ABSTRACT
Parrot bornavirus (PaBV) is a pathogen often found in psittacine populations. Infected, clinically healthy carrier birds are of major importance for epidemiology, but the underlying pathomechanism of this carrier status is poorly understood. The age, implying the maturation status of the immune system, at the time of infection might be significant for the clinical outcome. Therefore, two groups of 11 cockatiels of different ages (adult and newly hatched) were inoculated with a PaBV-4 isolate intravenously. The trial lasted for 233 days and all birds were observed for clinical signs, PaBV-RNA shedding and anti-PaBV antibody production. At the end of the trial, histopathology, immunohistochemistry, PCR and virus re-isolation were performed. All 22 birds seroconverted and shed PaBV-RNA during the investigation period; the juvenile group earlier and more homogeneously. Nine of 11 birds of the adult group developed clinical signs; five birds died or had to be euthanized before the end of the study. In the juvenile group none of the birds developed clinical signs and only one bird died due to bacterial septicaemia. Eight birds of the adult group, but none of the juvenile group, showed a dilatation of the proventriculus. PaBV-RNA detection and virus re-isolation were successful in all birds. Immunohistochemically, PaBV antigen was found in all birds. Histopathology revealed mononuclear infiltrations in organs in birds of both groups, but the juveniles were less severely affected in the brain.
Thus, PaBV infection at an age with a more naïve immune system makes the production of carrier birds more likely.
RESEARCH HIGHLIGHTS
PaBV infection at a young age might favour the development of carrier birds.
Cockatiels infected at a very young age showed inflammation but no clinical signs.
The juvenile group started seroconversion and PaBV-RNA shedding earlier.
Seroconversion and PaBV-RNA shedding occurred more homogeneously in the juveniles.
Abbreviations | ||
ABV | = | avian bornavirus (old taxonomy) |
BoDV | = | Borna disease virus (new taxonomy) |
cDNA | = | complementary deoxyribonucleic acid |
CEC-32 | = | a quail cell line |
CNS | = | central nervous system |
Ct | = | cycle threshold |
DNA | = | deoxyribonucleic acid |
dpi | = | lat.: dies post infectionem (= days post infection) |
g | = | 1. (phys.) gravitational force (here in centrifugation) |
= | 2. (phys.) gram (weight) | |
HE | = | haematoxylin & eosin (staining) |
hr | = | hour |
IIFA | = | indirect immunofluorescence assay |
MDCK | = | Madin-Darby canine kidney (cells) |
n | = | number of birds |
PaBV | = | parrot bornavirus (new taxonomy) |
PCR | = | polymerase chain reaction |
PDD | = | proventricular dilatation disease |
pi | = | lat.: post infectionem (= post infection) |
RNA | = | ribonucleic acid |
RT-PCR | = | reverse transcription polymerase chain reaction |
SPF | = | specific pathogen free |
TCID50 | = | 50% tissue culture infective dose |
Introduction
The genus Orthobornavirus includes eight virus species of which two have zoonotic potential (Mammalian 1 orthobornavirus and Mammalian 2 orthobornavirus) (Hoffmann et al., Citation2015; Schlottau et al., Citation2018). For psittacines, two species are of major importance in captive and wildlife populations (Psittaciform 1 orthobornavirus and Psittaciform 2 orthobornavirus). Studies demonstrate that up to 22.8% of the parrots, in various collections in captivity, are infected (Heffels-Redmann et al., Citation2011). Clinical signs of parrot bornavirus (PaBV) infection include central nervous system (CNS) signs like seizures, opisthotonus, ataxia, tremors or central blindness, and gastrointestinal signs like diarrhoea with undigested seeds in the faeces, dilated proventriculi and intermittent regurgitation and vomiting, polydipsia and polyuria as well as unspecific signs like general depression and weight loss leading to death (Mannl et al., Citation1987; Berhane et al., Citation2001; Hoppes et al., Citation2010; Dahlhausen & Orosz, Citation2018). Under field conditions, infection does not seem to occur frequently. In one study 32.2% of the birds in one infected population were tested positive (Lierz et al., Citation2009); in another study 26% of exposed birds were found to be positive despite close contact of the birds in the flock (Kistler et al., Citation2010). Furthermore, there are frequent cases of single pairs of parrots where only one of the partners is PaBV-positive. This field observation has already been verified through multiple studies where uninfected sentinel birds, that were kept in close contact with experimentally infected birds, did not get infected (Gancz et al., Citation2009; Gray et al., Citation2010; Hoppes et al., Citation2010; Rubbenstroth et al., Citation2014). Since the discovery of avian bornaviruses there has been an assumption of a faecal-oral transmission (Rinder et al., Citation2009; Kistler et al., Citation2010; Lierz et al., Citation2010; Raghav et al., Citation2010), but observations in the field raise doubts about that possibility. Experimentally, infection was possible through intramuscular, subcutaneous, intravenous or intracerebral injections or a combination of these routes (Gancz, Kistler, Clubb, et al., Citation2009; Gray et al., Citation2010; Hoppes et al., Citation2010; Mirhosseini et al., Citation2011; Piepenbring et al., Citation2012; Högemann et al., Citation2017). Heckmann et al. (Citation2017) were able to demonstrate for the first time that oral or nasal inoculations do not result in valid infections (Heckmann et al., Citation2017). The main natural route of transmission, therefore, remains unclear to this date.
Lierz et al. (Citation2011) investigated the possibility of vertical transmission by testing embryos that died before hatching from a PaBV-positive flock. It was possible to detect PaBV-RNA in two of 30 embryos (6.67%), but no histopathologic lesions were found (Lierz et al., Citation2011). This gives first hints of possible vertical transmission, but no virus could be isolated. Furthermore, it seems that infected embryos do not necessarily show histopathological alterations, in contrast to infected and diseased adults. In another study, PaBV-RNA was detected in embryos and hatchlings of PaBV-infected sun conures (Aratinga solstitialis), but the chicks were raised by their own parents, so a horizontal infection, at least in the hatchlings, could also be possible. Virus re-isolation was not performed in that study (Kerski et al., Citation2012).
Interestingly, there are cases where several birds are positive in one flock but only a few develop clinical signs (Heffels-Redmann et al., Citation2011), so infected clinically healthy individuals have been repeatedly reported (De Kloet & Dorrestein, Citation2009; Lierz et al., Citation2009; Encinas-Nagel et al., Citation2014). To date, factors that affect the outcome of the disease and the development of clinical signs still remain unclear.
