ABSTRACT
This study presents a case of clubbed down syndrome in conventional broilers. During the first week of life, severe growth retardation was observed in approximately 25% of the flock. The growth-retarded chicks weighed only 45 g and showed a typical feather disorder which was most apparent on their abdomen and was defined in literature as typical for clubbed down syndrome. Necropsies, histology, biochemical analysis of blood and liver samples, serology and different PCR tests were performed in broilers to assess the aetiology of the clinical signs that were present in the affected broiler farm. Because of the suspicion of a possible link with the broiler-breeder farms, different investigations including serology, PCR and feed analysis were also performed on these farms. The results suggest that an accidentally excessive amount of calcium and iron in the feed of broiler-breeders, 3 weeks prior to first clinical signs in broilers, led to the development of clubbed down in the offspring, because of a relative Zn-deficiency in broiler-breeders and an absolute Zn-deficiency in the hatching eggs that were produced during this period. This appeared to be a reversible process as no clinical signs were observed in younger offspring of these broiler-breeders after they had consumed more of the new batch of feed. A potential involvement of Astrovirus could not be completely ruled out. This study demonstrates the importance of correct mineral concentrations in broiler-breeder feed and the impact it can have on the development of the offspring.
Introduction
Clubbed down (CD) syndrome was first described by Lepkovsky et al. (Citation1938) as a failure of the down feather follicle to rupture the surrounding sheath, causing the feather to coil and assume the appearance of a “French knot”. Since then, other researchers have given slightly different descriptions of CD (Edwards Brean, Citation1989). McClymont and Hart (Citation1947) described CD in chickens as: “a failure of the down filament to emerge through the skin, resulting in a small, yellow protuberance in the skin”. Dawson et al. (Citation1962) described a CD condition in turkeys as “bubble-shaped” feather follicles. Hawes (Citation1965) provided a slightly different description for CD in ducks, describing it as “buckling of the plumule within the sheath, forming a club-like bulge at the base of the shaft”. Haves and Fox (Citation1962) also correlated the occurrence of CD with genes for extended black pigmentation. Apparently, CD was a feature that was quite common in poultry before the 1980s; however today this feature is reported only sporadically (Pass, Citation1989; Whitehead et al., Citation1993) and it is not described in most recent poultry veterinary textbooks.
To date, CD syndrome has been considered to be of unknown aetiology, although various possible aetiologies have been suggested. Lepkovsky et al. (Citation1938) stated that a deficiency of riboflavin in the diet of hens caused the appearance of CD in the offspring, and the addition of riboflavin to the diet resulted in better hatchability and reduced the appearance of CD. However, Hawes and Fox (Citation1962) and Whitehead et al. (Citation1993) found that dietary supplementation of riboflavin increased hatchability, but did not reduce the incidence of CD. Despite this finding, CD syndrome is currently still considered to be mainly related to riboflavin deficiency (Moran, Citation2007). Dawson et al. (Citation1962) also associated CD in turkeys with a pantothenic acid deficiency. Possible involvement of avian astrovirus in the pathogenesis of CD has recently gained some attention, as avian astrovirus is known to be involved in runting-stunting syndrome in broilers. Unfortunately, the link with CD remains unclear and has never been proven experimentally (Smyth, Citation2017). During the 1980s in Australia a series of cases occurred with typical CD lesions of unknown aetiology to which no known infectious disease could be linked, nor were they caused by riboflavin deficiencies. In some of these cases, CD could be associated with a zinc deficiency and possibly also a manganese deficiency (Pass, Citation1989).
Mineral deficiencies can be induced in developing embryos when breeders are not correctly supplemented (Vieira, Citation2007). In most cases, egg production is not affected, but egg mineral content may be limiting the development of the embryo (Richards & Steele, Citation1987). Trace mineral deficiencies may lead to reduced growth rate, abnormal organ development, and embryonic death in severe cases (Savage, Citation1968). Trace mineral concentrations deposited in the eggs are variable and dependent on the quantity and chemical form present in the hens’ diet (Naber, Citation1979). Moreover, it has been shown that high dietary concentrations of certain minerals such as iron and calcium, or even available phosphorus, can have a strong influence on the uptake of trace minerals in the intestine (Shafety et al., Citation1991; Ammerman et al., Citation1995).
Zinc is considered to be an essential trace element and plays an essential role in a wide variety of biochemical processes. It is also a cofactor required for the function of over 300 different enzymes (Vallee & Auld, Citation1990; Tako et al., Citation2005). Zinc is also needed for the synthesis of nucleic acids and is considered as an important structural cofactor for many proteins (Vallee & Falchuk, Citation1993; Tako et al., Citation2005). Embryonic zinc deficiency induces proliferation arrest in many cell types, suppresses growth and causes congenital malformations that involve nearly all organ systems in offspring of zinc-deprived birds (Dreosti et al., Citation1968; Keen et al., Citation1989; Falchuk & Montorzi, Citation2001; Tako et al., Citation2005).
This paper describes a case of CD syndrome in conventional broilers associated with an embryonic zinc deficiency caused by an accidentally excessive amount of calcium and iron in the feed of the broiler-breeders.
Materials and methods
Case presentation
In June 2016, a single-house broiler farm in Belgium with 16,400 broilers contacted their farm veterinarian for a three-day-old Ross 308 broiler flock. At three days of age, there was no increase in mortality, but about 25% of the flock showed severe growth retardation. At 6 days of age these retarded broilers weighed only 45–50 g, while the weight of the unaffected birds was around 140 g. The affected birds also showed feather disorders (, and ).
Figure 1. Growth retarded (left) and unaffected (right) six-day-old broiler chicks. Photo: Tommy van Limbergen.
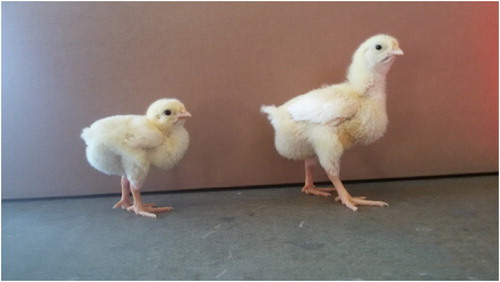
Figure 2. Six-day-old growth retarded broiler chicken with feather disorder at the wing. Photo: Tommy van Limbergen.
