ABSTRACT
Gallibacterium anatis is considered one of the most common bacterial causative agents of reproductive tract disorders in poultry. In this study, phylogenetic analysis of partial rpoB sequences and biotyping using MALDI-TOF MS was done in order to investigate the genetic diversity of Gallibacterium isolates from 13 farms with different biosecurity measures and management practices. Sampling was done as a part of regular monitoring, except for Farms 9–13 that were included in the study to represent extensive production systems with lowest biosecurity levels. Pharyngeal and cloacal swabs were taken from live birds, while swabs from trachea, liver, peritoneum and oviduct were taken during necropsies. After cultivation and identification, strains from each farm were randomly selected for sequencing and biotyping. Both results showed high level of heterogeneity among the isolates originating from farms with low biosecurity levels, unlike isolates from farms with higher biosecurity levels and proper management that were more closely related and clustered together. Such correlation was statistically significant. Low biosecurity levels enable horizontal transmission of the pathogens, as well as gene transfer. The results confirm the importance of adequate biosecurity measures and management on poultry farms as they greatly affect the genetic diversity of the pathogens. Therefore, implementation of basic biosecurity measures could help control the heterogeneity of Gallibacterium strains, which would alleviate control of the infection prevalence on farms through immunoprophylaxis, and consequently improve poultry production. Also, the genetic diversity of G. anatis on poultry farms could be a good bioindicator of management practices and biosecurity measures used.
RESEARCH HIGHLIGHTS
High correlation between low biosecurity and high diversity of Gallibacterium anatis.
Diversity of Gallibacterium is a good bioindicator of management practices on farms.
Introduction
Gallibacterium anatis (G. anatis) is a very common bacterial cause of reproductive tract disorders in poultry (Jordan et al., Citation2005; Bojesen et al., Citation2007; Kristensen et al., Citation2011; Varan Singh & Singh, Citation2015). Although it is a part of normal microflora in the upper respiratory and lower reproductive tract, it can cause various lesions that lead to production decrease and high-mortality rates (Bisgaard, Citation1977; Bisgaard, Citation1993; Bojesen et al., Citation2003; Christensen et al., Citation2003; Neubauer et al., Citation2009). There are no pathognomonic clinical signs or post mortem lesions that characterize the infection. It can be either acute or chronic, with more often chronic manifestation with an impact on production rates, as well as bird welfare. Vertical transmission was demonstrated in many studies related to experimental and natural infection (Paudel, Liebhart, Aurich et al., Citation2014; Paudel, Liebhart, Hess et al., Citation2014; Wang et al., Citation2016) but horizontal transmission is considered more frequent (Bojesen et al., Citation2003). The most common lesions are peritonitis, salpingitis, oophoritis, hepatitis and respiratory syndrome (Bojesen et al., Citation2004; Kristensen et al., Citation2011; Paudel et al., Citation2013; Persson & Bojesen, Citation2015; Varan Singh & Singh, Citation2015). Although various lesions have been reported, Gallibacterium has a tropism for reproductive tract tissue. Usually the respiratory tract is a place of primary colonization, from where the pathogen spreads through systemic circulation under the right circumstances (Neubauer et al., Citation2009; Paudel et al., Citation2013).
Analyses based on the partial sequences of the house keeping gene encoding the ß-subunit of the RNA polymerase (rpoB) have been successfully used in phylogenetic studies of many bacteria, such as Borrelia, Legionella, Mycobacterium, Mycoplasma and Gallibacterium (Mollet et al., Citation1997; Kim et al., Citation1999; Renesto et al., Citation2000; Renesto et al., Citation2001; Christensen et al., Citation2004; Korczak et al., Citation2004; De Zwaan et al., Citation2014). Low biosecurity measures and poor management practices could significantly influence the spread of microbes within and between the farms, which could be followed by their genetic diversity. Considering the impact of G. anatis infection on production and increasing rates of antimicrobial resistance, we wanted to investigate phylogenetic relationships of isolates within and between various farms with different biosecurity measures and management practices. The comparison was done based on the partial sequences of rpoB gene which is highly conserved within the family of Pasteurellaceae and has a high discriminatory power (Dahllof et al., Citation2000; Korczak et al., Citation2004).