For Borna disease virus (BoDV-1), the pathogen that causes Borna disease (BD) in mammals, it was shown that after experimental infection only immunocompetent adult rats develop clinical signs, whereas immunocompromised or neonatally infected rats remain healthy. Therefore, an immune-mediated pathogenesis was assumed and proven (Hirano et al., Citation1983; Narayan et al., Citation1983b; Rott et al., Citation1988). Virus replication in adult immunocompetent rats was restricted to the cells of the CNS and could be detected constantly over the investigation period (Narayan et al., Citation1983b; Herzog et al., Citation1984). By transfer of T-lymphocytes from rats with BD to immunocompromised birds, the disease was initiated in the latter (Rott et al., Citation1988) when given after the infection. This study supports the theory that clinically apparent BD is initiated through immunopathogenesis, which was later characterized as a T-cell-mediated delayed-type hypersensitivity (Stitz et al., Citation2002). After experimental immunosuppression with cyclosporin A before BoDV-1 infection, clinical signs, as well as histological alterations, were prevented. A challenge infection after completion of the treatment with cyclosporin A did not result in clinical signs either, suggesting that immunosuppression during infection causes immunotolerance in the infected birds (Stitz et al., Citation1989). Hameed et al. demonstrated that treatment with cyclosporin A also prevents clinical signs and dilated proventriculi in adult PaBV-infected cockatiels (Hameed et al., Citation2018). In contrast to the results of studies with BoDV-1 infected rats, newborn mice develop severe clinical signs after infection with BoDV-1 (Hallensleben et al., Citation1998), while adult mice seem to be resistant to the onset of clinical signs. After multiple passages in mouse brain tissue, an adaption to mice was accomplished, which resulted in the adult mice developing clinical signs of BD (Rubin et al., Citation1993). Gerbils (Meriones unguiculatus), on the other hand, develop clinical signs regardless of their age during infection and even after immunosuppression with cyclosporin A (Watanabe et al., Citation2001, Citation2003). These studies demonstrate that even closely related rodent species show very different reactions to BoDV-1 infection. For avian bornaviruses, no studies regarding age-dependent courses of infection have been carried out so far.
The hypothesis of this study was that immunoincompetent cockatiel chicks develop an immunotolerance for PaBV after infection in the first days of life and become virus carriers without showing clinical signs of proventricular dilatation disease (PDD) and potentially do not even show histopathological alterations. On the other hand, cockatiels infected as adults should develop signs and histopathological alterations. Therefore, the aim of this study was to evaluate age-dependent differences in the development and severity of clinical signs, as well as the shedding of virus and seroconversion after the infection of cockatiels of different ages.
Materials and methods
Experimental birds
Eleven adult cockatiels (Nymphicus hollandicus) (five breeding pairs and one additional female) from a specific pathogen-free breeding flock, that is regularly tested negative for paramyxovirus-1, psittacine herpesvirus, Chlamydia psittaci and PaBV, and 11 newly hatched cockatiel chicks were used. The birds of the juvenile group were termed “J1” to “J11”; the adults were ascribed a letter for each pair (“A” to “E” plus the additional female with the letter “F”) and a number for the sex (“1,0” for the male, “0,1” for the female of each pair). The adult birds were aged between 1 and 5 years, were in good general condition and showed no alterations in the radiographic examination. Prior to the start of this study the PaBV-free status of every adult bird was again confirmed by real-time reverse transcription PCR (RT–PCR) (Honkavuori et al., Citation2008) of crop and cloacal swabs as well as by serology (indirect immunofluorescence assay [IIFA]) (Herzog et al., Citation2010) twice with a 2-week interval. The 11 cockatiel chicks (aged 1–6 days at infection) were raised by the breeding pairs of the adult group, who acted as foster parents, so hand-raising would not influence the study. To ensure all 11 chicks were accepted and infection could occur at the same time, the breeding birds needed to be in the same breeding status. Therefore, they were synchronized by separation of the partners, that were kept solitarily for 2 weeks, and simultaneous reunion of all five pairs as well as optimal breeding conditions to stimulate the birds to breed. When three females started laying eggs, additional eggs were collected from the breeding flock and incubated at the same time in an incubator. Within one week, 11 chicks were distributed immediately after hatching to the three breeding pairs in the trial, which accepted them as their own (pair A: J1, J2, J3; pair B: J3, J4, J5, J7; pair C: J8, J9, J10, J11).
The birds were kept in breeding boxes (JoKo Systemtechnik, Maxhütte-Haidhof, Germany) that were customized for our purpose. The boxes were made from plastic, with three closed walls and one metal grid in the front, and were furnished with sterilized hazelnut branches. They measured 162.5 × 50 × 50 cm with the possibility to separate them into two compartments (81.25 × 50 × 50 cm) to catch each bird individually. This plastic separation wall was also used for the synchronization process. Food and water could be changed from the outside without reaching into the boxes and special UV-light bulbs in front of the cages ensured a natural light spectrum and calcium metabolism. Each box had two nesting boxes attached on the sides with a closable entrance. This way, breeding pairs could reproduce without disturbance, encouraging natural behaviour. Additionally, the nesting boxes were easily accessible and could be changed for cleaning without handling the adult birds. In the beginning, each box housed one pair of cockatiels (in one box due to the uneven number three cockatiels) plus their chicks. After weaning, the juveniles were separated from the parents and henceforth kept in pairs or groups of maximum four birds in identical conditions to the adult group. The birds were fed ad libitum with the standard seed-mix “Prestige Big Parakeets” (Versele Laga®, Belgium, 9800 Deinze), egg-food (CéDé®, Belgium, 9040 Evergem) and mineral grit (Futtermittel Jehl, Germany, 93142 Maxhütte-Haidhof).
This work was accomplished with governmental permission (file reference: GI 18/9-NR.36/2015).
Virus isolate
The PaBV-4 isolate (Ps 34) used for inoculation originated from brain material of a scarlet macaw (Ara macao) that died from PDD. As the same isolate was used in previous trials with the same route of infection (Piepenbring et al., Citation2012, Citation2016), comparison between these trials was possible. The isolate was passaged six times in a quail cell line (CEC-32). The PaBV-infected CEC-32 cells were sonicated in medium with 2% foetal bovine serum (FBS) and centrifuged for 10 min at 800 × g. The infectivity of the supernatant was verified in CEC-32 cells.
