTM Thermal Cycler (Thermo Fisher Scientific, Waltham, MA, USA) using the Qiagen one-step RT–PCR kit (Qiagen, Hilden, Germany) under the following conditions: reverse transcription reaction for 30 min at 50°C; denaturation 15 min at 95°C, followed by 45 cycles with 30 s at 95°C, 30 s at 50°C and 60 s at 72°C. The S1 amplicons were separated on a 1% agarose gel, and visualized with ethidium bromide staining and an ultraviolet light transilluminator. The purified amplicons were sequenced (BaseClear, Leiden, The Netherlands) bidirectionally using Sanger sequencing with both the XC1+ and XCE3–primers. Consensus sequences were constructed using MEGA7.0 (Kumar et al., Citation2016)
Sequencing of S gene of strain IBV D181
The complete S gene of strain D181 was sequenced and assembled from paired-end libraries using Illumina HiSeq 2000 sequencing (Illumina, Inc., San Diego, CA, USA) at BaseClear (Leiden, The Netherlands). For de novo assembly, reads below 150 bases were discarded, paired-end reads with a minimum distance of 50 bases and a maximum distance of 500 bases were included and a minimum contig length of 200 bases was used as a threshold. Scaffolds were produced from resulting contigs using the CLC Genomics Workbench, and the NCBI ORF finder tool was used to search for open reading frames and potential protein encoding segments. The sequence encoding for the spike protein was obtained using the SMART BLAST option. The S1 sequence of strain D181 was compared with a selection of the S1 sequences previously used by Valastro et al. (Citation2016) for the S1-gene-based phylogeny of IBV. Phylogenetic analysis with 100 bootstrap iterations was used to demonstrate phylogenetic relatedness of strain D181 to these known genotypes. The evolutionary history was inferred by using the Maximum Likelihood method based on the Tamura-Nei model (Tamura & Nei, Citation1993). The tree with the highest log-likelihood (−48746.42) is shown. Initial tree(s) for the heuristic search were obtained automatically by applying Neighbor-Join and BioNJ algorithms to a matrix of pairwise distances estimated using the Maximum Composite Likelihood approach, and then selecting the topology with superior log-likelihood value. The tree is drawn to scale, with branch lengths measured in the number of substitutions per site. The analysis involved 70 nucleotide sequences. Codon positions included were 1st + 2nd + 3rd + noncoding. All positions with less than 95% site coverage were eliminated. That is, fewer than 5% alignment gaps, missing data, and ambiguous bases were allowed at any position. There was a total of 1542 positions in the final dataset. Evolutionary analyses were conducted in MEGA7 (Kumar et al., Citation2016).
In silico analysis of PCR primers used for detection of D181
The D181 S1 sequence was used for in silico analysis of 10 IBV universal forward primers and 12 universal reverse primers that are widely used for the detection of the S1 of IBV (Adzhar et al., Citation1997; Keeler et al., Citation1998; Cavanagh et al., Citation1999; Farsang et al., Citation2002; Bochkov et al., Citation2007; Worthington et al., Citation2008). In addition, five D1466 genotype-specific forward primers and five D1466 genotype-specific reverse primers (Adzhar et al., Citation1997; Cavanagh et al., Citation1999; Jones et al., Citation2011; Domanska-Blicharz et al., Citation2017) were included in this comparison. Primer sequences were aligned with the nucleotide position of the D181 full S1 sequence; the number of mismatches per primer, mismatch at the 3′-end of the primer and mismatches in the last three nucleotides at the 3′-end were determined. Primers without a mismatch at the final 3′-end nucleotide and containing a maximum of three mismatches were considered capable of amplifying D181. Primers with a maximum of three mismatches, of which one was located at the second- or third-last nucleotide at the 3′-end, were considered able to amplify D181, but possibly with lower efficiency.