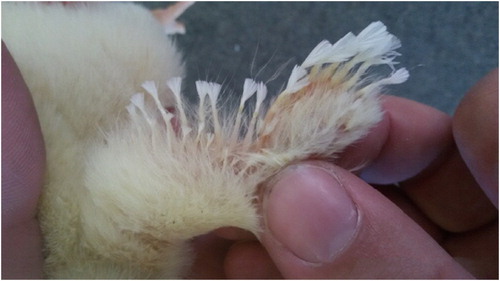
Figure 3. Growth retarded six-day-old broiler with feather disorder located at the breast, defined as clubbed down lesions. Photo: Tommy van Limbergen.
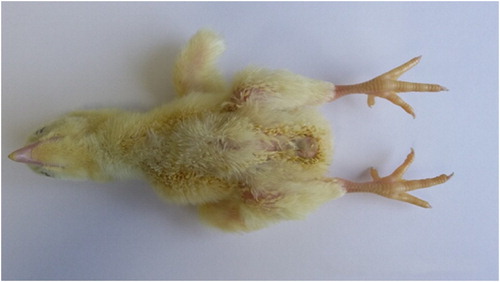
In the weeks that followed, several other broiler farms were found with similar problems. These flocks were traced down to their broiler-parents and all originated from two broiler-breeder farms. The first broiler-breeder farm (BB1) housed 54-week-old ROSS 308 broiler breeders, while the second broiler-breeder (BB2) farm housed 34-week-old Cobb broiler breeders. Since the link of clinical signs in broilers with the broiler-breeder farms BB1 and BB2 was discovered, the production of hatching eggs from these flocks was stopped. The birds of BB1 were slaughtered, while in BB2, a small sample of eggs was incubated every week to monitor the presence of retarded chicks. During the subsequent weeks, the presence of retarded chicks originating from BB2 decreased from approximately 25% to 3% by 4 weeks later.
Clinical signs
The one-day-old chicks showed no obvious clinical signs on the day of placement, but a subset of chicks (about 25% in the first case-farm) showed typical feather disorders which were most apparent on the wings () and in the breast region (). These feather disorders were similar to what has been described in literature as “clubbed down” (Lepkovsky et al., Citation1938): the feathers were coiled and took the shape of a French knot, resulting from a failure of the feather to rupture the surrounding sheath. Besides the feather disorders, about 5% of the affected one-day-old chicks were also found with curled toes. At necropsy of affected one-day-old chicks, no additional abnormalities were found.
Three days after placement, the flock became more heterogeneous because of the lack of growth of the affected birds (). At this stage, no rise in mortality was present, although there was an increased culling rate, resulting in overall first-week mortality of 4.05%.
These clinical problems persisted during the remainder of the cycle and the farmer was advised to cull the affected chicks as much as possible. The overall mortality rate (including culled broilers) of the flock was 7.40%. An overview of the performance parameters of the affected flock and one flock before and after at the same broiler farm is shown in .
Table 1. Performance parameters of three successive flocks in the same broiler farm where first chicks with clubbed down syndrome were found (i.e. affected flock).
The broiler-breeders did not show any clinical signs nor any change in weekly performance parameters in BB1. In BB2, all performance parameters were considered to be normal, except for a slight decrease in hatchability between 31 and 33 weeks of age. During this period, hatchability dropped from 82.9% at 30 weeks of age to 79.8% at 31 weeks, 79.5% at 32 weeks and 76.8% at 33 weeks of age. At 34 weeks of age, hatchability increased again to 83.7% and followed a normal curve during the remainder of the cycle. An overview of the main performance parameters of BB1 and BB2 is provided in . Both broiler-breeder farms were located in Belgium and operated following standard practices in producing hatching eggs. Both farms received the same feed formulation from the same feed company. Unfortunately, details of this feed formulation were not made available, but the feed formulation was based on the breeder nutritional guidelines (Aviagen, Citation2016).
Table 2. Farm characteristics and performance parameters of broiler-breeder farms.
Investigations in broilers
Twelve chicks (offspring from BB2) were necropsied at 1 and at 6 days of age at the department of Pathology, Bacteriology and Poultry Diseases, Faculty of Veterinary Medicine (Ghent, Belgium). Samples for histological examination were taken, stained with haematoxylin and eosin and examined.
Blood samples from 15 affected and 15 unaffected one-day-old broilers (offspring from BB2) were collected and tested by indirect ELISA (AniCon Labor GmbH, Höltinghausen, Germany) for the presence of antibodies against avian encephalitis virus (AEV), avian reovirus (ARV), chicken infectious anaemia virus (CIAV), fowl adenovirus (FAV) and chicken astrovirus group B (CAstV). The same blood samples were pooled and tested for AEV, ARV, CIAV and FAV by real-time (RT) PCR and agarose gel electrophoresis (AniCon Labor GmbH) and CAstV by RT-PCR.
Blood samples from another 30 affected and 30 unaffected one-day-old broilers (offspring from BB2) were collected and tested for biochemical parameters by spectrophotometry (Velab nv., Herentals, Belgium) to measure the concentrations of alkaline phosphatase (AP), creatine phosphokinase (CPK) and lactate dehydrogenase (LDH).
Liver samples were taken from 10 one-day-old chicks (offspring from BB2, five affected one-day-old chicks and five unaffected) and five unaffected one-day-old broilers from another broiler-breeder flock (offspring from broiler-breeders of the same age and breed as those present in BB2) to measure the concentration of zinc in these liver samples by spectrophotometry (ECCA nv., Merelbeke, Belgium). The concentration of superoxide dismutase (SOD) was measured (using spectrophotometry (RP Lab, Nivelles, Belgium)) in 10 blood samples from one-day-old chicks originating from BB2 and in 10 blood samples originating from one-day-old chicks (offspring from broiler-breeders, same breed and age as BB2 without CD-problems).
Investigations in broiler-breeders (including hatching eggs)
The performance parameters of the broiler-breeder flocks were investigated to detect potential abnormalities.
A PCR (MSD Animal Health, Brussels, Belgium) was performed on 10 cloacal and tracheal swabs to detect the presence of infectious bronchitis virus (IBV) in BB1. This was performed because, at that time, clinical problems of IB due to strain QX, were present in other farms in the same region where BB1 was located.
Paired blood samples were taken from 15 broiler-breeder hens in BB2 in order to detect the levels of AEV, ARV, CIAV, FAV and CAstV by indirect ELISA (AniCon Labor GmbH). Pre-samples were present from routine sampling procedures and were taken at 23 weeks of age. Post-samples were taken at 34 weeks of age.
For BB2 a break-out at hatching was also performed to inspect the eggs that did not hatch and to determine the cause of not hatching.