Experimental design
All 22 birds were inoculated in the Vena jugularis with the PaBV-4 isolate in a dose of 103 TCID50 one after the other within 2 h. At this time, the chicks were aged between 1 and 6 days.
Over a period of 233 days, the birds of both groups were examined twice a day for any clinical signs. Three times a week crop and cloacal swabs were taken to detect PaBV RNA using real-time RT-PCR (Honkavuori et al., Citation2008) and the body weight was measured. After all birds were continuously positive in the RT–PCR, the sampling was reduced to once per week. Once per week, blood samples were taken for detection of antibodies against PaBV by IIFA (Herzog et al., Citation2010). When all birds had seroconverted, blood sampling was reduced to once every 4 weeks. Only one juvenile bird (J8) was tested weekly until 183 days post-infection (dpi), because it seroconverted much later than the other birds. At the end of the trial all birds were euthanized by blood withdrawal in deep isoflurane anaesthesia. Birds that demonstrated severe signs were euthanized beforehand for humane reasons.
Necropsy
In all 22 birds, gross pathology was performed and alterations were recorded. Samples were taken from the cerebrum, cerebellum, spinal cord, eye, sciatic nerve, heart, liver, kidney, adrenal gland, spleen, crop, proventriculus, gizzard, small and large intestine, pancreas, pectoral muscle, gonads and skin with feathers (two regions) and stored at −80°C until real-time RT–PCR testing. The same sample selection was fixed in 4% buffered formalin for histopathologic and immunohistochemical examination. Fresh tissue from the brain, retina, liver, kidney and sciatic nerve of each bird was used for virus re-isolation. Additional reserve samples from all organs were also stored at –80°C.
For bacteriological examination, samples of blood from the heart, the liver and lung were taken, streaked on sheep blood agar plates and Gassner Lactose Agar (Water-Blue Metachrome Yellow Lactose Agar, Oxoid Deutschland GmbH, Wesel, Germany) and incubated for 48 h at 37°C. For mycological culturing, samples from crop and proventriculus were cultured on Kimmig Fungal Agar (Oxoid Deutschland GmbH) for 5 days at 28°C. Furthermore, smears of crop and proventriculus material were Giemsa stained and microscopically scanned for yeasts. Additionally, samples from the intestinal contents were examined microscopically for parasites.
RT–PCR
RNA extraction was performed using the RNeasy Mini Kit (Qiagen, Hilden, Germany) according to manufacturer’s instructions. A volume of 600 µl of isolated total RNA from the pooled crop and cloacal swabs or organ samples, respectively, of each bird and timepoint, were then transcribed by random hexamer primers (Qiagen) according to the manufacturer’s instructions. The real-time RT–PCR was done with a Rotor-Gene Q cycler (Qiagen) using the primer pair “1034-1322” (Honkavuori et al., Citation2008) for detecting the amino-terminal region of the phosphoprotein (P) gene of PaBV-4. The RT–PCR was considered positive when the course of the graphs was exponential and the Ct-value (cycle threshold value) was below 42.
Indirect immunofluorescence assay
IIFA for the investigation of specific antibodies against PaBV was performed with persistently PaBV-infected CEC-32 cells (homologous system) (Herzog et al., Citation2010).
Virus isolation
The re-isolation of virus was performed by infectivity assay (Narayan et al., Citation1983b) with polyclonal serum from experimentally BoDV-infected rats, (Herzog et al., Citation2010). Therefore, samples from the brain, retina, liver, kidney and sciatic nerve of each bird were sonicated and a 10% dilution in GibcoTM Glasgow Minimum Essential Medium (Thermo Fisher Scientific, Waltham, MA) with 2% foetal bovine serum was made. CEC-32 cells were incubated with the samples for 5 days at 37°C. Virus replication was examined by using indirect immunofluorescence.
Histopathology and immunohistochemistry
The tissue samples were stained with haematoxylin and eosin (H&E) for the identification of histological alterations. The avidin–biotin complex method, using the polyclonal rabbit anti-BoDV-P antibody directed against the bornaviral phosphoprotein p24, was used for immunohistochemistry (Herden et al., Citation1999; Herzog et al., Citation2010; Piepenbring et al., Citation2012).
Statistics
The t-test to compare first virus shedding and seroconversion of both groups, the Wilcoxon–Mann–Whitney test to compare the histological and immunohistochemical results, the Grubbs’ test for the calculation of outliers, and the Chi-squared test, as well as the Fisher’s test to compare clinical data were used. P-values < 0.05 were considered as statistically significant.
Results
Clinical observation
During the time of observation, nine of 11 adult birds developed typical clinical signs of a PaBV infection at different timepoints during infection (). The signs ranged from apathy (n = 6), gastrointestinal signs (vomiting n = 7, diarrhoea n = 1, undigested seeds in the faeces n = 3), neurological signs (ataxia n = 2, tremor n = 1), weight loss (n = 9), polyuria/polydipsia (n = 1) to death (n = 2). Three birds had to be euthanized due to the severity of the signs. One juvenile (J9) showed rapid weight loss from day 56 onwards and died shortly after (66 dpi) without other signs. The other 10 juvenile birds showed no abnormalities or clinical signs (). The differences between the two groups were highly significant (Fisher’s test, P = 0.001905). The weight curve of the adult birds showed more variation, especially in the laying females (B0,1, C0,1, E0,1 and F0,1) (), the weight of the juveniles was more stable during the trial (). The birds J3, J4, J7 and J11 started laying eggs after becoming sexually mature, which can also be seen as slight peaks in the weight curve. The peaks of the weight curves match the clutch of eggs of the laying females – the weight always increases before the first egg of a new clutch is laid.
Figure 1. Weight (g) of the adult group of cockatiels after infection with PaBV-4 at different ages. Laying females (A0,1, B0,1, C0,1, E0,1 and F0,1) in colour. Arrows mark the timepoint of the first egg of one clutch. Colour online.
