ABSTRACT
Chlamydia psittaci is an important zoonotic pathogen and its oral route of infection plays an important role in the transmission and persistence. Bacillus cereus (B. cereus) strain, a common contaminant of animal feed and feedstuffs, can cause severe diarrhoea and malnutrition in poultry. In our previous study, a B. cereus strain (Dawu C), isolated from the haemorrhagic lungs of infected chickens, was shown to harbour two virulence genes (hblC and cytk) and was able to induce haemorrhagic lesions in the lungs, as well as gizzard erosion and ulceration (GEU) syndrome in broilers. In the present study, we tested the hypothesis that B. cereus-induced GEU would aggravate C. psittaci infection. Our results showed that SPF chickens exposed to B. cereus developed a severe GEU syndrome. More interestingly, prior infection with B. cereus facilitated C. psittaci infection, and aggravated GEU and respiratory distress, which were accompanied by high chlamydial loads in the lungs and severe lesions in respiratory organs. Moreover, levels of local inflammatory cytokines were elevated and T cell responses were impaired in the infected birds. In conclusion, GEU caused by B. cereus may facilitate chlamydial transmission from the ventriculus to the lungs.
RESEARCH HIGHLIGHTS
Bacillus cereus contributes to the gizzard erosion and ulceration syndrome in chickens.
Exposure to Bacillus cereus exacerbates pneumonia in birds following chlamydial infection.
Bacillus cereus facilitates persistent chlamydial infection and exacerbates immune responses.
Introduction
Chlamydia psittaci (C. psittaci) is an obligate intracellular bacterium that primarily infects epithelial cells. It replicates within a vacuole, known as an inclusion, in the cytoplasm. Within an inclusion, C. psittaci undergoes a unique biphasic developmental cycle in which the infectious elementary body (EB) is first transformed into a replicative reticulate body, which then re-differentiates into an EB prior to release from the infected cell (Laroucau et al., Citation2015). C. psittaci infects a variety of avian species and spreads among flocks by close contact with carriers or sick birds. During the course of infection, chlamydial EBs are released into the immediate surroundings via the respiratory tract and the cloaca, and the aerosolization of these exudates is regarded as the most important mode of transmission of the parasite (Harkinezhad et al., Citation2009). Furthermore, C. psittaci has a wide host spectrum, infecting various species of domestic animals as well as humans. Beyond that, it exhibits a variety of tissue tropisms (Knittler & Sachse, Citation2015). After infection, diseased animals or patients develop flu-like respiratory distress or systemic inflammation. Clinical manifestations and the degree of morbidity depend on multiple factors, such as the virulence of the chlamydial strain, the infection route, the immune status of the host, the presence of co-infecting agents and environmental factors (Szymańska-Czerwińska & Niemczuk, Citation2016).
Chlamydiae can survive in the gastrointestinal tracts (GITs) of virtually all animals, which makes the GIT a reservoir for persistent infection. Thus, Chlamydia muridarum has been shown to persist in the caecum for 100 days following oral infection of mice. Even though no pathological changes in the GIT were observed over this period (Yeruva et al., Citation2013). Erkkilä et al. (Citation2008) infected mice with Chlamydia pneumoniae via the intragastric route, but no lesions developed in the GIT. However, chlamydial loads in lungs were positive until day 34 post infection (Erkkilä et al., Citation2008). Oral uptake of C. psittaci also resulted in systemic dissemination in ducks. Chlamydiae could be detected in spleen, liver, lungs and GITs at different sites for 10 days post infection. Thus, faecal-oral transmission appears to be a natural and significant route for chlamydial infection (Thierry et al., Citation2016).
In its broadest sense, Bacillus cereus (B. cereus) refers to a cluster of genetically similar forms assigned to the genus Bacillus, consisting of at least eight species (Jensen et al., Citation2003). B. cereus is cosmopolitan and is capable of forming spores, which allows it to survive under the most extreme conditions (Nicholson, Citation2002). Moreover, it is responsible for the spoilage of various foods and for food-borne infections that can cause vomiting or diarrhoea in humans. Non-gastrointestinal infections have also been reported in people with immunological dysfunction, as well as in neonates and trauma patients (Bottone, Citation2010). It can also cause downgrading of eggs, owing to premature loss of freshness and flavour, and reduced storage potential (Pan et al., Citation2011). In addition, B. cereus is one of the most significant contaminants of milk products and an aetiological agent of mastitis in ruminants (Andreas & Juan, Citation2011; Boor et al., Citation2017). Almost every species of B. cereus is able to synthesize a number of virulence factors, including enterotoxins (Nhe), haemolysins (Hbl, Cytk), phospholipases (Hly) and emetic toxin (cereulide). These toxins are able to promote intestinal cell destruction and compromise the function of the host immune system (Agata et al., Citation2002; Jeßberger et al., Citation2014).
Perhaps surprisingly, Bacillus species also have a long history as constituents of probiotics (Gaggìa et al., Citation2010). Despite the fact that members of the B. cereus group have been recognized as opportunistic pathogens, some of them are still in use as additives in human medicine (Bactisubtil, Bacillus CIP 5832) and animal feed (Paciflor, Bacillus CIP 5832; Toyerocin, B. cereus var. toyoi) (Cutting, Citation2011). Probiotic products are claimed to maintain the balance of gastrointestinal microbiota, improve nutrient utilization ratio and protect the GIT from some pathogenic infections (Chaucheyras-Durand & Durand, Citation2010). A recent survey of probiotics contaminated with B. cereus strains in China underlined the potential risks of using these bacteria. It revealed that most products were mislabelled, and that the strains they contained often carried toxin genes and/or transferable antimicrobial resistance genes (tet45) (Zhu et al., Citation2016). Paciflor has been withdrawn from the European market because it was found to contain haemolysin BL (Hbl) and non-haemolytic enterotoxin (Nhe) (Sánchez et al., Citation2009). Recently, we isolated and identified a B. cereus-like strain from a dead hen and its feed. This strain was subsequently found to belong to the B. cereus group and shown to harbour virulence factors (Zhang et al., Citation2019).