Virus cross-neutralization tests
Virus cross-neutralization tests were performed using strain D181 and strains of serotypes Mass, D1466, 793B, D388/QX, Arkansas, Var2 and Q1 and their monospecific antisera. The sera had been raised in groups of 4–8-week-old SPF White Leghorn chickens (GD Animal Health) except for the D181 serum that had been raised in the SPF layer chickens of the infection study described below. The antisera were pooled per virus and used in duplicate to determine the ability to neutralize a constant amount of 30–300 median tissue culture infectious doses (TCID50) of virus of each of the eight serotypes. The virus was mixed with two-fold dilutions of antisera and incubated for 1 h at 36–38°C. Subsequently, the virus-serum mixtures were transferred in duplicate to wells of microtitre plates which contained monolayers of primary chicken embryo kidney cells. The plates were placed in a CO2 incubator at 36–38°C for 72 h. The VN titres were expressed as the reciprocal of the highest dilution of serum that prevented cytopathic effects. The test was performed in the presence of appropriate controls.
Experimental infection study
Following a rapid increase in the number of IBV D181-positive layer and breeder flocks with production problems in 2018, an infection trial was conducted to assess the ability of this new strain to induce a production drop as well as to acquire a monospecific serum for virus neutralization testing. Thirty-eight SPF white layer hens of 19 weeks of age were taken from a SPF egg-production flock (GD Animal Health) and housed in two isolators. Food and water were given ad libitum and birds were checked daily for signs of disease and the number of eggs produced. At 26 weeks of age, the hens were at the peak of lay and were inoculated intratracheally with 0.5 ml diluent containing 106 EID50 of IBV D181 isolate CK/NL/D181/2018 and observed daily until the end of the experiment at 70 days post inoculation (dpi). At 5 dpi and at 8 dpi two hens per isolator were euthanized for the collection of organ samples in buffered 10% formalin, and collection of trachea for the ciliostasis test. Blood was collected from five hens per isolator at the day of inoculation as well as from all the remaining birds at the end of the experiment at 70 dpi.
Ciliostasis test
The level of damage to the trachea was determined using the ciliostasis test on five tracheal rings per bird. The tracheas were placed in Hank’s Minimum Essential Medium immediately after euthanizing the chickens by an intravenous injection of 0.1–0.2 ml Euthasol 20% (AST farma, Oudewater, The Netherlands) and subsequent bleeding. Five tracheal rings (equally divided over the total length of the trachea) were cut and placed in medium at 37°C. The level of ciliostasis was determined independently by two technicians between 1 and 4 h after the birds were killed. The level of beating of the cilia in each ring was expressed as 4 (<25% beating of cilia), 3 (25–50% beating), 2 (50–75% beating), 1 (75–99% beating) or 0 (all beating) (De Wit et al., Citation2013). One bird could score between 0 and 20 (five rings from each trachea; maximum score 4).
Immunohistochemistry
The presence of IBV antigen in trachea, lung, kidneys, proventriculus, conjunctiva, duodenum, jejunum, caecal tonsil and the shell gland, magnum and infundibulum of the oviduct was investigated using immunohistochemistry (IHC) (De Wit, Nieuwenhuisen-van Wilgen et al., Citation2011). After fixation for at least 24 h in buffered 10% formalin followed by dehydration in absolute ethanol and embedding in paraffin wax, sections were cut at 4 µm and mounted on glass slides. Endogenous peroxidase activity was blocked by incubation with 1% H2O2 containing 0.1% NaN3 for 20 min at room temperature, and subsequently boiled in Tris (0.01 M) EDTA (0.001 M), pH 9.0 for 10 min. The binding of Fc-receptors was blocked by incubation with 10% foetal bovine serum for 20 min at room temperature. The immunostaining of IBV-positive cells was performed using 1:20,000 diluted anti-IBV nucleoprotein monoclonal antibody 48.4 (Prionics, Lelystad, The Netherlands) in Normal Antibody Diluent (Klinipath, Duiven, The Netherlands) for 30 min at room temperature. After three subsequent wash steps with phosphate-buffered saline, the sections were treated with anti-mouse Dako EnVision+ (Dako UK Ltd, Cambridgeshire, UK) for 30 min at room temperature. Again, sections were washed three times with phosphate-buffered saline and then treated with DAB+ (Dako UK Ltd) for 5 min at room temperature. Finally, the sections were counter-stained using haematoxylin. Sections incubated in the absence of primary antibody were taken as negative controls.