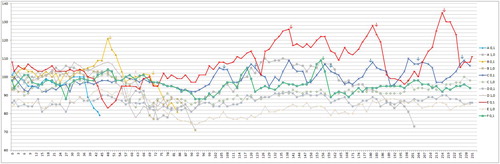
Figure 2. Weight (g) of the juvenile group of cockatiels after infection with PaBV-4 at different ages. Laying females (J3, J4, J7 and J11) as well as the outlier J9 in colour. Arrows mark the timepoint of the first egg of each clutch. Females J3, J4 and J7 were using the same nest, so it was not always possible to know which egg was from which female. The eggs where no assignment to one female was possible are not shown here. Colour online.
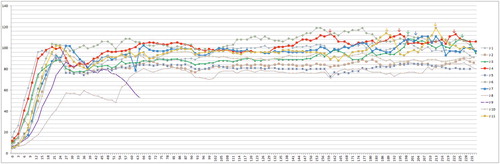
Table 1. Clinical findings of cockatiels after infection with PaBV-4 for the different age groups (values are dpi).
Indirect immunofluorescence assay
Anti-PaBV antibodies were detected in all birds. The first detection was possible in six birds (three juvenile, three adult birds) at 36 dpi, the latest seroconversion was shown by the bird J8 at 99 dpi, which could be calculated as outlier statistically via Grubbs’ test. The juvenile birds showed on average an earlier seroconversion than the adult birds, but with a low significance (P-value: 0.0502) (). The titre increased in all birds very fast, with highest values of 1:81,290, 1:163,840 or 1:327,680, respectively. There was no difference in the titre levels between the groups ( and ).
Figure 3. Box plot diagram showing the first detection of anti-PaBV antibodies in cockatiels after infection with PaBV-4 4 in different age groups (juvenile average: 44 dpi, min: 36 dpi, max: 57 dpi; adult average: 54 dpi, min: 36 dpi, max: 71 dpi). The juvenile group seroconverted earlier and more homogenously (P = 0.0502). One outlier in the juvenile group (seroconversion at 99 dpi) was excluded from the diagram.
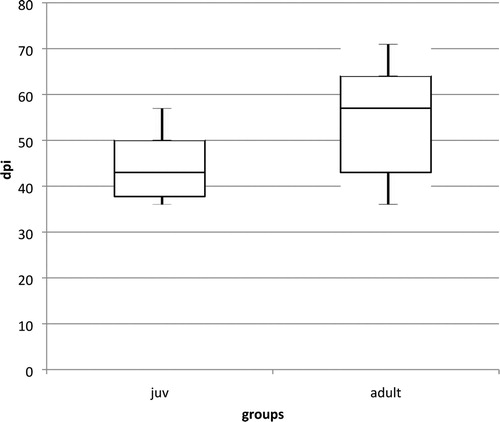
Necropsy
Eight of 11 adult birds showed alterations in gross pathology: dilated proventriculi (n = 4 severe, n = 2 moderate and n = 2 mild dilatation). Three adults showed no alterations. None of the juvenile birds revealed any macroscopic abnormalities (). The differences between the two groups were highly significant (P = 0.001032). The parasitological and bacteriological examinations of all but one bird showed no abnormal findings. Bacillus cereus and Escherichia coli were isolated from samples from the heart, liver and kidney of the juvenile that died (J9). Yeasts were detected microscopically in the microscopic investigation of smears from crop and proventriculus in two of 11 adult and six of 11 juvenile birds. The yeast could not be cultivated in any of these cases.
Table 2. Post mortem findings of cockatiels after infection with PaBV-4 in different age groups.
RT–PCR
In all 22 birds PaBV-RNA was detected in swabs during the observation period. The first detection was successful at 27 dpi in three birds of the juvenile group. The birds of the juvenile group started shedding on average earlier than the adult group (average 32.73 dpi versus 44.9 dpi), which was highly significant (P = 0.0011) (). In the juvenile group, one bird (J8) showed a much later PaBV-RNA shedding than the rest of the group (first detection on 52 dpi, intermittent shedding until 83 dpi) and was identified statistically as an outlier using Grubbs’ test. The rest of the juvenile group showed a very homogenous start of PaBV-RNA-shedding (27–36 dpi). In the adult group the values were more heterogenous (31–63 dpi). From 90 dpi onwards, all birds were constantly positive in the RT–PCR assay and the investigation of swabs was reduced to once per week ().
Figure 6. Box plot diagram showing the first detection of PaBV-RNA in combined crop and cloacal swabs of cockatiels after infection with PaBV-4 in different age groups (juvenile average: 31 dpi, min: 27 dpi, max: 36 dpi; adult average: 45 dpi, min: 31 dpi, max: 66 dpi). The juvenile group started shedding earlier and more homogenously, which was highly significant (P = 0.0011). One outlier in the juvenile group (first detection at 52 dpi) was excluded from the diagram.
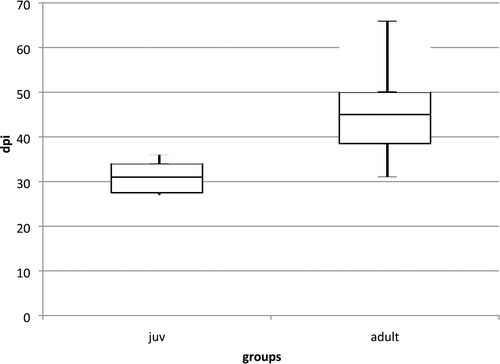
In all birds PaBV-RNA was found in the organ samples. Only a few samples from the pectoral muscle (birds C1,0, D1,0, J1, J2, J4, J7), two samples of the liver (C1,0, D1,0) and one sample of the crop (C 1,0) were negative. The Ct-values of positive samples ranged from 9.88 (cerebrum of J6) to 41.06 (pectoral muscle of C0,1). The lowest average Ct-values (meaning the highest viral load) were found in the cerebrum (14.44), cerebellum (14.05), sciatic nerve (14.03) and adrenal glands (14.23) ().
Table 3. Viral RNA content in the organ samples of cockatiels after infection with PaBV-4 at different ages (Ct-values).