Materials and methods
Selection of farms and epidemiological parameters
Farms were selected in order to represent a diverse selection of production systems, biosecurity levels and management practices (). Sampling on Farms 1–8 was done as a part of regular monitoring, while Farms 9–13 were included to represent extensive production with low biosecurity standards. Farms 9–12 regularly circulate the roosters among their flocks, participate in exhibitions and are geographically very close, so we considered them as one for the purpose of this study. All farms, except Farms 9–13, had reported Gallibacterium infections, and one of them (Farm 2) had current clinical problems. On all farms, basic epidemiological parameters were collected: number of flocks per farm, farm owns the hatchery or applies contract hatching from one or more sources, farm rears or imports their pullets, farm owns a feed mill or uses an external feed mill, one or multiple species on farm, compulsory change of clothes and footwear upon entering the farm, compulsory showering before entering the farm, workers designated to a specific flock, seasonal workers, downtime period is less than or at least 14 days and obligatory cleaning and disinfection during downtime. The values were used for calculation of epidemiological distance between the farms which are presented as a dendrogram with heat map using the online platform shinyheatmap (Khomtchouk et al., Citation2017). The results are presented using row clustering, column scaling, average linkage algorithm and euclidean distance metric.
Table 1. Description of farms included in the study.
Bacterial strains
Pharyngeal and cloacal swabs were taken from live birds, while swabs from trachea, liver, oviduct and peritoneum were taken during necropsies. Samples were then plated on Columbia agar (Oxoid, Basingstoke, UK) enriched with 5% sheep blood (Biognost, Zagreb, Croatia) and incubated aerobically at 37°C overnight. Identification was done based on morphological characteristics and biochemical analyses, and then was confirmed by Bruker Microflex LT matrix assisted laser desorption ionization- time of flight mass spectrometry (MALDI-TOF MS) (Bruker Daltonics, Bremen, Germany). All the samples were stored in BHI broth (Oxoid, Basingstoke, UK) with 50% glycerol at −80°C until further analyses. Strains from each farm were randomly chosen for sequencing. One strain per bird was selected for the analysis; the total number of selected strains per farm varied from two to ten, and these were grouped relating to the flock of origin.
DNA extraction, amplification and purification
DNA extraction was done using Chelex 100 (BioRad, Hercules, CA, USA) according to the manufacturer’s instructions. DNA concentration was measured using BioDrop µLite (Cambridge, UK) and adjusted to 20 ng/µl for amplification through polymerase chain reaction. The primers used were rpoB-F (5′-GCAGTGAAAGARTTCTTTGGTTC-3′) and rpoB-R (5′-GTTGCATGTTIGIACCCAT-3′) (Korczak et al., Citation2004). The reaction mixture was composed of 25 µl GoTaq G2 Hot Start Green Master Mix (Promega, Madison, WI, USA), 2 µl each of 10 pmol forward and reverse primers, 5 µl of DNA and 16 µl of Nuclease-Free Water (Promega, Madison, WI, USA) (total volume = 50 µl). The cycling parameters for amplification included: 94°C for 4 min and then 35 cycles of denaturation at 94°C for 30 s, annealing at 51°C for 30 s, and elongation at 70°C for 1 min; and final elongation at 70°C for 7 min. Polymerase chain reaction products were visualized using 1% agarose gel electrophoresis, stained with Midori Green Advance (Nippon Genetics Europe GmbH, Düren, Germany). The expected amplicon size was 560 bp. Purification of the amplicons from gel slices was done using ReliaPrep™ DNA Clean-up Concentration System (Promega, Madison, WI, USA) and Wizard® SV Gel and polymerase chain reaction Clean-Up System (Promega, Madison, WI, USA) for the first 34 isolates and the second 18 isolates, respectively, while the combination of Zymoclean™ Gel DNA Recovery Kit (Zymo Research, Irvine, CA, USA) and DNA Clean & Concentrator™ Zymo Research, Irvine, CA, USA) was used for the remaining 11 isolates. All the procedures were done according to the manufacturers’ instructions. The isolates were then subjected to sequence analysis (Macrogen Inc., Amsterdam, The Netherlands).