Nowadays, C. psittaci infection is prevalent on commercial poultry farms (Yin et al., Citation2015; Guo et al., Citation2016; Zuo et al., Citation2018), but its level of persistence is unknown. It is not clear whether there is any link between feed-borne B. cereus and chlamydial infection. In this study, chickens were inoculated with C. psittaci by the intranasal (i.n.) or intraesophageal (i.e.) route after or prior (and ongoing) exposure to B. cereus, chlamydial persistence and respiratory distress were evaluated to understand the impact of feed-borne B. cereus on the course of C. psittaci infection.
Materials and methods
Experimental birds and study design
Eighty 7-day-old specified pathogen-free (SPF) chickens purchased from Weitong Merial Laboratory Animal Co., Ltd. (Beijing, China) were used in this study, which was carried out in the Animal Experimental Center at China Agricultural University (Beijing, China). All birds were maintained in strict accordance with the Regulations for the Administration of Affairs Concerning Experimental Animals issued by the State Council of the People’s Republic of China. The protocols were approved by the Institutional Animal Care and Use Committee (IACUC) of the China Agricultural University.
Specified pathogen-free chickens were randomly assigned to four groups (20 birds/per group). Chickens from the four groups were weighed every week from the beginning to the end of the study. The birds in two of these groups each received 1 × 108 CFU of B. cereus via the oral route every day for 7 days. PBS solution alone was administered orally to those in the other two groups for the same period. The 14-day-old chickens in both treatment groups were then inoculated with 1 × 107 live C. psittaci EBs, either intranasally or by the intraesophageal route. All chickens were inoculated with C. psittaci only once (on day 14), but birds inoculated with B. cereus prior to exposure to the chlamydial pathogen continued to receive these oral infusions daily for the duration of the experiment (Figure S1). Although C. psittaci is generally regarded as a respiratory pathogen, it actually causes more widespread infection, especially of the GIT (Knittler & Sachse, Citation2015). In order to investigate the effects of Chlamydia and B. cereus co-infection of the GIT, we also inoculated chickens with Chlamydia via the intraesophageal route to avoid possible respiratory contamination through the larynx. We chose not to employ a PBS control group in this case because C. psittaci infection in avian hosts has been addressed in many previous studies including our own (Liu et al., Citation2015). We focused on the issue of whether the pathogenic B. cereus could enhance the infection of C. psittaci in chickens.
Identification and propagation of B. cereus isolate
The B. cereus strain used here (hereafter referred to as Dawu C) was isolated from the lungs of a 170-day-old hen which suffered respiratory distress before its demise. The same strain was also isolated from the feed given to the same bird flock. Post mortem examination revealed severe haemorrhage of lungs and erosion of the koilin layer, as well as ulceration on the mucosa of the gizzard. The isolates were identified based on the morphology of colonies grown on selective mannitol egg-yolk polymyxin (MYP) media and on Gram staining. Biochemical activities were analysed with commercial reagents (Sigma–Aldrich, Beijing, China). Bacterial DNA was prepared for 16S rRNA sequencing. The virulence genes nhe(A,B,C), cytk, ces and hbl(A,B,C,D) were detected by PCR as described previously (Guinebretière et al., Citation2002; Ehling-Schulz et al., Citation2005). The Dawu C strain was propagated in LB solution and the number of CFU was quantified on mannitol egg-yolk polymyxin-agar plates (Savini, Citation2016).
Preparation of C. psittaci EBs
The C. psittaci HJ strain was propagated in the Buffalo green monkey kidney (BGMK) cells cultured at 37°C in the presence of 5% CO2 for 72 h (Zhang et al., Citation2015). EBs were harvested and purified by density-gradient centrifugation. Purified EBs were quantified following direct fluorescent staining, then stored in sucrose phosphate glutamate (SPG) buffer at −70°C until use (Li et al., Citation2005). Before inoculation, EBs were diluted with SPG medium. Each bird in the intranasally infected groups was treated with 200-µl preparations containing 1 × 107 EBs. In the case of the intraesophageal groups, each bird received a 500-µl solution containing 1 × 107 EBs.
Detection of anti-chlamydia antibodies by ELISA
Blood samples were collected weekly from all chickens after the chlamydial challenge. Sera were obtained by centrifugation at 1509 × g for 10 min and stored at −20°C until use. Levels of antibodies specific for the C. psittaci major outer membrane protein (MOMP) were determined with a customized MOMP ELISA kit [Chlamydia ELISA rMOMP A testing kit for chickens, developed in-house and supplied by Dr. Daisy Vanrompay (Department of Molecular Biotechnology, Faculty of Bioscience Engineering, Ghent University, Belgium)].
Lymphocyte proliferation
Before Chlamydia challenge, six blood samples per group were collected from ulnar veins and treated with heparin saline (10 mg/ml). Lymphocytes were isolated using a commercial kit (Solarbio, Beijing, China). Each sample (106 cells/well) was inoculated into a 96-well plate in duplicate. To identify C. psittaci-specific stimulatory effects on lymphocytes, one of each pair of wells was stimulated with 1 × 106 IFUUV-inactivated EBs and the other was treated with PBS as control. After propagation at 37°C in 5% CO2 for 48 h, lymphocyte proliferations were measured twice for each sample and the sample mean ± standard deviation (SD) was determined (based on immunofluorescence at 450 nm) using cell proliferation BrdU ELISA kits (Abcam, Beijing, China). The stimulation index (SI) was calculated as recommended in the protocol supplied with the kit.
The method used for UV-inactivation of EBs was described previously (Li et al., Citation2010). The purified, quantified EBs were plated in a petri dish, which was exposed for 30 min to a 15 W UV light positioned at a distance of 20 cm from the plate surface (Stary et al., Citation2015). To confirm the complete inactivation, EBs were tested on fresh BGMK cells after 24 h of cultivation. Then the cells were washed with 1 × PBS three times and fixed with ice-cold methanol. Cells were stained with fluorescence-labeled anti-MOMP antibodies and examined under the microscope to determine whether they contained Chlamydia inclusions.