Histopathology and immunohistochemistry
Histopathologic examination revealed mononuclear infiltrates characteristic for PDD in the brain of all 11 birds of the adult group and in five of 11 of the juvenile group, that was also less severe in the juveniles, as well as in the spinal cord and ganglia of all 11 adults and 10 of 11 juveniles. In the crop of nine of 11 adult and eight of 11 juveniles, as well as in the proventriculus of five of 11 adults and eight of 11 juveniles, there were signs of inflammation also characteristic for PDD. There was a difference in the distribution pattern of the samples from the brain (more severe inflammation in the adults) and potentially in the samples from the proventriculus (more severe in the juveniles). The differences between the adults and the juveniles in the brain were significant (P = 0.0051), in contrast to the differences in the proventriculus (P = 0.0994). In the immunohistochemical examination, bornavirus antigen was detected in all 22 birds. All 22 birds were positive in the brain and spinal cord, 21 of 22 in the intestine, eight of 10 in the sciatic nerve, 14 of 21 in the eye, 15 of 17 in the adrenal gland, 20 of 22 in the crop, and 18 of 20 in the proventriculus. There was no detectable difference in the distribution pattern between the groups.
Virus isolation
Viability of the inoculated virus suspension used for infection was confirmed in CEC-32 cells. In all birds, virus was isolated from the liver, brain, retina, kidney and sciatic nerve. The samples from the liver and kidney of J2 were contaminated and therefore not evaluable, but the other organs were positive in this bird.
Discussion
The aim of the study was to investigate the age-dependency of the clinical outcome of infection with PaBV-4 in cockatiels which were either infected as adults or as chicks. It was shown that an early infection in the first days of life did not lead to PaBV-associated clinical signs during 233 days in the infected chicks, while adult cockatiels developed the typical severe clinical course of PaBV infections.
However, the course of infection was almost identical in both groups (virus shedding, seroconversion, presence of infectious virus). There was a tendency in the juvenile group for a slightly earlier and more homogenous presence of the aforementioned virus parameters. A possible explanation for this is that the dose of infection was the same in both groups, regardless of the different bodyweight, so that this might have influenced the incubation period. Moreover, the juvenile group could be per se much more homogenous itself regarding age and immune status compared to adult breeding pairs of very different ages and immunological history. Besides the similar course of infection, the clinical outcome was very different between the groups.
In the gross pathology, most of the adult birds showed dilatated proventriculi, which was not present in any of the juvenile birds. Except for the bird A0,1 that died with unspecific signs such as weight loss and apathy, but still showed a slightly dilatated proventriculus, all the other birds with dilatated proventriculi showed gastrointestinal signs (undigested seeds in the faeces, or regurgitation). For comparison, the juvenile group showed no macroscopic alterations, which matches with the clinical observations of no gastrointestinal signs in any of the juvenile birds. The difference between the two groups was highly significant and shows that the juveniles infected as chicks did not develop clinical PDD.
The Ct-values of the PaBV-RNA detection in the organ samples in the juvenile group were slightly lower on average than those of the adult group. For the interpretation of this difference between the groups it is important to notice that the highest Ct-values were seen in birds that died early (A0,1, B0,1, B1,0, D1,0), with the exception of C 1,0, which had relatively high Ct-values despite surviving until the end of the trial (). Five of 11 adult birds died or were euthanized before the end of the trial. These findings indicate an increase of viral load during infection (and therefore Ct-values decrease) as already seen in a similar study with subadult cockatiels (Piepenbring et al., Citation2016) and in BoDV-1 infections in rats (Hirano et al., Citation1983; Narayan et al., Citation1983a). The difference in the Ct-values between the groups can thus be caused by the different survival time after infection, rather than by a different course of infection between the juvenile and the adult group. The size of the organ samples was not standardized, therefore statistical calculations were not practical. Nevertheless, these data showed that very low Ct-values in the organ samples were present in birds of both groups without obvious differences in the distribution pattern of viral RNA in the organs between the groups.
Virus was re-isolated from all birds in most of the tested organs. Therefore, a valid infection in all birds was proven, again interestingly without differences between the groups. In previous studies using subadult cockatiels, virus re-isolation was also possible (Piepenbring et al., Citation2012, Citation2016), but in experimentally-infected embryos this was not possible (Wüst, Citation2017). This could point to the fact that an even earlier infection (before hatching) might lead to a different outcome than an early infection in already hatched chicks.
In the immunohistological examination, PaBV-antigen was found in multiple organs in all birds of both groups without any significant difference between the two cohorts regarding the virus distribution in the organs. The findings are in accordance with previous studies in subadult cockatiels, where multiple organs regularly harboured virus antigen (Piepenbring et al., Citation2012, Citation2016) but not with age-dependent BoDV-1 infection in rats. In these studies, BoDV-1 shows a strict neurotropism in adult rats in contrast to neonatally-infected rats where all organs were virus-positive (Herzog et al., Citation1984). In PaBV-infected cockatiel embryos in ovo, virus antigen was also only found in the brain, especially in the cerebellum (Wüst, Citation2017). This again indicates that the time of infection plays a very important role in the pathogenesis of PaBV infections.
In this study, it could be proven that the immune system of the infected chicks already reacts to the infection (antibody production, inflammation) indicating presence of immunocompetence. However, in comparison to adult birds, juveniles do not have a fully developed competent immune system (Seto, Citation1981). A complete immunotolerance did not seem to occur in the juvenile group, but a modulated immune reaction seems likely. Mostly responsible for the immunopathogenesis (Planz & Bilzer, Citation1995; Stitz et al., Citation1998; Furrer et al., Citation2001; Baur et al., Citation2008), is a delayed-type hypersensitivity reaction with CD4+ and CD8+ T-cells as effector cells (Nöske et al., Citation1998). This could also explain why the immunopathogenesis does not necessarily correlate with the production of serum antibodies. In this study, antibody titre increased in all birds and reached high titres (up to 1:163,840) in both groups.