Feed samples (n = 3) from BB1 house 2 were chemically analyzed (Labo Vanden Avenne – Ooigem nv., Ooigem, Belgium) from three batches of feed delivered for birds between 24 and 28 weeks of age. A wheat-based feed was used in broiler-breeders. Unfortunately, more information about feed formulation was not shared by the feed manufacturer, apart from the information that the feed formulation (including additives) was not changed over the subsequent feed deliveries.
Results
Broilers – necropsy findings
One-day-old chicks with clinical signs of CD showed no other abnormalities at necropsy, besides a high amount of cervical sub-cutaneous oedema. Histological investigation of these oedematous regions showed that this was most likely due to inflammation; however, no aetiological diagnosis could be established.
Necropsy of six-day-old chicks revealed no abnormalities, besides the apparent small stature, feather disorders and empty gastrointestinal tract. Histological examination of the skin showed the presence of apoptotic cells in the sheaths of the feather follicles, and swollen hepatocytes. There were no lesions in the thyroid gland.
Broilers – PCR and serological findings
An overview of serological results for broilers is provided as supplementary data. Seropositive samples were present for all investigated agents varying from all samples positive (n = 15) to one single positive sample. No clear differences were present between affected and unaffected broilers for AEV, ARV, CIAV, FAV and CAstV antibodies.
Real-time PCR did not detect AEV, ARV, CIAV and FAV in pooled blood samples from affected and unaffected one-day-old broilers. However, CAstV could be detected in pooled samples from affected one-day-old chicks, but not in samples from unaffected one-day-old chicks.
Broilers – biochemical findings
Biochemical analysis of blood- and liver samples showed some differences between affected and unaffected one-day-old broilers (supplementary data). Average AP concentration was significantly lower in blood samples from affected broilers compared to unaffected broilers (P < 0.01), namely 1143 vs. 2157 units per litre (U/l). Average creatine phosphokinase (CPK) and lactate dehydrogenase (LDH) concentrations in blood samples were significantly higher (P < 0.01 and P < 0.05, resp.) in affected broilers (i.e. 8592 and 2993 U/l, resp.) when compared to unaffected broilers (i.e. 6626 and 1683 U/l, resp.). The SOD concentration in blood samples was not significantly different (P > 0.05) between affected and unaffected samples originating from BB2, i.e. 872.7 vs. 873.7 units per gram haemoglobin (U/g HB). The concentrations of Zinc (Zn) in liver samples of one-day-old broilers originating from BB2 were significantly lower in affected broilers compared to unaffected broilers (). The average Zn-concentration in liver samples was 9.4 mg/kg in affected broilers and 11.6 mg/kg in unaffected broilers. The average Zn-concentration in liver samples from one-day-old chicks originating from BB2 was significantly (P < 0.05) lower (i.e. 10.5 mg/kg) compared to unaffected one-day-old broilers from another flock of broiler-breeders (i.e. 18.4 mg/kg) ().
Table 3. Zn-concentration (mg/kg) in liver samples from one-day-old chicks originating from BB2 and similar unaffected broiler-breeders (control).
Broiler-breeders – PCR and serological findings
PCR-analysis of cloacal and tracheal swabs did not show the presence of IB virus from samples of BB1.
An overview of the serological results by indirect ELISA for BB2 houses one and two is provided as supplementary data. Positive samples were present for all investigated diseases varying from all samples positive (n = 15) to 3 out of 15 samples positive.
In BB2 house one (clinical problems of CD in offspring) all pre- and post-samples (n = 15) were positive for ARV, CIAV and FAV. Antibody-titres for CIAV were similar in pre- and post-samples, while titres for ARV were lower in post-samples in comparison to pre-samples (i.e. 12479 vs. 15612, resp.). There was a clear increase in titres for FAV, the values in post- samples were approximately 2.5 times higher than in pre-samples (i.e. 14854 vs. 6218, respectively). For AEV, both the number of positive samples and the average titres decreased in time. During pre-sampling, 12 out of 15 samples were positive (average titre: 4664) and 10 weeks later this were only 7 out of 15 samples (average titre: 3262). The number of positive samples for CAstV declined from 8 out of 15 to 3 out of 15 samples, also the average titres declined from 1095 to 558.
In BB2 house two (no clinical problems of CD in the offspring) all pre- and post-samples (n = 15) were positive for CAstV, CIAV and FAV. Antibody-titres for CAstV and CIAV were similar in pre- and post-samples. For FAV, the average antibody titre increased from 5075 to 14,901. The number of positive samples for AEV increased from 11 out of 15 to 13 out of 15 samples. The average antibody titre for AEV increased from 4102 to 6700. For CAstV, an equal number of positive samples was present in pre- and post-samples, i.e. five out of 15 samples. The average antibody titre for CAstV was similar in pre- and post-samples.
Broiler-breeders – feed analysis results
Feed samples 1 and 3, delivered to birds at 24 and 28 weeks of age (see ) showed no irregularities in comparison to the nutritional reference values (see (Aviagen, Citation2016)). The feed that was provided at 26 weeks of age (feed sample 2) contained a lower amount of crude protein, fat, carbohydrates, fibres, NaCl, phosphorus, potassium and zinc, whereas an excessive amount of total ash (24.40% instead of ±12%), calcium (9.53% instead of ±3.50%) and iron (918 mg/kg instead of ±155 mg/kg) were present. Unfortunately, no detailed information was provided about formulation of the broiler-breeder feed.
Table 4. Overview of feed components in broiler-breeder feed samples (BB1).
Table 5. Overview of the nutritional reference values for broiler-breeder feed with respect to crude protein, minerals and added trace minerals (Aviagen, Citation2016).
Broiler-breeders – hatching egg investigation
For BB2 an investigation of 82 unhatched hatching eggs was performed. Cervical oedema was present in 39 eggs. The second most common finding (15 eggs) were malformations such as supplementary legs and conjoined twins. Almost 10% of the unhatched eggs (i.e. eight eggs) showed internal pipping and six eggs showed external pipping. CD syndrome was present in seven of the unhatched hatching eggs. A total of 6 hatching eggs appeared to be non-fertilized and 13 of the unhatched hatching eggs were in a decomposed state.
Discussion
In this study, we report growth retardation and feather disorders typical for CD syndrome. As a clear aetiology for CD could not be established, a broad series of investigations was performed.