Lung lavage fluids and measurement of cytokine production
Lung lavage fluids from five birds per group were harvested with a home-made tool (Figure S2) after euthanasia every week following the chlamydial challenge (Holt et al., Citation2005). After chickens were exposed to high concentrations of CO2 and loss of consciousness had been confirmed, 5 ml of sterilized PBS solution was injected into the lungs of each bird and 3 ml of lung lavage fluids was retrieved. Aliquots (200 μl) of each sample were used to measure the cytokines IL-6 and IL-10 with self-assembled commercial ELISA kits (Kingfisher Biotech Inc., Saint Paul, MN, USA).
Autopsy and gross lesions
After the collection of lung lavage fluids, the same five chickens from each group were subjected to lesion examination in air sacs, lungs and gizzards. Gross lesions observed in chicken lungs and air sacs were scored according to standards set out in our earlier study (Chu et al., Citation2016). Classification of gizzard ulcerations was performed as described previously (Okabe et al., Citation1976).
Quantification of chlamydial loads by qPCR in lung tissues
The lungs of five euthanized chickens from each group were aseptically dissected and roughly 1 g of tissue was collected from each lung, weighed on an electric scale and stored in 2SP buffer. Total DNA was extracted with a Universal DNA mini-kit (QIAGEN, Hilden, Germany). C. psittaci HJ genomic DNA was extracted from purified EBs by using a Bacterial Genomic DNA kit (TransGen Biotech, Beijing, China). The concentration and quality of the DNAs were confirmed with a Nano300 instrument (Allsheng, Hanzhou, China). Based on C. psittaci 16S rRNA (GenBank Accession No. NR_036864.2), forward (5′GCCAGTACAGAAGGTAGCAATATCG-3′) and reverse (5′-TGACACGCCATTACTAGCAATTC-3′) primers were used to amplify a fragment of 118 bp.
This fragment was then cloned into the pMD-19 T vector (Takara Bio Inc., Beijing, China) as a standard reference.
Absolute quantification of RT–PCR was performed using Transgen’s SYBR Green PCR kit (TransGen Biotech) and an ABI StepOnePlus Real-time PCR system under the following amplification conditions: denaturing (one cycle at 94°C for 2 min); PCR (40 cycles at 94°C for 30 s each; 59°C annealing for 30 s; 72°C for 30 s); melting (one cycle at 94°C for 5 s; 59°C for 20 s; 94°C for 5 s).
Statistical analysis
Body weight, lymphocyte proliferation and cytokine levels were analysed by one-way ANOVA with the LSD post hoc test. Antibody titres and chlamydial loads were log-linearized and calculated in the same way. The results are presented as means and standard deviations in charts. Gross lesions were analysed with the Wilcoxon and Mann–Whitney tests. All the statistical analyses were performed with SPSS version 25.0. Significant differences are indicated in the Figures (*P < 0.05; **P < 0.01).
Results
Characterization of the Dawu C isolate of B. cereus
After 24 h of cultivation of the Dawu C isolate on mannitol egg-yolk polymyxin agar, we observed white colonies surrounded by the pink precipitation zone caused by the hydrolysis of egg yolk typical of B. cereus. Light microscopy revealed the isolate to be a Gram positive, rod-shaped bacterium. Biochemical tests were consistent with the results for B. cereus in a previous study (Buesa Muñoz, Citation1981). Sequencing of 16S rRNA confirmed that the isolate belonged to the B. cereus group, as the level of identity was above 99%. A PCR-based search for potential virulence genes revealed that the Dawu C isolate harboured hblC and cytk genes.
Ongoing exposure to B. cereus exacerbates weight loss following chlamydial challenge
Although the body weight of all chickens increased over time, marked differences were observed between the groups inoculated with or not with B. cereus (“BCE”) both prior to and post infection with C. psittaci (“CPS”) (). From day 21 to day 42, chickens that had been inoculated with B. cereus (“BCE”) were lighter than those in the CPS-treated groups. Both B. cereus-treated groups displayed persistent clinical signs of intermittent diarrhoea. Furthermore, the BCE + CPS (i.e.) group exhibited a significantly greater decrease in body weight than did the CPS (i.e.) group from day 21 to day 42. Compared with the CPS (i.e.) group, the BCE + CPS (i.e.) group exhibited significant body weight losses on days 21 (P = 0.001), 28 (P = 0.000), 35 (P = 0.000) and 42 (P = 0.000). In the BCE + CPS (i.n.) group, weight loss set in later and became significant only on day 35 (P = 0.011) and 42 (P = 0.001), respectively as compared to that of the CPS (i.n.) group. Relative to the body weight loss seen in the CPS (i.n.) group, the CPS (i.e.) group was found to show statistically significant differences on days 28 (P = 0.003), 35 (P = 0.007) and 42 (P = 0.002). In the B. cereus-treated groups, the BCE + CPS (i.e.) group first showed a significant decrease in body weight relative to the BCE + CPS (i.n.) group on day 42 (P = 0.002).
Figure 1. Mean body weights of the four groups of chickens were measured weekly following the first exposure to B. cereus (where indicated) on day 7 and one-off inoculation with C. psittaci on day 14. The asterisks on days 21 and 28 represent significant differences between the BCE + CPS (i.e.) and CPS (i.e.) groups; asterisks on days 35 and 42 indicate significant changes in body weight between the BCE + CPS (i.e.) and CPS (i.e.) groups, and between the BCE + CPS (i.n.) and CPS (i.n.) groups.
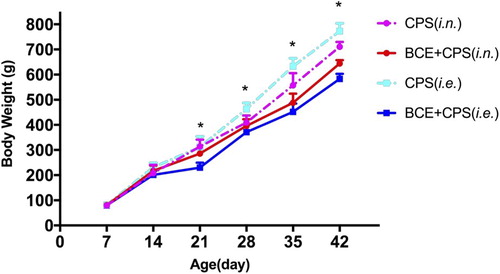
Effects of B. cereus on C. psittaci-specific immune responses and cytokine production
Specific IgG antibody responses elicited at different time-points following infection with BCE + CPS (i.e.), or BCE + CPS (i.n.), or CPS alone, were measured by subjecting serum samples to a standard ELISA assay (). On day 21, significantly higher IgG antibody titres were found in the BCE + CPS (i.n.) group and the CPS (i.n.) group (P = 0.007) relative to those of the BCE + CPS (i.e.) group (P = 0.047 and 0.007, respectively) and those of the CPS (i.e.) group (P = 0.004 and 0.001, respectively). While overall antibody levels declined gradually in all four groups from day 28 to day 42, antibody titres remained significantly higher in members of the BCE + CPS (i.n.) group (P = 0.004) and the CPS (i.n.) group (P = 0.005) compared to those of the CPS (i.e.) group on day 42. Interestingly, by the endpoint of the experiment, antibody titres had reached similar levels in both the BCE + CPS (i.e.) and BCE + CPS (i.n.) groups.