In contrast to the juvenile infected cockatiels in this study, in ovo-infected embryos did not show inflammatory lesions in any organ (Wüst, Citation2017). This might be due to a more immature immune system in the embryos so that a vertical infection in ovo results in less alterations maybe even in total immune tolerance. Age-dependent differences are already well known for BoDV-1 infections; adult rats became sick and died, while neonatal rats did not develop severe neurological signs (Hirano et al., Citation1983; Herzog et al., Citation1984; Briese et al., Citation1999). Nevertheless, juvenile rats in some studies showed behavioural abnormalities such as hyperactivity, inhibition of open-field exploration and stereotypic behaviours (Hornig et al., Citation1999; Pletnikov et al., Citation2002, Citation1999), dwarfism and anatomical differences in the brain structure (Narayan et al., Citation1983a; Carbone et al., Citation1991; Bautista et al., Citation1995; Eisenman et al., Citation1999; de la Torre, Citation2002; Pletnikov et al., Citation2002). Behavioural abnormalities (despite slight neurological signs like tremor and ataxia in the birds A1,0, C1,0 and D1,0) were not seen in any of the cockatiels in this study, especially not in the juvenile group. In none of these studies did the neonatal infected rats show signs of inflammation despite degenerative potentially developmental lesions in the brain (Hirano et al., Citation1983; Herzog et al., Citation1984; Carbone et al., Citation1991; Bautista et al., Citation1995; Eisenman et al., Citation1999; Hornig et al., Citation1999). The present study also showed no signs of neuroanatomical alterations in the juvenile group, but, in contrast to the rat studies, signs of inflammation occurred in the clinically healthy juvenile cockatiels. Histologically, both groups showed a non-purulent infiltration in many organs, but the inflammation was less severe or absent in the brain of the juveniles. A possible explanation for the presence of inflammation without the adequate clinical signs is that the immune cell activity is not fully developed or might rather still be based on the innate immune system than on a cellular one. A higher ability of regeneration in the juvenile neuronal tissue could also be the reason why the juveniles did not develop clinical disease in the period studied after infection. Additionally, the loss of function of neuronal cells might be better compensated in the juveniles than in the adult group due to more flexibility in the juvenile brain. The fact that many persistently-infected neonatal rats did not show neurological signs, regardless of severe morphological alterations, indicates that the juvenile brain is able to compensate these lesions at least functionally (Bautista et al., Citation1994; Citation1995; Eisenman et al., Citation1999). It is also possible, that the immune response was slightly different in the juveniles, leading to an elimination of the infection, as it has been shown for BoDV under special circumstances in rats. It was shown that passive immunization with CD4+ cells led to transient inflammation and elimination of the virus (Richt et al., Citation1994; Nöske et al., Citation1998).
In the juvenile group there was one outlier (J8) which seroconverted much later than the rest of the group and showed a delayed shedding of PaBV-RNA. One possible explanation could be that the infection route in this bird was accidentally subcutaneous rather than intravenous. In grey parrots a subcutaneous infection with PaBV resulted in a PaBV-RNA detection in swabs starting in weeks 12 and 20 in two birds (Högemann et al., Citation2017). Even if grey parrots and cockatiels cannot be compared directly, the route of infection seems to play a major role regarding the incubation period. The juvenile J9 died after a sudden weight loss during the time of weaning. In this bird the bacteriological examinations detected a Bacillus cereus isolate in all investigated organs, therefore a bacterial septicaemia is the presumed cause of death. During the time of weight loss, the father of this juvenile (C1,0) also showed apathy, and therefore suddenly stopped feeding the juveniles, which could also have played a role in the disease of J9. The other juveniles of this clutch were already feeding on their own; J9 was the last one that was sometimes seen begging for food. After the weight loss was noticed the bird was fed additionally with handfeeding formula, but it nevertheless died a few days afterwards.
In the parasitological and bacteriological examinations, as well as in the mycological cultivation, all birds except J9 were negative, therefore there were no signs of secondary infections except for that one bird.
In summary, this study provides the first evidence that the course of PaBV infections is dependent on the age at time of infection. The exact pathomechanism and different outcomes of PaBV infection are still unknown, but this study has clearly shown that PaBV infection of chicks leads to the clinically inapparent course of the infection with virus shedding typically found in carrier birds. Also, juvenile infected cockatiels started shedding of PaBV-RNA earlier than birds infected as adults and developed seroconversion with high virus-specific antibody titres, despite not showing any clinical signs. However, it should be emphasized that the course of disease seems to be strongly age-dependent, starting already in the short timeframe of vertical infection as embryo or infection of chicks, in contrast to the infection of adults. Thus, vertically or very early infected parrots could become clinically healthy carrier birds and play an important role in the epidemiology of PaBV infections. This knowledge is especially important for the interpretation of the testing results of juvenile birds. Based on this study in cockatiels, testing using the PCR in juveniles before the age of 7 weeks or using serology before 14 weeks may lead to false assumptions of virus-free birds. In other species those data may differ. More research is necessary to understand the underlying pathomechanism leading to different courses of the disease and the occurrence and chronological process of inflammation in juvenile infected psittacines.
Disclosure statement
No potential conflict of interest was reported by the authors.
Additional information
Funding
References
- Baur, K., Rauer, M., Richter, K., Pagenstecher, A., Götz, J., Hausmann, J. & Staeheli, P. (2008). Antiviral CD8 T cells recognize Borna disease virus antigen transgenically expressed in either neurons or astrocytes. Journal of Virology, 82, 3099–3108.
- Bautista, J.R., Rubin, S.A., Moran, T.H., Schwartz, G.J. & Carbone, K.M. (1995). Developmental injury to the cerebellum following perinatal Borna disease virus infection. Developmental Brain Research, 90, 45–53.
- Bautista, J.R., Schwartz, G.J., de la Torre, J.C., Moran, T.H. & Carbone, K.M. (1994). Early and persistent abnormalities in rats with neonatally acquired Borna disease virus infection. Brain Research Bulletin, 34, 31–40.
- Berhane, Y., Smith, D.A., Newman, S., Taylor, M., Nagy, E., Binnington, B. & Hunter, B. (2001). Peripheral neuritis in psittacine birds with proventricular dilatation disease. Avian Pathology, 30, 563–570.
- Briese, T., Hornig, M. & Lipkin, W.I. (1999). Bornavirus immunopathogenesis in rodents: models for human neurological diseases. Journal of Neurovirology, 5, 604–612.
- Carbone, K.M., Park, S.W., Rubin, S.A., Waltrip II, R.W. & Vogelsang, G.B. (1991). Borna disease: association with a maturation defect in the cellular immune response. Journal of Virology, 65, 6154–6164.
- Dahlhausen, R.D. & Orosz, S.E. (2018, September). Avian bornaviral ganglioneuritis in clinical practice. In Proceedings of the annual association of avian veterinarians, exotics conference. e veterinary clinics of North America (Vol. 21, pp. 33–67). Exotic Animal Practice.