Phylogenetic analysis
Sequences were edited and cut to equal lengths for alignment using BioEdit Sequence Alignment Editor version 7.0.5.3 (Hall, Citation1999). A query was made using sequences through the basic local alignment search tool (BLAST) of the National Center of Biotechnology Information (NCBI, USA) to search for similar organisms based on sequences available in GenBank. A phylogenetic tree was constructed by the Maximum Likelihood method and Hasegawa–Kishino–Yano model with Gamma distribution (Hasegawa et al., Citation1985) using Molecular Evolutionary Genetics Analysis (MEGA X) version 10.0.5. (Kumar et al., Citation2018). Sequences available in GenBank with the accession numbers NC015460.1, GQ464988.1, HQ629846.1, HQ629831.1 and AY314033.1 were used for construction of the phylogenetic tree as they showed high similarities to our sequences according to BLAST, while Haemophilus influenzae (AAC22173.1) was used as an outgroup. Identity of 99.80% or higher was considered a cut-off value for defining a cluster. Sequences analysed in this study were deposited in GenBank under accession numbers MN342174-MN342232 and MN442324- MN442327. Detailed description is given in the supplementary material (Supplementary Table S1).
Dendrogram construction
A dendrogram was constructed using MALDI Biotyper 3.0 software (Bruker Daltonics) based on mass signal patterns. Distance measure was set at correlation, linkage at average and score threshold value for a single organism at 700 (Alispahic et al., Citation2012). H. influenzae ATCC 35056 THL was used as an outgroup.
Statistical analysis
The data were analysed using nonparametric Spearman rank test in order to determine a correlation between the biosecurity level of the farm and the number of clusters per farm formed in the phylogenetic and biotyping analyses. The analyses were done in Statistica (Statistica 13.5.0.17., Tulsa, OK, USA) software. The statistical significance was set at P < 0.05.
Results and discussion
Our study was focused on the phylogenetic relationships of the Gallibacterium isolates from farms with different levels of biosecurity measures and management practices. Out of 63 sequences used for the analysis, 62 were identified as G. anatis, while one was identified as Gallibacterium genomospecies 1 (). Both taxa share phenotypic similarities (Christensen et al., Citation2003), which complicates correct identification without genotyping. Farm 1 and Farm 2 have their own parent flock, hatchery and same rearing farm that are situated in the proximity of Farm 2. However, isolates from the mentioned farms did not cluster altogether. Isolates from Farm 1 showed heterogeneity in the analysis and occurred individually in very distant subgroups with isolates from various farms, as opposed to isolates from Farm 2 that were very closely related. Unlike workers on Farm 2, workers on Farm 1 are not required to shower before entering the farm and they manage all poultry houses. Substantial phylogenetic distance between the isolates from both farms indicates that horizontal transmission of Gallibacterium is more dominant than vertical transmission. As expected, phylogenetic analysis showed a high level of homogeneity of the isolates from Farm 2 and Farm 4, as they have pullets only from their own rearing farms and have high biosecurity levels compared to other farms. Only two isolates from Farm 3 were included in the study but they were closely related and, surprisingly, clustered with isolates from Farm 4. Farm 5 and Farm 6 showed high diversity amongst the isolates, which corresponds to low biosecurity levels and poor management practices on the farms. Both farms source pullets from a number of rearing farms, their own and from abroad, and do not apply “all-in-all-out” principle. On the contrary, isolates from Farm 7 showed uniformity and were grouped together, except for one isolate. Farm 7 has its own rearing farm, and it applies high biosecurity measures. The workers are required to