Figure 2. C. psittaci-specific antibody titres in serum were measured at weekly intervals beginning on day 21. The results are shown as geometric means and geometric standard deviations. Both the BCE + CPS (i.n.) and BCE + CPS (i.e.) groups showed lower IgG antibody titres than the CPS (i.n.) group.
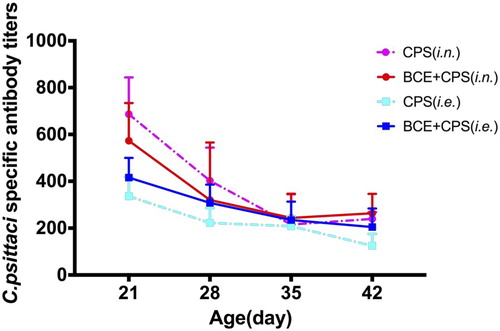
All groups showed a decrease in the peripheral blood lymphocyte (PBL) stimulation index over time. B. cereus administration clearly compromised lymphocyte proliferation in both BCE + CPS (i.e.) and BCE + CPS (i.n.) groups in comparison with the CPS (i.n.) group (). Although the lymphocyte stimulation level in the BCE + CPS (i.e.) group was comparable to those seen in the three groups, it declined at a slower rate than in the BCE + CPS (i.n.) group, finally reaching a similar value to that of the BCE + CPS (i.n.) group.
Figure 3. Proliferation index of C. psittaci-specific lymphocytes in peripheral blood lymphocytes. The BCE + CPS group (i.e.) exhibited the lowest stimulation index from day 28 to day 42. *P < 0.05; **P < 0.01.
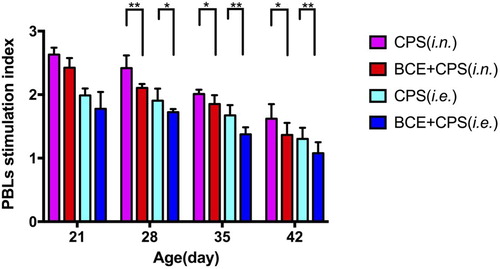
The BCE + CPS (i.e.) group exhibited a markedly higher IL-6 level than the CPS (i.e.) group on days 21 and 42. However, the BCE + CPS (i.n.) group only showed a relatively higher IL-6 concentration on days 28 and 35, owing to a pronounced rise in the CPS (i.n.) group in the final week. Both the BCE + CPS (i.e.) and BCE + CPS (i.n.) groups displayed significantly increased IL-10 levels as compared to the CPS (i.e.) group on days 35 and 42 ().
Figure 4. Levels of interleukins found in lung lavage fluids at four different timepoints. (A) Levels of IL-6 were higher in the BCE + CPS (i.e.) group than in the CPS (i.e.) group at all four times. (B) Both BCE + CPS (i.n.) and BCE + CPS (i.e.) groups produced higher amounts of IL-10 cytokines than the CPS (i.e.) group. The asterisks indicate significant differences between the co-infected groups and the group infected with C. psittaci alone.
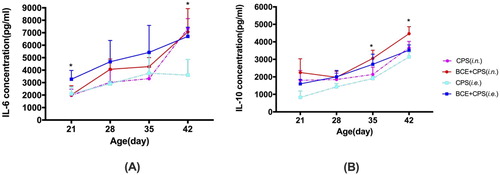
B. cereus induces ulceration and erosion of the gizzard
On the post mortem analysis, severe erosion and ulceration of gizzards were found in members of both B. cereus-treated groups (A). Most importantly, co-infected groups displayed much more severe gizzard erosion and ulceration syndrome (GEU) lesions than did either of the groups infected with C. psittaci alone (B). Not surprisingly, lesion scores were generally higher in the BCE + CPS (i.e.) group than in the CPS (i.n.) group or in the CPS (i.e.) group, and this difference was found to be statistically significant.
Figure 5. Gross lesions in the gizzards of treated chickens. (A) Erosion of the koilin layer of the gizzard and ulceration of muscular layers caused by administration of B. cereus. (B) GEU lesion scores. Five birds from each group were sacrificed at each time point. Asterisks indicate significant differences between the BCE + CPS-treated groups and the CPS-treated groups *P < 0.05.
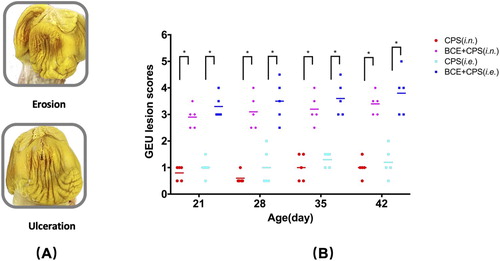
Continuing exposure to B. cereus aggravates air sac lesions and lung inflammation in co-infected birds
Following inoculation with C. psittaci via the intranasal route, the air sacs developed thickened membranes with fibrinous exudates, while haemorrhage and red foamy exudates were observed on the surfaces of lungs in the CPS group (i.n.) and in the BCE + CPS (i.n.) group (A). However, while no obvious lesions were found in thoracic air sacs in birds infected via intraesophageal route, haemorrhagic lesions were detected in the lungs. Lesions developed progressively in all groups. On day 28, the BCE + CPS (i.e.) group showed notably more severe lesions than the CPS group (i.e.) (P = 0.049), but this difference essentially disappeared at later timepoints. Similarly, by day 42, the lesions found in the BCE + CPS (i.e.) and BCE + CPS (i.n.) groups had become equally severe (B).
Figure 6. Lesions in the air sacs and lungs following inoculation with BCE + CPS or CPS alone. (A) Haemorrhagic inflammation in the lungs. (B) Lesion scores in air sacs and lungs. Five birds of each group were sacrificed at each time point. Higher lesion scores were assigned to the BCE + CPS (i.n.) group than any of the other groups on days 21 and 28. *P < 0.05.