Growth retardation in broilers is a clinical feature that can be caused by numerous factors, including non-infectious and infectious (Morrow et al., Citation2008; Aviagen, Citation2010). In the current case, the effect of growth retardation was already seen at 3 days of age. In the event of a single case only showing growth retardation on one farm, the primary reason would most likely be linked to problems in the management of the young broilers. In the described case, the farm veterinarian performed a broad investigation and found no abnormalities related to the accessibility of feed and water, climatic conditions and light administration (not shown in results). Because this case appeared to be not the only one at that time, and similar clinical findings in other farms with different broiler feed manufacturers were found, the focus of the investigation was not on management factors.
All farms with clinical signs of CD syndrome were found to be linked to two broiler-breeder farms. It has been reported in the literature that a variety of infectious and non-infectious factors in broiler breeders can affect the health and performance of their progeny (Glavits et al., Citation1984; Giovanardi et al., Citation2005). These include vertical transmission of bacterial diseases such as avian pathogenic Escherichia coli (Giovanardi et al., Citation2005) and Staphylococcus aureus (Glavits et al., Citation1984). During the investigation of this case, a primary bacterial cause originating from broiler breeders tended to be most unlikely as no signs of bacterial infection were found during necropsies.
Serological investigation of pre- and post-sera from broiler-breeders (BB2) revealed all samples were positive for ARV, CIAV and FAV. There was an increase in titres for FAV post-sera in both investigated houses of BB2. All sera from broilers were positive for FAV, while titres were the same in broilers with or without clinical signs of CD, and it was not possible to detect virus in any of the investigated broilers. A relationship between FAV and growth retardation and/or feather disorders in broilers during the first days post-hatch is not reported in the literature and the data from this case also suggest no relationship between the clinical signs and involvement of FAV.
For ARV and CIAV, virus was not detected in samples from broilers, while serology was positive for all broiler-breeder samples and most broiler samples. The presence of antibodies to ARV and CIAV was due to maternal vaccination and transfer of maternal antibodies to their progeny (De Herdt et al., Citation1999; Sommer & Cardona, Citation2003). ARV is sometimes linked to Runting-Stunting Syndrome (RSS) in broilers. RSS in broilers is a transmissible disease with an unknown pathogenesis (Kang et al. Citation2012). RSS affects broilers early post-hatch and is specifically characterized by growth retardation, ruffled feathers, and diarrhoea. The current case differs from RSS in that no diarrhoea was observed and the feather disorders were very specific for what was previously described as CD syndrome (Lepkovsky et al. Citation1938); these specific feather disorders were never described in relation to RSS.
AEV infection is known to cause a slight reduction in egg production in susceptible hens and can also be transmitted to embryos (McNulty et al., Citation1990; Marvil et al., Citation1999). Clinical signs of an AEV-infection include reduced hatching and characteristic tremors and/or signs of ataxia in affected broilers between 1 and 7 days of age (Marvil et al., Citation1999). Although a slight decrease in hatchability occurred in broiler-breeders in BB2 at the time that clinical signs were found in broilers, AEV was not detected in samples from affected- and non-affected broiler chicks. Also, the AEV-typical clinical signs were absent in broilers. Furthermore, AEV titres in broiler breeders were present, but did not show a clear increase in either of the broiler-breeder houses (BB2). AEV-titres were higher in unaffected broilers in comparison to broilers with clinical signs of CD syndrome. These data indicate that the problem was probably not due to AEV.
CAstV infections are common in poultry farms. A serological investigation in UK demonstrated that 10 out of 10 tested broiler flocks were positive and 78% of the investigated broiler samples had CAstV antibodies (Todd et al., Citation2009). For broiler-breeders, more than 90% of investigated flocks were positive, and 53% of the investigated samples from broiler breeders had antibodies, which is much higher than what was found in the present study. CAstV was also present in grand parent and great grand-parent flocks (Todd et al., Citation2009). CAstV was suggested to be linked to RSS, White Chicks Hatchery disease, and to growth retardation in young SPF broilers (McNulty et al., Citation1990; McNeilly et al., Citation1994; Smyth, Citation2017). It has also been suggested to be involved in the pathogenesis of CD syndrome, although this link remains unclear (Hammond, Citation2014; Smyth, Citation2017). In the present case, CAstV was detected in pooled samples from affected one-day-old chicks, while no virus was present in samples from unaffected one-day-old chicks. This might suggest a possible involvement in the pathogenesis of CD syndrome, although this might also be coincidental since this result originates only from one sampled flock. CAstV titres were higher in affected one-day-old chicks in comparison to non-affected chicks, but only a limited number (i.e. 3 out of 30) of one-day-old chicks had CAstV antibodies. This low maternal protection might also contribute to the hypothesis that CAstV might be involved by vertical transmission in the clinical appearance of CD. The relationship between CAstV and CD has not been proven in the literature, but certain types of CAstV are known to spread vertically. Sajewicz-Krukowska et al. (Citation2016) described a 30-week-old broiler-breeder flock in Poland where a 4–5% hatchability decrease was observed over a 4-week period when, at the same time, a maximum of 1% of chicks was pale and weak. The Polish and our current observations are indicative of a vertical virus transmission. Smyth et al. (Citation2013) reported that Finnish breeder flocks affected by White Chicks Hatchery disease only experienced the disease once during their lifetime. It thereby seems probable that acquired immunity prevents disease recurrence and further vertical transmission (Smyth et al., Citation2017). Given the complexity of CAstV epidemiology and its involvement in similar disease patterns that include growth retardation, we are not able to exclude CAstV as a potential cause for this case of CD. However, given the fact that CD cases are rare and CAstV-infections are quite common, we suggest that this case was not primarily caused by CAstV, although we cannot rule out that it is involved in the pathogenesis of CD.