Results
Survey of affected farms
Included in the survey were 13 farms with egg-laying chickens and one broiler farm. The results of the post mortem examinations of these cases indicated that IBV D181 was the main finding, although avian metapneumovirus (1×), IBV QX-D388 (1×) and IBV 793B (4×, vaccine-like) and Escherichia coli (6×) were detected in several of these flocks as well. Increased mortality was reported in 12 of the 14 farms, including the broiler farm. None of the participants could properly quantify the amount of losses directly associated with the D181 infection, because they had problems identifying when mortality due to the D181 infection started, ended and what should be considered “normal mortality” for their farm. There did not appear to be a sharp increase and subsequent decrease in mortality in the affected flocks, as opposed to what was reported for the egg production. A decrease in egg production was reported in 10 out of 13 farms with layers or broiler breeders. There was a drop typically between 5% and 7% with a single farm reporting 15% loss of production. Only four out of 10 flocks returned to a normal level of egg production afterwards. This happened at 4, 5, 8 or 14 weeks after the start of the drop. Eggshell abnormalities were seen in five of the 10 flocks with production drops but not in any of the flocks without loss of production. In four out of these five flocks there was loss of colour of the eggshell and in three flocks the egg packing station noted a decrease in eggshell strength during routine measurements. In two flocks there was an increased incidence of misshapen eggs. Respiratory signs were only reported in one of the 14 flocks, and this flock subsequently experienced a drop in egg production. Feed intake was lowered in five egg-laying farms and in the broiler farm. No effect on water intake was reported.
RT–PCR and S sequencing
Sequence analysis of the partial S1 gene demonstrated that the strain was a D1466 -like strain and was named CK/NL/D181/2018 or D181. The partial S1 sequence has been deposited in GenBank (acc. no. MH325904). Illumina sequencing and de novo assembly resulted in the full S sequence with very high coverage (3000×). The full S sequence of the D181 strain has been deposited in GenBank (acc.no. MK840961). Maximum likelihood analysis of strain D181 and known IBV genotypes demonstrates that, also based on the full S1 gene, strain D181 is most related to strain D1466 (GII-1) showing 90% sequence similarity (). The mutations making up the remaining 10% were divided over the complete S1 gene, the recombination analysis showed no indication that the S1 gene of D181 was the result of a recombination of a D1466 strain with another IBV strain.
In silico analysis of PCR primers used for detection of D181
Based on sequence comparisons, IBV universal primers “XCE2-” and “D7-” (Adzhar et al., Citation1997; Cavanagh et al., Citation1999), “S5” and “S9” (Bochkov et al., Citation2007) and “CK2” and “CK4” (Keeler et al., Citation1998) gave an almost perfect match with the S1 sequence of D181 (supplementary table). All D1466 genotype-specific primers also gave a very good match with the sequence of D181. The remaining eight IBV universal forward primers and eight universal IBV reverse primers contained either more than three mismatches in total or a mismatch at the final 3′-end nucleotide, and were therefore considered less suitable for efficient detection of strain D181. These include the following primers “XCE 1+”, “XCE 3–”, “S27–”, “S3”, “S4”, “S6”, “S7”, “S8”, “S11”, “S12”, “ÇO1”, “ÇO2”, “SX1”, “SX3”, “SX2” and “SX4” as detailed in the supplementary table.