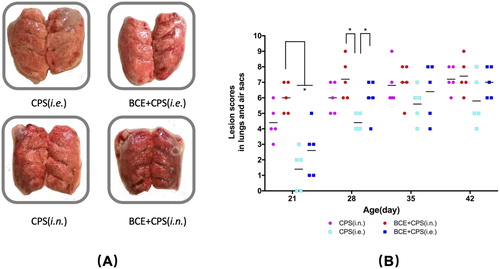
B. cereus facilitates persistent chlamydial infection in the lungs
Levels of chlamydial DNA in the lungs of chickens in the BCE + CPS (i.n.) group were significantly higher than in any of the other groups (P < 0.01) on day 21 (). From day 21 to day 28, amounts of chlamydia-specific DNA in the BCE + CPS (i.e.) group rose significantly, to become comparable to the levels found in the CPS (i.n.) and the BCE + CPS (i.n.) groups at this time point but higher than that in the CPS (i.e.) group (P = 0.002). On day 42, no significant differences were found among the BCE + CPS (i.n.), BCE + CPS (i.e.) and CPS (i.n.) groups but the chlamydial load in the CPS (i.e.) group was clearly lower than in any of the other three groups (P < 0.01).
Figure 7. Detection of chlamydial DNA in the lungs on days 21 and 42 by the qPCR method. Higher levels of chlamydial DNA were detected in the BCE + CPS (i.n.) group than in the BCE + CPS (i.e.) group on days 21 and 28. In contrast, amounts of chlamydial DNA increased gradually in the BCE + CPS (i.e.) group from day 21 to day 35. *P < 0.05; **P < 0.01.
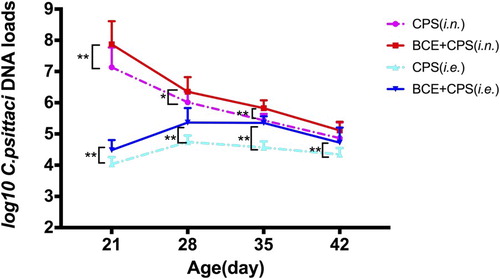
Discussion
In the current study, chickens inoculated with BCE + CPS either intranasally or intraesophageally developed much more severe GEU than birds exposed to CPS alone. Interestingly, both groups exhibited more severe lesions in air sacs and lungs on day 28. However, lower levels of lymphocyte proliferation were induced in the BCE + CPS (i.e.) group as compared to other groups. Taken together, these observations indicate that prior infection with B. cereus facilitates C. psittaci infection, and aggravates GEU and respiratory distress.
Chickens exposed to B. cereus were characterized as displaying typical GEU from day 21 to day 42, and a higher incidence of GEU was found in the BCE + CPS (i.e.) group at all timepoints. The pathogenesis of typical GEU is unknown, but it may be attributable to injury to the koilin layer of the gizzard following administration of B. cereus, while C. psittaci infection via the intraesophageal route further facilitates GEU development. In the literature, GEU syndrome has been associated with many factors, including congenital factors, starvation, the composition of feed, toxic material, microbial infections and colonization (Gjevre et al., Citation2013). It has been shown that B. cereus spores adhere to the hydrophobic surfaces of epithelia (Andersson et al., Citation1998). Around 80% of the koilin layer of the gizzard is made up of proteins that contain high levels of leucine and arginine. These proteins may contribute to the formation of a biofilm that is resistant to gastric acid and mechanical digestion (Majed et al., Citation2016). Secondly, the metabolism of B. cereus and possibly the production of toxins could directly damage the gizzard membrane. Indeed, both Hbl and Cytk are haemolysins that impair the koilin layer of the gizzard, leading to ulceration in the long term. Moreover, Hbl contributes to diarrhoea by damaging the GIT (Ehling-Schulz et al., Citation2006). The crystal structure of Hbl is homologous to that of the pore-forming haemolysin of Escherichia coli, which triggers dermonecrotic activity and alters the permeability of blood vessels (Wijnands et al., Citation2002). Furthermore, Cytk displays both cytotoxic and haemolytic properties, belongs to the family of β-barrel channel-forming toxins and induces pores in epithelial cells as well (Hardy et al, Citation2001). These two secreted toxins generally reach their maximum activity near the end of the exponential phase (Fagerlund et al., Citation2010). Unfortunately, the amounts secreted by B. cereus Dawu C could not be determined owing to the lack of commercially available kits. Further studies will need to be done in order to understand the potential association between the two toxins and gizzard ulceration following exposure to B. cereus.
In light of the higher respiratory lesion scores in the BCE + CPS (i.n.) and BCE + CPS (i.e.) groups on day 21, and the fact that the BCE + CPS (i.n.) group developed more severe lesions from day 21 to day 35 than did the control CPS group, it is likely that B. cereus aggravates the inflammation of the respiratory tract associated with intranasal introduction of CPS. Among the possible reasons are B. cereus-mediated pneumonia and thymus dysfunction. Post mortem analyses revealed haemorrhagic lesions in both the thymus and lungs after the daily administration of B. cereus. Following subsequent inoculation with C. psittaci, both air sacs and lungs were subjected to further damage, and haemorrhagic lungs exhibited exudate lesions, which might lead to respiratory distress and breathing dysfunction. More important, haemorrhagic inflammations in the thymus might greatly impair T-cell activation, and facilitate chlamydial transmission in the respiratory tract, leading to much more severe lesions (Abraham et al., Citation1989; Chu et al., Citation2016; Fu et al., Citation2019). In our study, higher levels of chlamydial DNA were found in both the BCE + CPS (i.n.) and the BCE + CPS (i.e.) groups relative to the CPS (i.e.) group on days 21 and 35. On the other hand, chlamydial DNA concentrations increased gradually in the BCE + CPS (i.e.) group from day 21 to day 35, but had decreased again by day 42, indicating that B. cereus-mediated GEU might facilitate chlamydial transmission from the GIT to the respiratory system via the bloodstream. According to a previous report, ducklings given a high oral dose of C. psittaci developed none of the typical signs of chlamydiosis except for mild diarrhoea, and no histopathological lesions were found in their organs. However, chlamydial loads were increased in the spleen, liver, GIT and lungs (Thierry et al., Citation2016). In another study, mice infected with C. pneumoniae via the intragastric route were found to have only low-level chlamydial loads in the lungs and no significant changes were detected at later times (Erkkilä et al., Citation2008). Therefore, C. psittaci is able to reach the lungs from the GIT but the resulting lesions and chlamydial loads may vary with strain virulence and tissue tropism.