shower-in before entering the farms and they are assigned to a specific flock. Isolates from Farm 8 were allocated into three clusters. Although the farm applies high biosecurity measures, they do not have their own rearing farm and they import pullets from various sources, which explains the diversity of the isolates. Farms 9–12 are backyard flocks reared to conserve the indigenous Croatian chicken breed. Given the purpose, the breeders regularly circulate the roosters to prevent inbreeding in their flocks and they participate in exhibitions, which accounts for a very low biosecurity level. Since the mentioned farms are geographically very close, we considered them as one for the purpose of this study. The isolates originating from Farms 9–12 showed a high level of phylogenetic diversity and clustered in many subgroups with isolates from various farms, which can be explained by mandatory introduction of new birds to the flock and circulation between farms that promotes horizontal transmission of microorganisms. Farm 13 is also a backyard flock with the same indigenous breed but the chickens are being raised solely as the owner’s hobby. They originate from a different breeder than those from Farms 9–12, they have never been taken to exhibitions, and no roosters from other farms have been introduced to the flock. Interestingly, isolates from Farm 13 were the most separately clustered and were distant to the isolates from Farms 9–12.
Figure 1. (a) Phylogenetic tree of Gallibacterium isolates based on comparison of partial rpoB sequences. Data was analysed using the Maximum likelihood method based on the Hasegawa–Kishino–Yano model in MEGA X. In total, 413 positions were used in the final dataset and a discrete Gamma distribution was used to model evolutionary rate differences among sites. (b) Graph showing correlation with linear fit between number of clusters according to the phylogenetic analysis and epidemiological data used in the study with correlation coefficient and statistical significance value.
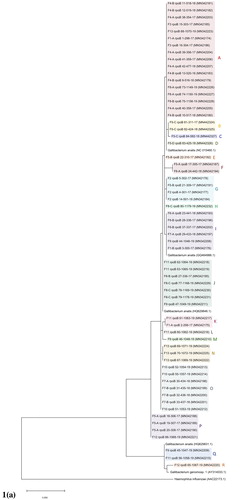
We used 11 epidemiological parameters for the construction of the heat map with dendrogram (), and combined them with number of clusters per farm, shown in (a). The heat map dendrogram shows the separation of farms according to their biosecurity/management standards, which is in congruence with lower and higher numbers of clusters on the same farms. The results of the correlation between the level of biosecurity/management practices on the farms and the number of clusters formed in the phylogenetic analysis ((b)) are statistically significant (r = 0.8050, P = 0.005), which proves our hypothesis. The hypothesis is also confirmed with the MALDI Biotyper showing high and significant correlation (r = 0.74334, P = 0.014) between biosecurity measures/management practices and number of clusters on the MALDI dendrogram ((b)).
Figure 2. Dendrogram with the heat map depicting relatedness of the farms according to their biosecurity measures and management practices together with the number of Gallibacterium clusters per farm. Construction of the dendrogram and the heat map was done using the values of each epidemiological parameter per farm. Epidemiological parameters used for the analyses were: par1 = number of flocks per farm; par2 = farm owns the hatchery/ contract hatching, one source/ contract hatching, multiple sources; par3 = farm rears their pullets/ pullets are imported; par4 = farm owns a feed mill/farm uses external feed mill; par5 = multiple species on farm/one species on farm; par6 = obligatory change of clothes and footwear upon entering the farm; par7 = obligatory showering before entering the farm; par8 = workers designated to a specific floc; par9 = seasonal workers; par10 = downtime period ≥14 days/ <14 days; par11 = obligatory cleaning and disinfection during downtime. Detailed description of the epidemiological parameters is available in the supplementary material (Supplementary Table S2).