Virus cross-neutralization tests
The inoculation of the SPF birds with D181 resulted in a high level of neutralizing antibodies against this strain (). The results of the two-way cross-neutralization tests using strains D181, D1466, M41, D388, 793B-4/91, Arkansas, Var2 and Q1 and their antisera showed that the strains were all of different serotypes (). Although the cross-neutralization of the D181 and D1466 antigens and antisera showed they were antigenically different, the level of cross-neutralization showed that they were antigenically more related than with the other tested serotypes of IBV. The level of antigenic relationship between the D188 and D1466 strain was determined using the formula of Archetti and Horsfall (Citation1950). The r-value was 1/11.4, meaning a 9% antigenic cross-relationship between the two strains. D181 can therefore be considered a new serotype of IBV GII (Valastro et al., Citation2016).
Table 1. Results of virus cross-neutralization tests using eight different IBV variants and their antisera.
Experimental infection study
Clinical signs
Respiratory signs were noted in two hens at 1 dpi, in one hen at 3 dpi and in one hen at 4 dpi. These signs consisted of laboured respiration and, at 1 dpi, one of the two affected hens was seen coughing. No other clinical findings were recorded for the rest of the experiment, either before or after inoculation.
Egg production
Egg production was analysed based on 5-day averages. Production started normally but showed a dip from an 83% average at 1–5 dpi to an average of 73% at 6–10 dpi. The decreased egg production persisted for about 8 weeks. At 61–65 dpi, egg production was recovered and remained stable during the last 10 days of the experiment.
Ciliostasis test
The level of damage to the cilia beating showed considerable variation between the birds (). As expected, the level of tracheal damage was higher at 5 dpi than at 8 dpi (De Wit & Cook, Citation2014). At 5 dpi two hens scored 20 (no cilia beating anymore), one hen scored 8 and one hen scored 0 (all cilia beating). At 8 dpi two hens scored 5 and two hens scored 0 (all cilia beating).
Table 2. Results of the ciliostasis test and immunohistochemical staining for IBV in various organs at 5 and 8 days post inoculation (dpi) with IBV strain D181.
Immunohistochemistry
At 5 dpi all four hens had positive staining in both tracheal epithelial cells ((a)) and caecal tonsils ((b)), where immunostaining was mostly confined to epithelial cells lining the luminal side of the tonsil as well as cells directly subepithelial, although occasionally a few unidentified cells scattered within the caecal tonsil were also positive. Two hens also had immunopositive staining in the lung, which was limited to the epithelial cells lining the primary bronchi. Another hen also had positive staining in the conjunctiva. No positive staining was seen in any sample from proventriculus, duodenum, jejunum, kidney or shell gland, magnum or isthmus of the oviduct.
Figure 2. (a) Trachea of a hen at 8 days post inoculation with IBV D181, immunostained with monoclonal anti-IBV-nucleoprotein antibody, showing strong immune-reactivity mainly in epithelial cells. (b) Caecal tonsil of a hen at 8 days post inoculation with IBV D181, immunostained with monoclonal anti-IBV-nucleoprotein antibody, showing strong immune-reactivity mainly in epithelial cells.
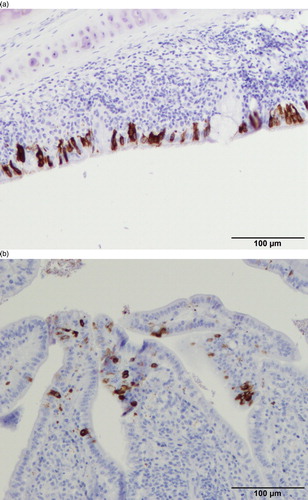
At 8 dpi almost all tissues were negative and only the caecal tonsils of two out of four hens showed immunostaining ().