Furthermore, exposure to B. cereus prior to infection with C. psittaci via either the intranasal or intraesophageal route was associated with lower induction of C. psittaci-specific IgG antibodies and weaker lymphocyte proliferation than was observed in the CPS group (i.n.). Moreover, levels of both secreted IL-6 and IL-10 were markedly elevated in lung lavages obtained from the BCE + CPS (i.n.) and BCE + CPS (i.e.) groups. Although Th-1-associated cytokines such as IL-2, IL-12 and IFN-γ are known to be critical for the limitation of intracellular infection and the clearance of Chlamydia in hosts, we did not find any significant differences in their titres between the four groups. IL-6 levels have been shown to increase gradually in mice post C. muridarum respiratory infection (Williams et al., Citation1998) and in pigs post Chlamydia suis respiratory infection (Reinhold et al., Citation2008). In addition, IL-10 knock-out mice infected with C. muridarum developed fewer lesions than wild-type mice (Xi et al., Citation1999). Thus, IL-6 and IL-10 are regarded as useful biomarkers post Chlamydia infection. In our current study, these findings indicate that infection with B. cereus suppresses the ability to mount an effective immune response to subsequent challenge with C. psittaci and that birds might be susceptible to injury due to persistence of the chlamydial infection. Our data are in agreement with earlier reports that co-infection of C. psittaci with other pathogens induces typical airsacculitis and high mortality by aggravating immune suppression (Dumont et al., Citation2015; Chu et al., Citation2017). In another study (Erkkilä et al., Citation2008), mice inoculated orally with C. pneumoniae were shown to have lower IgG antibody titres and higher IL-10 concentrations. Moreover, mice displayed local inflammatory cell infiltration. After oral reinfection, mice showed stronger inflammatory responses than mice inoculated via the intranasal route.
Therefore, B. cereus-facilitated persistence of chlamydial infection in part accounts for the prevalence of respiratory distress in the poultry industry, and may help to explain the high C. psittaci seropositivity in diseased birds.
In conclusion, several pathogenic B. cereus have now been isolated and identified from poultry feed (Barbosa et al., Citation2005; Chaiyawan et al., Citation2010). Feed-borne B. cereus is a threat to bird health and has broad economic consequences. More importantly, persistent infection by C. psittaci will also promote transmission of the pathogen from poultry to humans. Without eradication of feed-borne B. cereus, the risk of C. psittaci infection is virtually impossible to control. An effective strategy for combating feed-originated B. cereus and a vaccine against C. psittaci infection are therefore urgently needed.
Supplemental Material
Download Zip (1,015.6 KB)Acknowledgements
We are grateful for the contributions of team members Zhenhai Han, Qin Shi, Shunqin Li and Xuan Han to this work.
Disclosure statement
No potential conflict of interest was reported by the author(s).
Additional information
Funding
References
- Abraham, E. & Freitas, A.A. (1989). Hemorrhage produces abnormalities in lymphocyte function and lymphokine generation. Journal of Immunology, 142, 899–906.
- Agata, N., Ohta, M. & Yokoyama, K. (2002). Production of Bacillus cereus emetic toxin (cereulide) in various foods. International Journal of Food Microbiology, 73, 23–27. doi: 10.1016/S0168-1605(01)00692-4
- Andersson, A., Granum, P.E. & Rönner, U. (1998). The adhesion of Bacillus cereus spores to epithelial cells might be an additional virulence mechanism. International Journal of Food Microbiology, 39, 93–99. doi: 10.1016/S0168-1605(97)00121-9
- Andres, G.C. & Juan, M.R. (2011). Mastitis: comparative etiology and epidemiology. Journal of Mammary Gland Biology and Neoplasia, 16, 339–356. doi: 10.1007/s10911-011-9234-0
- Barbosa, T.M., Serra, C.R., La Ragione, R.M., Woodward, M.J. & Henriques, A.O. (2005). Screening for Bacillus isolates in the broiler gastrointestinal tract. Applied and Environmental Microbiology, 71, 968–978. doi: 10.1128/AEM.71.2.968-978.2005
- Boor, K.J., Wiedmann, M., Murphy, S. & Alcaine, S. (2017). A 100-year review: microbiology and safety of milk handling. Journal of Dairy Science, 100, 9933–9951. doi: 10.3168/jds.2017-12969
- Bottone, E.J. (2010). Bacillus cereus, a volatile human pathogen. Clinical Microbiology Reviews, 23, 382–398. doi: 10.1128/CMR.00073-09
- Buesa Muñoz, F.P. (1981). Bacillus cereus: epidemiology, pathogenicity and pathogenesis. Revista Cubana de Medicina Tropical, 33, 121–127.
- Chaiyawan, N., Tayeeteptaikul, P., Wannissorn, B., Ruengsomwong, S., Klungsupya, P., Buaban, W. & Itsaranuwat, P. (2010). Characterization and probiotic properties of Bacillus strains isolated from broiler. Thai Journal of Veterinary Medicine, 40, 207–214.