Each type of broiler-breeders has specific nutrition management guidelines (Aviagen, Citation2016). The comparison of the advised ROSS 308 broiler-breeder nutritional specifications with the composition of the feed provided to the broiler breeders of BB1 at the time that clinical signs were found in their offspring, showed a clear excess in the concentration of calcium (three times too high) and iron (six times too high), due to an excess of crushed oyster shells (presumably by accident). It has been shown that an excess in dietary calcium can lead to signs of zinc deficiency in swine and poultry and that this adverse effect of an excess in dietary calcium can be overcome by supplementing dietary zinc (Morrison & Sarett, Citation1958; O’Dell et al., Citation1958; Roberson & Schaible, Citation1960; Kienholz et al., Citation1961; Faria et al., Citation2020). No clear signs of a zinc deficiency were observed in broiler-breeders related to the present case. This could be due to the fact that this excess in calcium was only present in one feed delivery, and that 6 days later new feed with normal levels of calcium was delivered, although this does not exclude the possibility that this temporal excess induced clinical Zn deficiency in the offspring. Berg et al. (Citation1962) were able to temporarily induce a feather disorder, i.e. an abnormal wing feather condition, in the offspring of hens receiving a feed with high calcium concentrations. The feather disorder disappeared when the hens or the chicks were supplemented with dietary zinc. Zinc deficiency signs were most evident in the chicks hatched from the first eggs laid. The severity of deficiency signs decreased with each successive hatch, being practically absent after the pullet breeders had been in lay for six weeks. In the present case, the broiler-breeder feed contained excess calcium while the zinc level was very low (i.e. 70 mg/kg) compared to broiler-breeder guidelines (i.e. 110 mg/kg, Aviagen, Citation2016). This low level of dietary zinc could have potentiated the Zn deficiency that was caused by the excess in calcium.
It has been demonstrated that a high calcium diet: (1) increases the pH of crop and ileum contents; (2) reduces the solubility of minerals (i.e. iron, magnesium and zinc) in the gastrointestinal tract and increases the size of mineral complexes during their passage from duodenum to ileum; and (3) reduces the proportion of soluble zinc associated with small complexes. Moreover, high calcium and available phosphorus diets reduce the proportions of soluble magnesium and zinc associated with small complexes (Shafety et al., Citation1991; Guinotte et al., Citation1995; Powell et al., Citation2011).
High dietary concentrations of iron (800–1600 ppm) have been shown to negatively affect mortality and feed intake, mainly in young birds (van Ravenswaay, Citation1986, Cao et al., Citation1996). The broiler-breeders in the current case were 35 weeks old and were fed with 918 ppm iron in the feed during a period of at least 6 days. No toxicity was detected in broiler-breeders as a consequence of this high iron feed. This might be due to an age-related reduced iron absorption (Forbes & Reina, Citation1972), and also the high dietary calcium levels that were present at the same time could reduce the negative effects of high dietary iron by reducing the solubility of minerals and increasing the size of mineral complexes in the gut (Shafety et al., Citation1991). Moreover, the bioavailability of iron is dependent on the source of iron that is provided to the birds (Fairweather-Tait, Citation1987). Differences in bioavailability depending on the source of mineral have been shown for manganese, zinc, selenium and copper (Ammerman et al., Citation1995), but for high concentration iron diets no difference in bioavailability by source of iron could be demonstrated (Cao et al., Citation1996). Solomons and Jacob (Citation1981) found that high doses of inorganic iron decrease zinc uptake as measured by changes in plasma zinc over the next 4 h after an oral dosage of iron. These findings indicate that the high dietary iron content in the present case could also induce lower uptake of zinc in broiler breeders.
A deficiency in crude protein (CP) was also noticed, i.e. 11% instead of 13%. Lopez and Leeson (Citation1995) showed that reducing the CP level (with constant lysine and methionine levels) to 10% in broiler-breeder feed had no effect on the number of eggs produced; however, the eggs were smaller and lighter and chicks were smaller at hatch. Although the latter was not noted in the present case, we cannot completely exclude a possible involvement of the lower CP level. In addition to the potential effects due to the lower level in CP, the excess in dietary calcium could also worsen the case by negatively influencing phytase efficacy, which could also have a negative influence on protein digestion in the gizzard and subsequent amino acid digestibility (Cowieson & Ravindran, Citation2007; Walk et al., Citation2012).
Our case included growth retardation and feather disorders in broilers. We hypothesize that this was caused by the imbalanced trace elements (i.e. excess in calcium and iron) in the feed provided to the broiler breeders, leading to the production of eggs, embryos and one-day-old chicks with trace mineral deficiencies (i.e. zinc). Mineral deficiencies can be induced quickly in developing embryos, when breeders are not correctly supplemented (Vieira, Citation2007). Mineral deficiencies in broiler-breeder feed do not necessarily affect egg production, but the eggs that are produced can be depleted in trace minerals (Richards & Steele, Citation1987). Deficiencies of trace minerals in developing embryos can cause impaired growth, abnormal development of all the major organ systems and, in extreme deficiencies, also embryonic death (Savage, Citation1968; Richards & Steele, Citation1987; Richards, Citation1997). Excessive amounts of trace minerals have also been shown to damage the developing embryo, leading to embryotoxic and teratogenic effects (Richards, Citation1997). Although the broiler breeders in this case were accidentally fed with six times the normal amount of iron, it was shown before that hens fed with an excessive amount of iron do not produce eggs with an increased level of iron (Richards, Citation1997). This supports our hypothesis that the clinical findings are due to deficiencies in the egg caused by the excessive amount of calcium in the feed and that the excessive amount of iron in the feed had no major impact on the levels of iron in the eggs. Up to 91.8% of the egg zinc storage is located in the yolk, of which more than 90% is bound to lipovitellin and phosvitin (Tupper et al., Citation1954; Richards, Citation1991). Zinc is transported in the plasma to the ovary by an oestrogen-induced yolk precursor protein, vitellogenin, which binds trace metals (Richards, Citation1989). Mitchell and Carlisle (Citation1991) suggested that plasma zinc levels can be indirect indicators of vitellogenin levels in the plasma. This supports our hypothesis that a calcium-induced zinc deficiency in hens could lead to a lower amount of zinc being stored in the yolk of their eggs (not investigated). During the chick’s embryonic development, concentrations of zinc, copper, iron and manganese decrease (Dewar et al., Citation1974; Richards & Weinland, Citation1985; Richards, Citation1997; Yair & Uni, Citation2011). Most of the available minerals are consumed by embryonic day 17 (E17) (Yair & Uni, Citation2011); in the case of zinc this is up to 94.93% of the amount available in the yolk. Between day of setting and embryonic day 11 (E11) there is moderate to mild consumption of iron, zinc, copper and manganese (Yair & Uni, Citation2011). There is increased consumption between E11 and E17. However, from E17 to the day of hatch, there is almost no consumption of iron, zinc, copper, and manganese. These changes indicate either mineral limitation or limited mineral demand during the last 4 days of incubation. Given the fact that these minerals are important for the development of the cardiovascular, immune, and skeletal systems, it is reasonable to assume that low levels and minimal consumption can impair the developmental potential and performance of chicks (Yair & Uni, Citation2011).