Discussion
This is the first report of D181, a new serotype of IBV that is now frequently isolated from Dutch poultry with several findings in German and Belgian layer and breeder flocks as well. Based on the sequence of the S gene, D181 is most closely related to genotype II lineage 1 strain D1466 (GII-1) (Valastro et al., Citation2016) showing 90% sequence similarity. The D1466 variant (also called D212) was detected for the first time in The Netherlands in the late 1970s, when it was recognized to have antigenic and molecular properties significantly different from known IBV strains (Davelaar et al., Citation1984). Based on the distribution of mutations in S1, D181 seems to have evolved from D1466 by drift. These mutations have resulted in distinctive antigenic differences as shown by the cross-neutralization test results where only 9% cross-relationship between these strains was found. This means that D181 can be considered as a new serotype and the second lineage within genotype II (GII-2) (Valastro et al., Citation2016). To the knowledge of the authors, until now, D181 has only been detected in The Netherlands, Belgium and Germany. The lack of reports from other countries might be due to a limited geographic distribution, but under-diagnosis cannot be ruled out, since in silico evaluation of 10 forward and 12 reverse primers commonly used in diagnostic general IBV PCR tests indicated that 16 of these 22 might be unsuitable to detect D181 strains.
Detection of IBV D181 in Dutch poultry was associated with increased mortality and, in 10 out of 13 egg-laying flocks with decreased egg production, typically around 5–7%, but without respiratory signs. The experimental infection of SPF layers confirmed the ability of D181 to cause a drop in egg production. Similar to the field cases, this drop in egg production was long-lasting. There was neither mortality nor noticeable respiratory signs. Experimental IBV infections may lead to fewer clinical signs compared to field outbreaks because co-infections with potential synergistic effects are absent (van Empel et al., Citation1996; Dwars et al., Citation2009; Feberwee & Landman, Citation2010; De Wit & Cook, Citation2014). However, the absence of noticeable respiratory signs was in line with the rather mild and variable tracheal damage at 5 dpi, when the average level of ciliostasis was 60%. These results might indicate that the strain is not very pathogenic for the tracheal epithelium, although only a limited number of birds were tested for the level of ciliostasis. If more work confirms these findings in the respiratory tract, it might explain why D181 was mostly detected in egg-laying flocks and only rarely in broilers. Interestingly, this is also the case for the genetically related D1466 strain (Davelaar et al., Citation1984; Worthington et al., Citation2008; Domanska-Blicharz et al., Citation2012). More work is needed to understand why and to what extent both genotype II lineages show this behaviour.
Despite the moderate respiratory damage and the limited number of IHC positive organs in the birds tested at 5 and 8 dpi, the rapid emergence of this strain in layers and breeders that had been vaccinated using live vaccines of the Massachusetts, 793B and QX serotypes and boosted with an inactivated vaccine with at least a M41 antigen, suggests an antigenic advantage for this new field strain compared to earlier strains. Based on the results of the cross-neutralization tests, a single vaccination using a heterologous vaccine of the IBV serotypes tested is not expected to induce protection against D181 infection (Cook et al., Citation1999; De Wit et al., Citation2013). However, this remains to be tested, as does the possible benefit of combinations of IBV vaccines of different serotypes.
In summary, D181 can be considered as a new second lineage and serotype of GII of IBV for which adapted primer sets are recommended in order to be able to detect it with good sensitivity. Many general primers have multiple nucleotide mismatches, potentially resulting in reduced PCR efficiency, lower sensitivity or even failure in detecting this strain. D181 is mostly detected in laying birds with a drop in egg production and increased mortality, and this drop in egg production could be reproduced in an experimental infection of SPF birds. Intensity and organ distribution of immunostaining further suggests that tracheal swabs would be a suitable sample to detect early infections, while cloacal swabs might be better for detecting older infections as has been described for other IBV strains (De Wit, Citation2000).
Supplemental Material
Download MS Word (42.1 KB)Disclosure statement
No potential conflict of interest was reported by the authors.