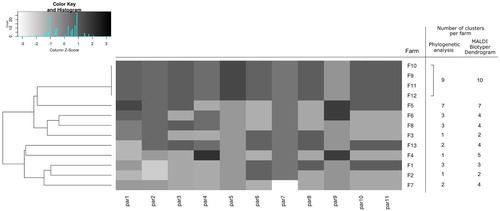
Figure 3. (a) Dendrogram based on the minimum spanning tree, constructed using MALDI Biotyper, showing the relationship between the isolates used in the study. The vertical line at a 100-distance level indicates the cut-off value for defining a cluster. (b) Graph showing correlation with linear fit between number of clusters according to dendrogram constructed with MALDI Biotyper and epidemiological data used in the study with correlation coefficient and statistical significance value.
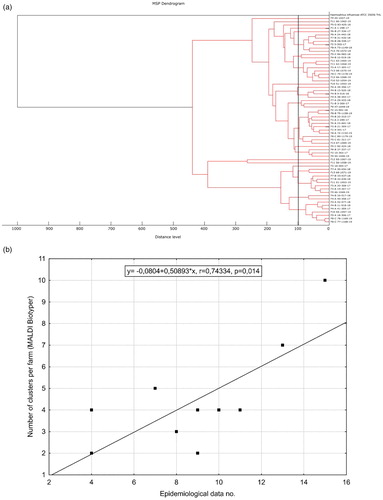
Next to immunoprophylaxis, biosecurity measures are considered the most important way of preventing infectious diseases. Although G. anatis is a part of the normal microflora in poultry and can be isolated from healthy chickens (Bisgaard, Citation1993; Bojesen et al., Citation2003), it has also been a cause of many lesions. The horizontal transmission route has been considered more frequent and relevant in the pathogenesis of Gallibacterium infection. Bojesen et al. (Citation2003) demonstrated the effect of different biosecurity levels on the prevalence of Gallibacterium spp., confirming that a lower level of biosecurity results in a higher prevalence of these mentioned bacteria. Since the extensive variation of G. anatis strains complicates the development of a universal vaccine that could provide cross-protection, prevention of the infection is currently based on application of autogenous vaccines. Implementation of high biosecurity standards could have a beneficial effect for antimicrobial control, by preventing the introduction to the farm and by decreasing the need for use of antimicrobials to treat bacterial diseases (Davies & Wales, Citation2019). Gallibacterium does not survive well in the environment due to cleaning and disinfection (Bisgaard, Citation1993); nevertheless, spread of the pathogen because of poor biosecurity and management can contribute to the genetic diversity.
Previous results on layer farms showed that G. anatis strains have a tendency to maintain clonality (Alispahic et al., Citation2012). On the other hand, our results using two different typing methods ( and ) confirmed that low biosecurity and management practices could directly influence the diversity of G. anatis isolates on the farm. Predisposing factors such as co-infection, hormonal status, impaired immunity, genetic predisposition, stress, age, poor zoohygienic conditions and even season change, additionally affect the prevalence, as well as the severity of infections caused by Gallibacterium (Bisgaard, Citation1977; Shaw et al., Citation1990; Bojesen et al., Citation2003; Jordan et al., Citation2005; Neubauer et al., Citation2009; Elhamid et al., Citation2017; Lawal et al., Citation2017; Paudel et al., Citation2017). Some of these factors can be controlled with proper management on the farm. Although introduction and maintenance of high biosecurity levels and good management on the farm could decrease the probability of horizontal transmission of the pathogen, the conditions do not always allow implementation of said measures to the practice. None of the farms in our study could be presented as an ideal model regarding biosecurity. Frequent clinical issues on smaller commercial farms are often related to mixing different ages in the same farmhouse, housing that was not properly built, or to the general lack of workers. In case of shortage of people, farmers recruit seasonal workers (who are not familiar with biosecurity plans) for labour like vaccination or relocation of flocks. However, the main cause of the high diversity of Gallibacterium on the farms is mixing the flocks from different sources, as in the case of Farm 5. Although they have their own rearing farm, they additionally import flocks from several EU countries, which, in combination with poor biosecurity, continuously causes problems on the farm. Such imports, even with highest biosecurity, could always bring new microorganisms in, also because of dissimilar vaccination programmes. One such example is the case of infectious laryngotracheitis on a non-vaccinated farm, imported with a flock containing a silent strain vaccinated according to the immunoprophylaxis programme adequate to the epidemiological area where the rearing farm was situated.