We measured the concentration of zinc in the livers of one-day-old chicks. The offspring of BB2 were found to have only half of the concentration of zinc in their liver in comparison to one-day-old chicks that originated from broiler-breeders of similar age that received a balanced feed. At slaughter age, the liver of broilers contains 33.89 mg/kg zinc (Majewska et al., Citation2016), which is two times the level that we found in healthy one-day-old chicks, suggesting that healthy chicks hatch with an already, physiological normal, low level of zinc. This strongly supports our hypothesis that the severe growth retardation and feather disorders were caused by a maternal zinc deficiency due to an unbalanced feed. Not all chicks that originated from BB2 showed typical lesions. Chicks with clinical signs had a lower level of zinc in the liver, up to almost 2 mg/kg, in comparison with chicks that showed no clinical signs. The latter might suggest a critical threshold value in zinc concentrations available to the one-day-old chick and when this is not reached, clinical signs of growth retardation and feather disorders may arise. This hypothesis is also supported by the decreased levels of AP in chicks with clinical signs when compared to chicks that showed no signs. AP contains four zinc ions per molecule, and zinc plays a crucial role in its mode of action as the substrates of AP are positioned between zinc ions in the active sites of the enzyme. A sufficient level of zinc must therefore be available for zinc transporters to load this essential component into enzymes like AP (Coleman, Citation1992; Suzuki et al., Citation2004). LDH levels in chicks with clinical signs were almost double the LDH levels in chicks without clinical signs. Although a direct link between zinc deficiency and high LDH levels in poultry has not been reported in the literature, Gerson and Meyer (Citation1977) showed an increased level of LDH activity in buccal epithelium of zinc-deficient rats. Zinc is an important factor of the antioxidant enzyme copper–zinc SOD, which helps defend the body by converting superoxide anions into hydrogen peroxide (deHaan et al., Citation1994). We analysed SOD from one-day-old chicks with and without clinical signs originating from BB2, but no clear difference was found. The latter could be due to the possibility that SOD also binds copper, and SOD is thereby not specific enough to use in the diagnosis of zinc deficiency in one-day-old chicks (Wilson et al., Citation1992).
Previous authors were able to induce feather disorders as described in our case by feeding an unbalanced feed to parent stock (Kienholz et al., Citation1961; Berg et al., Citation1962). The specific clinical feature, CD, was at its first description by Lepkovsky et al. (Citation1938) linked to riboflavin deficiency. In our case, riboflavin was supplemented to broiler-breeders immediately after the first chicks hatched with typical CD lesions. Supplementation, however, did not have any effect and chicks continued to hatch with typical CD lesions. In Australia during the 1980s a series of comparable cases occurred with typical CD lesions of unknown aetiology to which no known infectious disease could be linked, and there was also no link with riboflavin deficiencies (Pass, Citation1989). These outbreaks in Australia also showed a reduced serum AP activity in plasma of newly hatched affected chicks in comparison with the normal chicks. Reduced AP activity was believed to be associated with zinc and possibly manganese deficiency in some outbreaks (Pass, Citation1989).
Growth retardation due to zinc deficiency is observed often in many animal species (including humans), most likely because of impairment of nucleic acid biosynthesis and amino acid utilization or protein synthesis (O’Dell et al., Citation1958; Young et al., Citation1958; Salim et al., Citation2008). Zinc is a key element in the synthesis of proteins essential for growth (Golden & Golden, Citation1992). Insufficient quantities of zinc during embryogenesis may therefore influence the final phenotype of all organs (Terrin et al., Citation2015).
In conclusion, the results of these different analyses suggest that this outbreak of CD syndrome was caused by a nutritional error that occurred in the feed of the broiler-breeders, 3 weeks prior to the first clinical signs in broilers. We suspect that the excessive amount of calcium and iron led to the development of CD in the offspring in this particular case of CD in broilers, because of an absolute Zn-deficiency in the livers of the one-day-old chicks that were produced during this period. This appeared to be a reversible process as no clinical signs were observed in younger offspring of these broiler-breeders after the latter had consumed more of the new balanced feed.
More studies, preferably under experimental conditions, are needed to confirm the findings of the present case study.
Supplemental Material
Download MS Word (12.7 KB)Supplemental Material
Download MS Word (15.6 KB)Acknowledgements
The authors are grateful to the owners of the broilers and broiler-breeders for their help during the investigation of this case and for furnishing the production records, and to Dr M. Claeskens for carrying out some of the samplings.
Disclosure statement
No potential conflict of interest was reported by the author(s).
References
- Ammerman, C.B., Baker, D.H. & Lewis, A.J. (1995). Bioavailability of Nutrients for Animals: Amino Acids, Minerals, and Vitamins. San Diego, CA: Academic Press.
- Aviagen. (1999). Ross Breeders Broiler Management Manual. Newbridge: Aviagen. Midlothian, Scotland.
- Aviagen. (2010). Ross Environmental Management in the Broiler House. Newbridge: Aviagen. Midlothian, Scotland.
- Aviagen. (2016). Ross 308 Parent Stock: Nutrition Specifications. Newbridge: Aviagen. Midlothian, Scotland.
- Berg, L.R., Bearse, G.E. & Merrill, L.H. (1962). Evidence for a high zinc requirement at the onset of egg production. Poultry Science, 41, 703–707.
- Cao, J., Luo, X.G., Henry, P.R., Ammerman, C.B., Littell, R.C. & Miles, R.D. (1996). Effect of dietary iron concentration, age, and length of iron feeding on feed intake and tissue iron concentration of broiler chicks for use as a bioassay of supplemental iron sources. Poultry Science, 75, 495–504.
- Coleman, J.E. (1992). Structure and mechanism of alkaline phosphatase. Annual Review of Biophysics and Biomolecular Structure, 21, 441–483.
- Cowieson, A.J. & Ravindran, V. (2007). Effect of phytic acid and microbial phytase on the flow and amino acid composition of endogenous protein at the terminal ileum of growing broiler chickens. British Journal for Nutrition, 98, 745–752.
- Dawson, E., Ferguson, T.M., Deyoe, C.W. & Court, J.R. (1962). Pantothenic acid deficient turkey embryos. Poultry Science, 41, 1639.
- deHaan, J.B., Tymms, M.J., Cristiano, F. & Kola, I. (1994). Expression of copper/zinc superoxide dismutase and glutathione peroxidase in organs of developing mouse embryos, fetuses, and neonates. Pediatric Research, 35, 188–196.