ORCID
R. J. Molenaar http://orcid.org/0000-0003-0336-1226
J. J. de Wit http://orcid.org/0000-0002-3459-1000
Additional information
Funding
References
- Adzhar, A., Gough, R.E., Haydon, D., Shaw, K., Britton, P. & Cavanagh, D. (1997). Molecular analysis of the 793/B serotype of infectious bronchitis virus in Great Britain. Avian Pathology, 26, 625–640. doi: 10.1080/03079459708419239
- Archetti, I. & Horsfall, F.L., Jr. (1950). Persistent antigenic variation of influenza A viruses after incomplete neutralization in ovo with heterologous immune serum. Journal of Experimental Medicine, 92, 441–462. doi: 10.1084/jem.92.5.441
- Bochkov, Y.A., Tosi, G., Massi, P. & Drygin, V.V. (2007). Phylogenetic analysis of partial S1 and N gene sequences of infectious bronchitis virus isolates from Italy revealed genetic diversity and recombination. Virus Genes, 35, 65–71. doi: 10.1007/s11262-006-0037-0
- Cavanagh, D., Mawditt, K., Britton, P. & Naylor, C.J. (1999). Longitudinal field studies of infectious bronchitis virus and avian pneumovirus in broilers using type-specific polymerase chain reactions. Avian Pathology, 28, 593–605. doi: 10.1080/03079459994399
- Cook, J.K.A., Orbell, S.J., Woods, M.A. & Huggins, M.B. (1999). Breadth of protection of the respiratory tract provided by different live-attenuated infectious bronchitis vaccines against challenge with infectious bronchitis viruses of heterologous serotypes. Avian Pathology, 28, 477–485. doi: 10.1080/03079459994506
- Davelaar, F.G., Kouwenhoven, B. & Burger, A.G. (1984). Occurrence and significance of infectious bronchitis virus variant strains in egg and broiler production in the Netherlands. Veterinary Quarterly, 6, 114–120. doi: 10.1080/01652176.1984.9693924
- De Wit, J.J. (2000). Detection of infectious bronchitis virus. Avian Pathology, 29, 71–93. doi: 10.1080/03079450094108
- De Wit, J.J., Boelm, G.J., van Gerwe, T.J.W.M. & Swart, W.A.J.M. (2013). The required sample size in vaccination-challenge experiments with infectious bronchitis virus, a meta-analysis. Avian Pathology, 42, 9–16. doi: 10.1080/03079457.2012.751485
- De Wit, J.J., Cazaban, C., Dijkman, R., Ramon, G. & Gardin, Y. (2018). Detection of different genotypes of infectious bronchitis virus and of infectious bursal disease virus in European broilers during an epidemiological study in 2013 and the consequences for the diagnostic approach. Avian Pathology, 47, 140–151. doi: 10.1080/03079457.2017.1387231
- De Wit, J.J. & Cook, J.K.A. (2014). Factors influencing the outcome of infectious bronchitis vaccination and challenge experiments. Avian Pathology, 43, 485–497. doi: 10.1080/03079457.2014.974504
- De Wit, J.J., Cook, J.K.A. & van der Heijden, H.M.J.F. (2011). Infectious bronchitis virus variants: a review of the history, current situation and control measures. Avian Pathology, 40, 223–235. doi: 10.1080/03079457.2011.566260
- De Wit, J.J., Nieuwenhuisen-van Wilgen, J., Hoogkamer, A., Van de Sande, H., Zuidam, G.J. & Fabri, T.H.F. (2011). Induction of cystic oviducts and protection against early challenge with infectious bronchitis virus serotype D388 (genotype QX) by maternally derived antibodies and by early vaccination. Avian Pathology, 40, 463–471. doi: 10.1080/03079457.2011.599060
- Domanska-Blicharz, K., Lisowska, A., Jatczak, J., Mamczur, J. & Minta, Z. (2012). D1466-like genotype of infectious bronchitis virus responsible for a new epidemic in chickens in Poland. Veterinary Record, 171, 351. doi: 10.1136/vr.100888