Our data indicate that implementation of simple biosecurity measures, such as showering and changing clothes before entering the farm and having workers designated to a specific flock, could control horizontal spread, as a main route of Gallibacterium transmission, what could later contribute to the lower genetic diversity on poultry farms. Additionally, rearing management and pullet outsourcing probably have much higher impact on the Gallibacterium diversity. Therefore, genetic diversity of G. anatis on the layer/breeder farms could be a good bioindicator of management practices and biosecurity measures performed, as shown in this study.
Supplemental Material
Download MS Word (39.2 KB)Acknowledgements
The authors would like to thank Zlatko Janječić, Zlatko Herceg, poultry farmers and veterinarians for their cooperation regarding sample collection, and Maja Maurić and Zvonimir Poljak for the consultations about statistical analysis.
Disclosure statement
No potential conflict of interest was reported by the authors.
References
- Alispahic, M., Christensen, H., Hess, C., Razzazi-Fazeli, E., Bisgaard, M. & Hess, M. (2012). MALDI-TOF mass spectrometry confirms clonal lineages of Gallibacterium anatis between chicken flocks. Veterinary Microbiology, 160, 269–273. doi: 10.1016/j.vetmic.2012.05.032
- Bisgaard, M. (1977). Incidence of pasteurella haemolytica in the respiratory tract of apparently healthy chickens and chickens with infectious bronchitis: characterisation of 213 strains. Avian Pathology, 6, 285–292. doi: 10.1080/03079457708418238
- Bisgaard, M. (1993). Ecology and significance of pasteurellaceae in animals. Zentralblatt fur Bakteriologie, 279, 7–26. doi: 10.1016/S0934-8840(11)80487-1
- Bojesen, A.M., Nielsen, O.L., Christensen, J.P. & Bisgaard, M. (2004). In vivo studies of Gallibacterium anatis infection in chickens. Avian Pathology, 33, 145–152. doi: 10.1080/03079450310001652059
- Bojesen, A.M., Nielsen, S.S. & Bisgaard, M. (2003). Prevalence and transmission of haemolytic Gallibacterium species in chicken production systems with different biosecurity levels. Avian Pathology, 32, 503–510. doi: 10.1080/0307945031000154107
- Bojesen, A.M., Vazquez, M.E., Robles, F., Gonzalez, C., Soriano, E.V., Olsen, J.E. & Christensen, H. (2007). Specific identification of Gallibacterium by a PCR using primers targeting the 16S rRNA and 23S rRNA genes. Veterinary Microbiology, 123, 262–268. doi: 10.1016/j.vetmic.2007.02.013
- Christensen, H., Bisgaard, M., Bojesen, A.M., Mutters, R. & Olsen, J.E. (2003). Genetic relationship among avian isolates classified as Pasteurella haemolytica. “Actinobacillus salpingitidis” or Pasteurella anatis with proposal of Gallibacterium anatis gen. nov., comb. nov. and description of additional genomospecies within Gallibacterium gen. nov. International Journal of Systematic and Evolutionary Microbiology, 53, 275–287. doi: 10.1099/ijs.0.02330-0
- Christensen, H., Kuhnert, P., Olsen, J.E. & Bisgaard, M. (2004). Comparative phylogenies of the housekeeping genes atpD, infB and rpoB and the 16S rRNA gene within the Pasteurellaceae. International Journal of Systematic and Evolutionary Microbiology, 54, 1601–1609. doi: 10.1099/ijs.0.03018-0