- Chaucheyras-Durand, F. & Durand, H. (2010). Probiotics in animal nutrition and health. Beneficial Microbes, 1, 3–9. doi: 10.3920/BM2008.1002
- Chu, J., Zhang, Q., Zhang, T., Han, E., Zhao, P., Khan, A., He, C. & Wu, Y. (2016). Chlamydia psittaci infection increases mortality of avian influenza virus H9N2 by suppressing host immune response. Scientific Reports, 6, 29421. doi: 10.1038/srep29421
- Chu, J., Zhang, Q., Zuo, Z., El-Ashram, S., Guo, Y., Zhao, P., Huang, S., He, C. & Khan, A. (2017). Co-infection of Chlamydia psittaci with H9N2, ORT and Aspergillus fumigatus contributes to severe pneumonia and high mortality in SPF chickens. Scientific Reports, 7, 13997. doi: 10.1038/s41598-017-14519-1
- Cutting, S.M. (2011). Bacillus probiotics. Food Microbiology, 28, 214–220. doi: 10.1016/j.fm.2010.03.007
- Dumont, A., De Boeck, C., Vanrompay, D. & Kalmar, I. (2015). Longitudinal monitoring for respiratory pathogens in broiler chickens reveals co-infection of Chlamydia psittaci and Ornithobacterium rhinotracheale. Journal of Medical Microbiology, 64, 565–574. doi: 10.1099/jmm.0.000047
- Ehling-Schulz, M., Guinebretiere, M.-H.L.N., Monthà n, A., Berge, O., Fricker, M. & Svensson, B. (2006). Toxin gene profiling of enterotoxic and emetic Bacillus cereus. FEMS Microbiology Letters, 260, 232–240. doi: 10.1111/j.1574-6968.2006.00320.x
- Ehling-Schulz, M., Svensson, B., Guinebretiere, M.H., Lindbäck, T., Andersson, M., Schulz, A., Fricker, M., Christiansson, A., Granum, P.E., Märtlbauer, E., Nguyen-The, C., Salkinoja-Salonen, M. & Scherer, S. (2005). Emetic toxin formation of Bacillus cereus is restricted to a single evolutionary lineage of closely related strains. Microbiology, 151, 183–197. doi: 10.1099/mic.0.27607-0
- Erkkilä, L., Saario, E., Laitinen, K., Saikku, P. & Leinonen, M. (2008). Intragastric primary infection sensitizes to lung reinfection in a Chlamydia pneumoniae mouse model. Vaccine, 26, 2503–2509. doi: 10.1016/j.vaccine.2008.03.034
- Fagerlund, A., Lindbäck, T. & Granum, P.E. (2010). Bacillus cereus cytotoxins Hbl, Nhe and CytK are secreted via the Sec translocation pathway. BMC Microbiology, 10, 304. doi: 10.1186/1471-2180-10-304
- Fu, L.S., Wang, X.Y., Zhai, J., Qi, W., Jing, L., Ge, Y.Y., Gao, L.X., Liu, C.N., Lv, X.P. & Zheng, S.M. (2019). Changes in apoptosis, proliferation and T lymphocyte subtype on thymic cells of SPF chickens infected with reticuloendotheliosis virus. Molecular Immunology, 111, 87–94. doi: 10.1016/j.molimm.2019.04.003
- Gaggìa, F., Mattarelli, P. & Biavati, B. (2010). Probiotics and prebiotics in animal feeding for safe food production. International Journal of Food Microbiology, 141, S15–S28. doi: 10.1016/j.ijfoodmicro.2010.02.031
- Gjevre, A.G., Kaldhusdal, M. & Eriksen, G.S. (2013). Gizzard erosion and ulceration syndrome in chickens and turkeys: a review of causal or predisposing factors. Avian Pathology, 42, 297–303. doi: 10.1080/03079457.2013.817665
- Guinebretière, M.H. & Clinical, V.B.J.O. (2002). Enterotoxigenic profiles of food-poisoning and food-borne Bacillus cereus strains. Journal of Clinical Microbiology, 40, 3053–3056. doi: 10.1128/JCM.40.8.3053-3056.2002
- Guo, W., Li, J., Kaltenboeck, B., Gong, J., Fan, W. & Wang, C. (2016). Chlamydia gallinacea, not C. psittaci, is the endemic chlamydial species in chicken (Gallus gallus). Scientific Reports, 6, 19638. doi: 10.1038/srep19638
- Hardy, S.P., Lund, T. & Granum, P.E. (2001). Cytk toxin of Bacillus cereus forms pores in planar lipid bilayers and is cytotoxic to intestinal epithelia. FEMS Microbiology Letters, 197, 47–51. doi: 10.1111/j.1574-6968.2001.tb10581.x
- Harkinezhad, T., Geens, T. & Vanrompay, D. (2009). Chlamydophila psittaci infections in birds: a review with emphasis on zoonotic consequences. Veterinary Microbiology, 135, 68–77. doi: 10.1016/j.vetmic.2008.09.046
- Holt, P.S., Stone, H.D., Moore, R.W. & Gast, R.K. (2005). Development of a lavage procedure to collect lung secretions from chickens for evaluating respiratory humoral immunity. Avian Pathology, 34, 396–398. doi: 10.1080/03079450500268476
- Jensen, G.B., Hansen, B.M., Eilenberg, J. & Mahillon, J. (2003). The hidden lifestyles of Bacillus cereus and relatives. Environmental Microbiology, 5, 631–640. doi: 10.1046/j.1462-2920.2003.00461.x
- Jeßberger, N., Dietrich, R., Bock, S., Didier, A. & Märtlbauer, E. (2014). Bacillus cereus enterotoxins act as major virulence factors and exhibit distinct cytotoxicity to different human cell lines. Toxicon, 77, 49–57. doi: 10.1016/j.toxicon.2013.10.028
- Knittler, M.R. & Sachse, K. (2015). Chlamydia psittaci: update on an underestimated zoonotic agent. Pathogens and Disease, 73, 1–15. doi: 10.1093/femspd/ftu007
- Laroucau, K., Vanrompay, D. & Sachse, K. (2015). Avian chlamydiosis. Current Clinical Microbiology Reports, 2, 10–21. doi: 10.1007/s40588-014-0010-y
- Li, D., Vaglenov, A., Kim, T., Wang, C., Gao, D. & Kaltenboeck, B. (2005). High-yield culture and purification of Chlamydiaceae bacteria. Journal of Microbiological Methods, 61, 17–24. doi: 10.1016/j.mimet.2004.10.020
- Li, W., Murthy, A.K., Guentzel, M.N., Chambers, J.P., Forsthuber, T.G., Seshu, J., Zhong, G. & Arulanandam, B.P. (2010). Immunization with a combination of integral chlamydial antigens and a defined secreted protein induces robust immunity against genital chlamydial challenge. Infection and Immunity, 78, 3942–3949. doi: 10.1128/IAI.00346-10
- Liu, S., Sun, W., Chu, J., Huang, J., Wu, Z., Yan, M., Zhang, Q., Zhao, P., Igietseme, J.U., Black, C.M., He, C. & Li, Y. (2015). Construction of recombinant HVT expressing PmpD, and immunological evaluation against Chlamydia psittaci and Marek’s disease virus. PLoS One, 10, e0124992. doi: 10.1371/journal.pone.0124992
- Majed, R., Faille, C., Kallassy, M. & Gohar, M. (2016). Bacillus cereus biofilms – same, only different. Frontiers in Microbiology, 172, 1–16.