- De Kloet, S.R. & Dorrestein, G.M. (2009). Presence of avian bornavirus RNA and anti-avian bornavirus antibodies in apparently healthy macaws. Avian Diseases, 53, 568–573.
- de la Torre, J.C. (2002). Bornavirus and the brain. Journal of Infectious Diseases, 186, 241–247.
- Eisenman, L.M., Brothers, R., Tran, M.H., Kean, R.B., Dickson, G.M., Dietzschold, B. & Hooper, D.C. (1999). Neonatal Borna disease virus infection in the rat causes a loss of Purkinje cells in the cerebellum. Journal of NeuroVirology, 5, 181–189.
- Encinas-Nagel, N., Enderlein, D., Piepenbring, A., Herden, C., Heffels-Redmann, U., Felippe, P.A., Arns, C., Hafez, H.M. & Lierz, M. (2014). Avian bornavirus in free-ranging psittacine birds, Brazil. Emerging Infectious Diseases, 20, 2103–2106.
- Furrer, E., Bilzer, T., Stitz, L. & Planz, O. (2001). Neutralizing antibodies in persistent Borna disease virus infection: prophylactic effect of gp94-specific monoclonal antibodies in preventing encephalitis. Journal of Virology, 75, 943–951.
- Gancz, A.Y., Kistler, A.L., Clubb, S., Skewes-Cox, P., Fischer, K., Sorber, K., Chiu, C.Y., Lublin, A., Mechani, S., Farnoushi, Y., Greninger, A., Wen, C.C., Karlene, S.B., Ganem, D. & Derisi, J.L. (2009). Divergent Borna viruses are associated with proventricular dilatation disease. Proceedings of the 10th European Association of Avian Veterinarians (EAAV) Annual Conference (pp. 147–154), Antwerpen.
- Gancz, A.Y., Kistler, A.L., Greninger, A.L., Farnoushi, Y., Mechani, S., Perl, S., Berkowitz, A., Perez, N., Clubb, S., DeRisi, J.L., Ganem, D. & Lublin, A. (2009). Experimental induction of proventricular dilatation disease in cockatiels (Nymphicus hollandicus) inoculated with brain homogenates containing avian bornavirus 4. Virology Journal, 6, 100.
- Gray, P., Hoppes, S., Suchodolski, P., Mirhosseini, N., Payne, S., Villanueva, I., Shivaprasad, H.L., Honkavuori, K.S., Briese, T., Reddy, S.M. & Tizard, I. (2010). Use of avian bornavirus isolates to induce proventricular dilatation disease in conures. Emerging Infectious Diseases, 16, 473–479.
- Hallensleben, W., Schwemmle, M., Hausmann, J., Stitz, L., Volk, B., Pagenstecher, A. & Staeheli, P. (1998). Borna disease virus-induced neurological disorder in mice: infection of neonates results in immunopathology. Journal of Virology, 72, 4379–4386.
- Hameed, S.S., Guo, J., Tizard, I., Shivaprasad, H.L. & Payne, S. (2018). Studies on immunity and immunopathogenesis of parrot bornaviral disease in cockatiels. Virology, 515, 81–91.
- Heckmann, J., Enderlein, D., Gartner, A.M., Bücking, B., Malberg, S., Herzog, S., Heffels-Redmann, U., Herden, C. & Lierz, M. (2017, March 25–29). Investigations of natural transmission routes of a parrot bornavirus-4 isolate (PaBV-4) in cockatiels. 3rd International Conference on Avian, Herpetological and Exotic Mammal Medicine (ICARE), Venice, Italy (pp. 656–657).
- Heffels-Redmann, U., Enderlein, D., Herzog, S., Herden, C., Piepenbring, A., Neumann, D., Müller, H., Capelli, S., Müller, H., Oberhäuser, K., Gerlach, H., Kaleta, E.F. & Lierz, M. (2011). Occurrence of avian bornavirus infection in captive psittacines in various European countries and its association with proventricular dilatation disease. Avian Pathology, 40, 419–426.
- Herden, C., Herzog, S., Wehner, T., Zink, C., Richt, J.A. & Frese, K. (1999). Comparison of different methods of diagnosing Borna disease in horses post mortem. In U. Wernery, J. Wade, J.A. Mumford & O.R. Kaaden (Eds.), Equine Infectious Diseases 8th edn (pp. 286–290). Newmarket: R&W Publications.
- Herzog, S., Enderlein, D., Heffels-Redmann, U., Piepenbring, A., Neumann, D., Kaleta, E.F., Müller, H., Lierz, M. & Herden, C. (2010). Indirect immunofluorescence assay for intra vitam diagnosis of avian bornavirus infection in psittacine birds. Journal of Clinical Microbiology, 48, 2282–2284.
- Herzog, S., Kompter, C., Frese, K. & Rott, R. (1984). Replication of Borna disease virus in rats: age-dependent differences in tissue distribution. Medical Microbiolgy and Immunology, 173, 171–177.
- Hirano, N., Kao, M. & Ludwig, H. (1983). Persistent, tolerant or subacute infection in Borna disease virus-infected rats. Journal of General Virology, 64, 1521–1530.
- Hoffmann, B., Tappe, D., Höper, D., Herden, C., Boldt, A., Mawrin, C., Niederstraßer, O., Müller, T., Jenckel, M., van der Grinten, E., Lutter, C., Abendroth, B., Teifke, J.P., Cadar, D., Schmidt-Chanasit, J., Ulrich, R.G. & Beer, M. (2015). A variegated squirrel bornavirus associated with fatal human encephalitis. New England Journal of Medicine, 373, 154–162.
- Högemann, C., Richter, R., Korbel, R. & Rinder, M. (2017). Plasma protein, haematologic and blood chemistry changes in African grey parrots (Psittacus erithacus) experimentally infected with bornavirus. Avian Pathology, 46, 556–570.
- Honkavuori, K.S., Shivaprasad, H.L., Williams, B.L., Quan, P.L., Hornig, M., Street, C., Palacios, G., Hutchison, S.K., Franca, M., Egholm, M., Briese, T. & Lipkin, W.I. (2008). Novel Borna virus in psittacine birds with proventricular dilatation disease. Emerging Infectious Diseases, 14, 1883–1886.