- Dahllof, I., Baillie, H. & Kjelleberg, S. (2000). rpoB-based microbial community analysis avoids limitations inherent in 16S rRNA gene intraspecies heterogeneity. Applied and Environmental Microbiology, 66, 3376–3380. doi: 10.1128/AEM.66.8.3376-3380.2000
- Davies, R. & Wales, A. (2019). Antimicrobial resistance on farms: a review including biosecurity and the potential role of disinfectants in resistance selection. Comprehensive Reviews in Food Science and Food Safety, 18, 753–774. doi: 10.1111/1541-4337.12438
- De Zwaan, R., Van Ingen, J. & Van Soolingen, D. (2014). Utility of rpoB gene sequencing for identification of nontuberculous mycobacteria in the Netherlands. Journal of Clinical Microbiology, 52, 2544–2551. doi: 10.1128/JCM.00233-14
- Elhamid, H., Lakany, H., Bekheet, A., Elbestawy, A. & Mataried, N. (2017). Pathogenicity of ten Gallibacterium anatis isolates in commercial broiler chickens. Alexandria Journal of Veterinary Sciences, 49, 42–49.
- Hall, T.A. (1999). Bioedit: a user-friendly biological sequence alignment editor and analysis program for Windows 95/98/NT. Nucleic Acids Symposium Series, 40, 95–98.
- Hasegawa, M., Kishino, H. & Yano, T.A. (1985). Dating of the human-ape splitting by a molecular clock of mitochondrial DNA. Journal of Molecular Evolution, 22, 160–174. doi: 10.1007/BF02101694
- Jordan, F.T.W., Williams, N.J., Wattret, A. & Jones, T. (2005). Observations on salpingitis, peritonitis and salpingoperitonitis in a layer breeder flock. Veterinary Record, 157, 573–577. doi: 10.1136/vr.157.19.573
- Khomtchouk, B.B., Hennessy, J.R. & Wahlestedt, C. (2017). Shinyheatmap: ultra fast low memory heatmap web interface for big data genomics. PLoS One, 12, 1–9. doi: 10.1371/journal.pone.0176334
- Kim, B.J., Lee, S.H., Lyu, M.A., Kim, S.J., Bai, G.H., Kim, S.J., Chae, G.T., Kim, E.C., Cha, C.Y. & Kook, Y.H. (1999). Identification of mycobacterial species by comparative sequence analysis of the RNA polymerase gene (rpoB). Journal of Clinical Microbiology, 37, 1714–1720. doi: 10.1128/JCM.37.6.1714-1720.1999
- Korczak, B., Christensen, H., Emler, S., Frey, J. & Kuhnert, P. (2004). Phylogeny of the family Pasteurellaceae based on rpoB sequences. International Journal of Systematic and Evolutionary Microbiology, 54, 1393–1399. doi: 10.1099/ijs.0.03043-0
- Kristensen, B.M., Frees, D. & Bojesen, A.M. (2011). Expression and secretion of the RTX-toxin GtxA among members of the genus Gallibacterium. Veterinary Microbiology, 153, 116–123. doi: 10.1016/j.vetmic.2011.05.019
- Kumar, S., Stecher, G., Li, M., Knyaz, C. & Tamura, K. (2018). MEGA x: molecular evolutionary genetics analysis across computing platforms. Molecular Biology and Evolution, 35, 1547–1549. doi: 10.1093/molbev/msy096
- Lawal, J.R., Ndahi, J.J., Bello, A.M., Wakil, Y., Dauda, J. & Ibrahim, U.I. (2017). Prevalence, isolation and antimicrobial susceptibility of Gallibacterium anatis from local breed of female Muscovy ducks (Cairina moschata) in Maiduguri, Northeastern Nigeria. Direct Research Journal of Veterinary Medicine and Animal Science, 2, 27–37.