- De Herdt, P., Ducatelle, R., Uyttebroek, E., Hermans, J., Sneep, A. & Torbeyns, R. (1999). Reovirus serology in broiler parents and their progeny and its correlation with performance. Avian Diseases, 43, 271–278.
- Dewar, W.A., Teague, P.W. & Downie, J.N. (1974). The transfer of minerals from the egg to the chick embryo from the 5th to 18th days of incubation. British Poultry Science, 15, 119–129.
- Dreosti, I.E., Tao, S.H. & Hurley, L.S. (1968). Plasma zinc and leukocyte changes in weaning and pregnant rats during zinc deficiency. Proceedings of the Society for Experimental Biological Medicine, 128, 169–174.
- Edwards Brean, S. (1989). Dietary riboflavin and the production of clubbed down in chick embryo. Thesis for the degree of Master of Science in Poultry Science. Oregon State University, USA.
- Falchuk, K.H. & Montorzi, M. (2001). Zinc physiology and biochemistry in oocytes and embryos. Biometals, 14, 385–395.
- Fairweather-Tait, S.J. (1987). The concept of bioavailability as it relates to iron. Nutrional Research, 7, 319–325.
- Faria, B.D., Silva, L.M., Junior, V.R., do Nascimento Ferreira, A.H., Rostagno, H.S., Teixeira, A.L.F. & Hannas, M.I. (2020). Organic trace minerals and calcium levels in broilers’ diets to 21 days old. Scientific Agriculture, 77, e20180071.
- Forbes, G.B. & Reina, J.C. (1972). Effect of age on gastrointestinal absorption (Fe, Sr, Pb) in the rat. Journal of Nutrition, 102, 647–652.
- Gerson, S.J. & Meyer, J. (1977). Increased lactate dehydrogenase activity in buccal epithelium of zinc-deficient rats. The Journal of Nutrition, 107, 724–729.
- Giovanardi, D., Campagnari, E., Sperati Ruffoni, L., Pesente, P., Ortaliand, G. & Furlattini, V. (2005). Avian pathogenic Escherichia coli transmission from broiler breeders to their progeny in an integrated poultry production chain. Avian Pathology, 34, 313–318.
- Glavits, R., Ratz, F., Fehervari, T. & Povazsan, J. (1984). Pathological studies in chicken embryos and day-old chicks experimentally infected with Salmonella typhimurium and Staphylococcus aureus. Acta Veterinaria Hungarica, 32, 39–49.
- Golden, B.E. & Golden, M.H. (1992). Effect of zinc on lean tissue synthesis during recovery from malnutrition. European Journal of Clincal Nutrition, 46, 697–706.
- Guinotte, F., Gautron, J., Nys, Y. & Soumarmon, A. (1995). Calcium solubilization and retention in the gastrointestinal tract in chicks (Gallus domesticus) as a function of gastric acid secretion inhibition and of calcium carbonate particle size. British Journal for Nutrition, 73, 125–139.
- Hammond, P.P. (2014, 8 April). New diseases or re-emerging threats: current knowledge state - avian reoviruses and clubbed down syndrome. Proceedings SWCA 8th April 2014, 25, 1–33.
- Hawes, R.O. (1965). Association of clubbed down and black down-color in ducks. Journal of Heredity, 56, 116–118.
- Hawes, R.O. & Fox, T.W. (1962). The influence of riboflavin and extended black pigmentation of defective down condition in the domestic fowl. Poultry Science, 41, 1504–1508.
- Kang, K.I., El-Gazzar, M., Sellers, H.S., Dorea, F., Williams, S.M., Kim, T., Collett, S. & Mundt, E. (2012). Investigation into the aetiology of runting and stunting syndrome in chickens. Avian Pathology, 41, 41–50.
- Keen, C.L., Peters, J.M. & Hurley, L.S. (1989). The effect of valproic acid on 65Zn distribution in the pregnant rat. Journal of Nutrition, 119, 607–611.
- Kienholz, E.W., Turk, D.E., Sunde, M.L. & Hoekstra, W.G. (1961). Effects of zinc deficiency in the diets of hens. Journal of Nutrition, 75, 211–221.
- Lepkovsky, S., Taylor, L.W., Jukes, T.H. & Almquist, H.J. (1938). The effect of riboflavin and the filtrate factor on egg production and hatchability. Hilgardia, 11, 559–591.
- Lopez, G. & Leeson, S. (1995). Response of broiler breeders to low protein diets: adult breeder performance. Poultry Science, 74, 685–695.
- Majewska, D., Szczerbińska, D., Ligocki, M., Bucław, M., Sammel, A., Tarasewicz, Z., Romaniszyn, K. & Majewski, J. (2016). Comparison of the mineral and fatty acid profiles of ostrich, turkey and broiler chicken livers. British Poultry Science, 57, 193–200.
- Marvil, P., Knowles, N.J., Mockett, A.P., Britton, P., Brown, T.D.K. & Cavanagh, D. (1999). Avian encephalomyelitis virus is a picornavirus and is most closely related to hepatitis A virus. Journal of General Virology, 80, 653–662.
- McClymont, G.L. & Hart, L. (1947). Studies on nutrition of poultry. 1. Investigations of the inadequacy of high wheat content rations for hatchability in White Leghorn pullets. Australian Veterinary Journal, 23, 247–253.
- McNeilly, F., Connor, T.J., Calvert, V.M., Smyth, J.A., Curran, W.L. & Morley, A.J. (1994). Studies on a new enterovirus-like virus isolated from chickens. Avian Pathology, 23, 313–327.
- McNulty, M.S., Connor, T.J., McNeilly, F. & McFerran, J.B. (1990). Biological characterization of avian enteroviruses and enterovirus-like viruses. Avian Pathology, 19, 75–87.
- Mitchell, M.A. & Carlisle, A.J. (1991). Plasma zinc as an index for vitellogenin production and reproductive status in the domestic fowl. Comparative Biochemistry and Physiology, 100, 719–724.
- Moran, E.T. (2007). Nutrition of the developing embryo and hatchling. Poultry Science, 86, 1043–1049.
- Morrison, A.B. & Sarett, H.P. (1958). Studies on zinc deficiency in the chick. Journal of Nutrition, 58, 267–280.
- Morrow, C. (2008). Management as a cause of disease in poultry. In M. Patisson, P.F. McMullin, J.M. Bradbury & D.J. Alexander (Eds.), Poultry Diseases 6th ed. (pp. 536–542). Beijing: Saunders Elsevier.