- Nicholson, W.L. (2002). Roles of Bacillus endospores in the environment. Cellular and Molecular Life Sciences (CMLS), 59, 410–416. doi: 10.1007/s00018-002-8433-7
- Okabe, S., Takata, Y., Takeuchi, K., Naganuma, T. & Takagi, K. (1976). Effects of carbenoxolone Na on acute and chronic gastric ulcer models in experimental animals. The American Journal of Digestive Diseases, 21, 618–625. doi: 10.1007/BF01071954
- Pan, Q., Yang, J.J., Li, S.M., Ou, C.B., Wu, Z.X. & He, C. (2011). Isolation and identification of a Bacillus cereus in deteriorated eggs. Chinese Journal of Veterinary Medicine, 04, 18–20.
- Reinhold, P., Kirschvink, N., Theegarten, D. & Berndt, A. (2008). An experimentally induced Chlamydia suis infection in pig results in severe lung function disorders and pulmonary inflammation. Veterinary Research, 39, 35. doi: 10.1051/vetres:2008012
- Savini, V. (2016). The Diverse Faces of Bacillus cereus 1st edn (pp. 18–22). Salt Lake City, UT: Academic Press.
- Sánchez, B., Arias, S., Chaignepain, S., Denayrolles, M., Schmitter, J.M., Bressollier, P. & Urdaci, M.C. (2009). Identification of surface proteins involved in the adhesion of a probiotic Bacillus cereus strain to mucin and fibronectin. Microbiology, 155, 1708–1716. doi: 10.1099/mic.0.025288-0
- Stary, G., Olive, A., Radovic-Moren, A.F., Gondek, D., Alvarez, D., Basto, P.A., Perro, M., Vrbanac, V.D., Tager, A.M., Shi, J., Yethon, J.A., Farokhzad, O.C., Langer, R., Starnbach, M.N. & Andrian, U.H. (2015). A mucosal vaccine against Chlamydia trachomatis generates two waves of protective memory T cells. Science, 348, 1331, aaa8205. doi: 10.1126/science.aaa8205
- Szymańska-Czerwińska, M. & Niemczuk, K. (2016). Avian chlamydiosis zoonotic disease. Vector-Borne and Zoonotic Diseases, 16, 1–3. doi: 10.1089/vbz.2015.1839
- Thierry, S., Vorimore, F., Borel, N., Sachse, K., Rossignol, C., Scharf, S., Berthon, P., Durand, B., Virlogeux-Payant, I. & Laroucau, K. (2016). Oral uptake of Chlamydia psittaci by ducklings results in systemic dissemination. PLoS One, 11, e0154860. doi: 10.1371/journal.pone.0154860
- Wijnands, L.M., Dufrenne, J.B. & van Leusden, F.M. (2002). The pathogenic mechanism of the diarrheal syndrome caused by Bacillus cereus. Rijksinstituut voor Volksgezondheid en Milieu RIVM Report, 250912001.
- Williams, D.M., Gradbbs, B.G., Darville, T., Kelly, K. & Rank, R.G. (1998). A role for interleukin-6 in host defense against murine Chlamydia trachomatis infection. Infection and Immunity, 66, 4564–4567. doi: 10.1128/IAI.66.9.4564-4567.1998
- Xi, Y., John, G., Lihua, Z., Shuhe, W. & Robert, C.B. (1999). IL-10 gene knockout mice show enhanced Th1-l like protective immunity and absent granuloma formation following Chlamydia trachomatis lung infection. Journal of Immunology, 162, 1010–1017.
- Yeruva, L., Spencer, N., Bowlin, A.K., Wang, Y. & Rank, R.G. (2013). Chlamydial infection of the gastrointestinal tract: a reservoir for persistent infection. Pathogens and Disease, 68, 88–95. doi: 10.1111/2049-632X.12052
- Yin, L., Kalmar, I.D., Boden, J. & Vanrompay, D. (2015). Chlamydia psittaci infections in Chinese poultry: a literature review. World’s Poultry Science Journal, 71, 473–482. doi: 10.1017/S0043933915002226
- Zhang, Q., Wu, Z.X., Chu, J., Han, E., Zhang, Y.X., Ling, Y., Gong, Y.P., Li, D.F., Wu, H.L., He, C. & Ni, P.X. (2015). Whole genome sequences of Chlamydia psittaci strain HJ isolated from meat pigeons with severe respiratory distress and high mortality. Genome Announcements, 3, e00035-15.
- Zhang, Q., Zuo, Z.H., Guo, Y.X., Zhang, T.Y., Han, Z.H., Huang, S.J., Karama, M., Saleemi, K.M., Khan, A. & He, C. (2019). Contaminated feed-borne Bacillus cereus aggravates respiratory distress post avian influenza virus H9N2 infection by inducing pneumonia. Scientific Reports, 9, 7231. doi: 10.1038/s41598-019-43660-2
- Zhu, K., Hölzel, C.S., Cui, Y., Mayer, R., Wang, Y., Dietrich, R., Didier, A., Bassitta, R., Märtlbauer, E. & Ding, S. (2016). Probiotic Bacillus cereus strains, a potential risk for public health in China. Frontiers in Microbiology, 7, 718.
- Zuo, Z.H., Zhang, T.Y., Guo, Y.X., Chu, J., Qu, G.G., Miao, L.Z., Shen, Z.Q. & He, C. (2018). Serosurvey of Avian metapneumovirus, Orithobacterium rhinotracheale, and Chlamydia psittaci and their potential association with avian airsacculitis. Biomedical and Environmental Sciences: BES, 31, 403–406.