- Mollet, C., Drancourt, M. & Raoult, D. (1997). rpoB sequence analysis as a novel basis for bacterial identification. Molecular Microbiology, 26, 1005–1011. doi: 10.1046/j.1365-2958.1997.6382009.x
- Neubauer, C., De Souza-Pilz, M., Bojesen, A.M., Bisgaard, M. & Hess, M. (2009). Tissue distribution of haemolytic Gallibacterium anatis isolates in laying birds with reproductive disorders. Avian Pathology, 38, 1–7. doi: 10.1080/03079450802577848
- Paudel, S., Alispahic, M., Liebhart, D., Hess, M. & Hess, C. (2013). Assessing pathogenicity of Gallibacterium anatis in a natural infection model: the respiratory and reproductive tracts of chickens are targets for bacterial colonization. Avian Pathology, 42, 527–535. doi: 10.1080/03079457.2013.843160
- Paudel, S., Hess, M. & Hess, C. (2017). Coinfection of Avibacterium paragallinarum and Gallibacterium anatis in specific-pathogen-free chickens complicates clinical signs of infectious Coryza, which can be prevented by vaccination. Avian Diseases, 61, 55–63. doi: 10.1637/11481-081016-Reg
- Paudel, S., Liebhart, D., Aurich, C., Hess, M. & Hess, C. (2014). Pathogenesis of Gallibacterium anatis in a natural infection model fulfils Koch’s postulates: 2. Epididymitis and decreased semen quality are the predominant effects in specific pathogen free cockerels. Avian Pathology, 43, 529–534. doi: 10.1080/03079457.2014.967176
- Paudel, S., Liebhart, D., Hess, M. & Hess, C. (2014). Pathogenesis of Gallibacterium anatis in a natural infection model fulfils Koch’s postulates: 1. Folliculitis and drop in egg production are the predominant effects in specific pathogen free layers. Avian Pathology, 43, 443–449. doi: 10.1080/03079457.2014.955782
- Persson, G. & Bojesen, A.M. (2015). Bacterial determinants of importance in the virulence of Gallibacterium anatis in poultry. Veterinary Research, 46, 1–11. doi: 10.1186/s13567-015-0206-z
- Renesto, P., Gouvernet, J., Drancourt, M., Roux, V. & Raoult, D. (2001). Use of rpoB gene analysis for detection and identification of Bartonella species. Journal of Clinical Microbiology, 39, 430–437. doi: 10.1128/JCM.39.2.430-437.2001
- Renesto, P., Lorvellec-Guillon, K., Drancourt, M. & Raoult, D. (2000). rpoB gene analysis as a novel strategy for identification of spirochetes from the genera borrelia, treponema, and leptospira. Journal of Clinical Microbiology, 38, 2200–2203. doi: 10.1128/JCM.38.6.2200-2203.2000
- Shaw, D.P., Cook, D.B., Maheswaran, S.K., Lindeman, C.J. & Halvorson, D.A. (1990). Pasteurella haemolytica as a co-pathogen in pullets and laying hens. Avian Diseases, 34, 1005–1008. doi: 10.2307/1591397
- Varan Singh, S. & Singh, B.R. (2015). Gallibacterium anatis: an emerging pathogen of poultry birds and domiciled birds. Journal of Veterinary Science & Technology, 7, 324–330. doi: 10.4172/2157-7579.1000324
- Wang, C., Robles, F., Ramirez, S., Riber, A.B. & Bojesen, A.M. (2016). Culture-independent identification and quantification of Gallibacterium anatis (G. anatis) by real-time quantitative PCR. Avian Pathology, 45, 538–544. doi: 10.1080/03079457.2016.1184743