- Naber, E.C. (1979). The effect of nutrition on the composition of eggs. Poultry Science, 58, 518–528.
- O’Dell, B.L., Newberne, P.M. & Savage, J.E. (1958). Significance of dietary zinc for the growing chicken. Journal of Nutrition, 65, 503.
- Pass, D.A. (1989). The pathology of the avian integument: a review. Avian Pathology, 18, 1–72.
- Powell, S., Bidner, T.D. & Southern, L.L. (2011). Phytase supplementation improved growth performance and bone characteristics in broilers fed varying levels of dietary calcium. Poultry Science, 90, 604–608.
- Richards, M.P. & Weinland, B.T. (1985). Hepatic zinc, copper and iron in the developing turkey embryo and newly hatched poult. Biological Trace Element Research, 7, 269–283.
- Richards, M.P. & Steele, N.C. (1987). Trace element metabolism in the developing avian embryo: a review. Journal of Experimental Zoology, 1, 39–51.
- Richards, M.P. (1989). Influence of egg production on zinc, copper and iron metabolism in the turkey hen (Meleagris gallopavo). Comparative Biochemistry and Physiology, 93, 811–817.
- Richards, M.P. (1991). Distribution and storage of trace minerals in the avian egg. Chapter 25 In B. Momcilovic (Ed.), Trace Elements in Man and Animals (pp. 21–23). Zagreb: IMI Press.
- Richards, M.P. (1997). Trace mineral metabolism in the avian embryo. Poultry Science, 76, 152–164.
- Roberson, R.H. & Schaible, P.J. (1960). The effect of elevated calcium and phosphorus levels on the zinc requirement of the chick. Poultry Science, 39, 837–840.
- Sajewicz-Krukowska, J., Krzysztof, P., Lisowska, A., Pikuła, A., Zenon, M., Króliczewska, B. & Domanska-Blicharz, K. (2016). Astrovirus-induced “white chicks” condition – field observation, virus detection and preliminary characterization. Avian Pathology, 45, 2–12.
- Salim, H.M., Cheorun, J. & Lee, B.D. (2008). Zinc in broiler feeding and nutrition. Avian Biology Research, 1, 5–18.
- Savage, J.E. (1968). Trace minerals and avian reproduction. Federation Proceedings, 27, 927–931.
- Shafety, T.M., McDonald, M.W. & Dingle, J.G. (1991). Effects of dietary calcium and available phosphorus concentration on digesta pH and on the availability of calcium, iron, magnesium and zinc from the intestinal contents of meat chickens. British Poultry Science, 32, 185–194.
- Smyth, V.J., Kaukonen, E., Trudgett, J., Wylie, M., Jewhurst, H., Conway, B., Welsh, M.D. & Todd, D. (2013). Chicken astrovirus detected in hatchability problems associated with “White Chicks”. Veterinary Record, 173, 403–404.
- Smyth, V.J. (2017). A review of the strain diversity and pathogenesis of chicken astrovirus. Viruses, 9, 29–39.
- Solomons, N.W. & Jacob, R.A. (1981). Studies on the bioavailability of zinc in humans: effect of heme and nonheme iron on the absorption of zinc. American Journal of Clinical Nutrition, 34, 475–482.
- Sommer, F. & Cardona, C. (2003). Chicken anemia virus in broilers: dynamics of the infection in two commercial broiler flocks. Avian Diseases, 47, 1466–1473.
- Suzuki, T., Ishihara, K., Migaki, H., Matsuura, W., Kohda, A., Okumura, K., Nagao, M., Yamaguchi-Iwai, Y. & Kambe, T. (2004). Zinc transporters, ZnT5 and ZnT7, are required for the activation of alkaline phosphatases, zinc-requiring enzymes that are glycosylphosphatidylinositol-anchored to the cytoplasmic membrane. The Journal of Biological Chemistry, 280, 637–643.
- Tako, E., Ferket, P.R. & Uni, Z. (2005). Changes in chicken intestinal zinc exporter mRNA expression and small intestinal functionality following intra-amniotic zinc-methionine administration. Journal of Nutritional Biochemistry, 16, 339–346.
- Terrin, G., Canini, R.B., Di Chiara, M., Pietravalle, A., Aleandri, V., Conte, F. & De Curtis, M. (2015). Zinc in early life: a key element in the fetus and preterm neonate. Nutrients, 7, 10427–10446.
- Todd, D., Wilkinson, D.S., Jewhurst, H.L., Wylie, M., Gordon, A.W. & Adair, B.M. (2009). A seroprevalence investigation of chicken astrovirus infections. Avian Pathology, 38, 301–309.
- Tupper, R., Watts, R.W.E. & Wormall, A. (1954). The incorporation of 65Zn into avian eggs. Biochemistry, 57, 245–255.
- Vallee, B.L. & Auld, D.S. (1990). Zinc coordination, function, and structure of zinc enzymes and other proteins. Biochemistry, 29, 5647–5659.
- Vallee, B.L. & Falchuk, K.H. (1993). The biochemical basis of zinc physiology. Physiological Reviews, 73, 79–118.
- van Ravenswaay, R. O. (1986). Effect of dietary iron on tissue mineral composition as a measure of bioavailability of different iron sources. M.S. thesis, University of Florida, Gainesville, FL.
- Vieira, S.L. (2007). Chicken embryo utilization of egg micronutrients. Brazilian Journal of Poultry Science, 9, 1–8.
- Walk, C.L., Bedford, M.R. & McElroy, A.P. (2012). Influences of limestone and phytase on broiler performance, gastrointestinal pH, and apparent ileal nutrient digestibility. Poultry Science, 91, 1371–1378.
- Wilson, J.X., Lui, E.M.K. & Del Maestro, R.F. (1992). Developmental profiles of antioxidant enzymes and trace metals in chick embryo. Mechanisms of Ageing and Development, 65, 51–64.
- Whitehead, C.C., Rennie, J.S., McCormack, H.A. & Hocking, P.M. (1993). Defective down syndrome in chicks is not caused by riboflavin deficiency in breeders. British Poultry Science, 34, 619–623.
- Yair, R. & Uni, Z. (2011). Content and uptake of minerals in the yolk of broiler embryos during incubation and effect of nutrient enrichment. Poultry Science, 90, 1523–1531.
- Young, R.J., Edwards, H.M. & Gillis, M.B. (1958). Studies on zinc in poultry nutrition. II. zinc requirement and deficiency symptoms of chicks. Poultry Science, 37, 1100–1107.