- Domanska-Blicharz, K., Lisowska, A., Pikuła, A. & Sajewicz-Krukowska, J. (2017). Specific detection of GII-1 lineage of infectious bronchitis virus. Letters in Applied Microbiology, 65, 141–146. doi: 10.1111/lam.12753
- Dwars, R.M., Matthijs, M.G., Daemen, A.J., van Eck, J.H., Vervelde, L. & Landman, W.J. (2009). Progression of lesions in the respiratory tract of broilers after single infection with Escherichia coli compared to superinfection with E. coli after infection with infectious bronchitis virus. Veterinary Immunology and Immunopathology, 127, 65–76. doi: 10.1016/j.vetimm.2008.09.019
- Farsang, A., Ros, C., Renstrom, L.H., Baule, C., Soos, T. & Belak, S. (2002). Molecular epizootiology of infectious bronchitis virus in Sweden indicating the involvement of a vaccine strain. Avian Pathology, 31, 229–236. doi: 10.1080/03079450220136530
- Feberwee, A. & Landman, W.J. (2010). Induction of eggshell apex abnormalities in broiler breeder hens. Avian Pathology, 39, 133–137. doi: 10.1080/03079451003657637
- Jackwood, M.W., Hilt, D.A., Lee, C.W., Kwon, H.M., Callison, S.A., Moore, K.M., Moscoso, H., Sellers, H. & Thayer, S. (2005). Data from 11 years of molecular typing infectious bronchitis virus field isolates. Avian Diseases, 49, 614–618. doi: 10.1637/7389-052905R.1
- Jones, R.M., Ellis, R.J., Cox, W.J., Errington, J., Fuller, C., Irvine, R.M. & Wakeley, P.R. (2011). Development and validation of RT-PCR tests for the detection and S1 genotyping of infectious bronchitis virus and other closely related gammacoronaviruses within clinical samples. Transboundary Emerging Diseases, 58, 411–420. doi: 10.1111/j.1865-1682.2011.01222.x
- Keeler, C.L., Jr., Reed, K.L., Nix, W.A. & Gelb, J., Jr. (1998). Serotype identification of avian infectious bronchitis virus by RT-PCR of the peplomer (S-1) gene. Avian Diseases, 42, 275–284. doi: 10.2307/1592477
- Kumar, S., Stecher, G. & Tamura, K. (2016). MEGA7: Molecular Evolutionary Genetics Analysis version 7.0 for bigger datasets. Molecular Biology and Evolution, 33, 1870–1874. doi: 10.1093/molbev/msw054
- Marandino, A., Vagnozzi, A., Craig, M.I., Tomas, G., Techera, C., Panzera, Y., Vera, F. & Perez, R. (2019). Genetic and antigenic heterogeneity of infectious bronchitis virus in South America: implications for control programmes. Avian Pathology, 48, 270–277. doi: 10.1080/03079457.2019.1583315
- Rapportage monitoring diergezondheid Pluimvee. (2018). Retrieved from https://www.gddiergezondheid.nl/diergezondheid/monitoring/hoofdpunten-monitoring-pluimvee
- Tamura, K. & Nei, M. (1993). Estimation of the number of nucleotide substitutions in the control region of mitochondrial DNA in humans and chimpanzees. Molecular Biology and Evolution, 10, 512–526.
- Valastro, V., Holmes, E.C., Britton, P., Fusaroa, A., Jackwood, M.W., Cattoli, G. & Monne, I. (2016). S1 gene-based phylogeny of infectious bronchitis virus: an attempt to harmonize virus classification. Infection, Genetics and Evolution, 39, 349–364. doi: 10.1016/j.meegid.2016.02.015
- van Empel, P., van den Bosch, H., Goovaerts, D. & Storm, P. (1996). Experimental infection in turkeys and chickens with Ornithobacterium rhinotracheale. Avian Diseases, 40, 858–864. doi: 10.2307/1592310
- Worthington, K.J., Currie, R.J. & Jones, R.C. (2008). A reverse transcriptase-polymerase chain reaction survey of infectious bronchitis virus genotypes in Western Europe from 2002 to 2006. Avian Pathology, 37, 247–257. doi: 10.1080/03079450801986529