- Hoppes, S., Gray, P.L., Payne, S., Shivaprasad, H.L. & Tizard, I. (2010). The isolation, pathogenesis, diagnosis, transmission, and control of avian bornavirus and proventricular dilatation disease. Veterinary Clinics of North America – Exotic Animal Practice, 13, 495–508.
- Hornig, M., Weissenböck, H., Horscroft, N. & Lipkin, W.I. (1999). An infection-based model of neurodevelopmental damage. Proceedings of the National Academy of Sciences of the United States of America, 96, 12102–12107.
- Kerski, A., de Kloet, A.H. & de Kloet, S.R. (2012). Vertical transmission of avian bornavirus in Psittaciformes: avian bornavirus RNA and anti-avian bornavirus antibodies in eggs, embryos, and hatchlings obtained from infected sun conures (Aratinga solstitialis). Avian Diseases, 56, 471–478.
- Kistler, A.L., Smith, J.M., Greninger, A.L., DeRisi, J.L. & Ganem, D. (2010). Analysis of naturally occurring avian bornavirus infection and transmission during an outbreak of proventricular dilatation disease among captive psittacine birds. Journal of Virology, 84, 2176–2179.
- Lierz, M., Hafez, H.M., Honkavuori, K.S., Gruber, A.D., Olias, P., Abdelwhab, E.M., Kohls, A., Lipkin, W.I. & Briese, T. (2009). Anatomical distribution of ABV in parrots, its occurrence in clinical healthy birds and ABV-antibody detection. Avian Pathology, 38, 491–496.
- Lierz, M., Herden, C., Herzog, S. & Piepenbring, A. (2010). Die neuropathische Drüsenmagendilatation der Psittaziden und das aviare Bornavirus als potenzielle Ursache. Tierarztliche Praxis Ausgabe K: Kleintiere – Heimtiere, 38, 87–94.
- Lierz, M., Piepenbring, A., Herden, C., Oberhäuser, K., Heffels-Redmann, U. & Enderlein, D. (2011). Vertical transmission of avian bornavirus in psittacines. Emerging Infectious Diseases, 17, 2390–2391.
- Mannl, A., Gerlach, H. & Leipold, R. (1987). Neuropathic gastric dilatation in Psittaciformes. Avian Diseases, 31, 214–221.
- Mirhosseini, N., Gray, P.L., Hoppes, S., Tizard, I., Shivaprasad, H.L. & Payne, S. (2011). Proventricular dilatation disease in cockatiels (Nymphicus hollandicus) after infection with a genotype 2 avian bornavirus. Journal of Avian Medicine and Surgery, 25, 199–204.
- Narayan, O., Herzog, S., Frese, K., Scheefers, H. & Rott, R. (1983a). Behavioral disease in rats caused by immunopathological responses to persistent Borna virus in the brain. Science, 220, 1401–1403.
- Narayan, O., Herzog, S., Frese, K., Scheefers, H. & Rott, R. (1983b). Pathogenesis of Borna disease in rats: immune-mediated viral ophthalmoencephalopathy causing blindness and behavioral abnormalities. Journal of Infectious Diseases, 148, 305–315.
- Nöske, K., Bilzer, T., Planz, O. & Stitz, L. (1998). Virus-specific CD4+ T cells eliminate Borna disease virus from the brain via induction of cytotoxic CD8+ T cells. Journal of Virology, 72, 4387–4395.
- Piepenbring, A.K., Enderlein, D., Herzog, S., Al-Ibadi, B., Heffels-Redmann, U., Heckmann, J., Lange-Herbst, H., Herden, C. & Lierz, M. (2016). Parrot bornavirus (PaBV)-2 isolate causes different disease patterns in cockatiels than PaBV-4. Avian Pathology, 45, 156–168.
- Piepenbring, A.K., Enderlein, D., Herzog, S., Kaleta, E.F., Heffels-Redmann, U., Ressmeyer, S., Herden, C. & Lierz, M. (2012). Pathogenesis of avian bornavirus in experimentally infected cockatiels. Emerging Infectious Diseases, 18, 234–241.
- Planz, O. & Bilzer, T. (1995). Immunopathogenic role of T-cell subsets in Borna disease virus-induced progressive encephalitis. Journal of Virology, 69, 896–903.
- Pletnikov, M.V., Moran, T.H. & Carbone, K.M. (2002). Borna disease virus infection of the neonatal rat: developmental brain injury model of autism spectrum disorders. BioScience, 1, 80–89.
- Pletnikov, M.V., Rubin, S.A., Schwartz, G.J., Moran, T.H., Sobotka, T.J. & Carbone, K.M. (1999). Persistent neonatal Borna disease virus (BDV) infection of the brain causes chronic emotional abnormalities in adult rats. Physiology & Behavior, 66, 823–831.
- Raghav, R., Taylor, M., DeLay, J., Ojkic, D., Pearl, D.L., Kistler, A.L., DeRisi, J.L., Ganem, D. & Smith, D.A. (2010). Avian bornavirus is present in many tissues of psittacine birds with histopathologic evidence of proventricular dilatation disease. Journal of Veterinary Diagnostic Investigation, 22, 495–508.
- Richt, J.A., Schmeel, A., Frese, K., Carbone, K.M., Narayan, O. & Rott, R. (1994). Borna disease virus-specific T cells protect against or cause immunopathological Borna disease. Journal of Experimental Medicine, 179, 1467–1473.
- Rinder, M., Ackermann, A., Kempf, H., Kaspers, B., Korbel, R. & Staeheli, P. (2009). Broad tissue and cell tropism of avian bornavirus in parrots with proventricular dilatation disease. Journal of Virology, 83, 5401–5407.
- Rott, R., Herzog, S., Richt, J. & Stitz, L. (1988). Immune-mediated pathogenesis of Borna disease. Zentralbl Bakteriol Mikrobiol Hyg A, 270, 295–301.
- Rubbenstroth, D., Brosinski, K., Rinder, M., Olbert, M., Kaspers, B., Korbel, R. & Staeheli, P. (2014). No contact transmission of avian bornavirus in experimentally infected cockatiels (Nymphicus hollandicus) and domestic canaries (Serinus canaria forma domestica). Veterinary Microbiology, 172, 146–156.
- Rubin, S.A., Waltrip II, R.W., Bautista, J.R. & Carbone, K.M. (1993). Borna disease virus in mice: host-specific differences in disease expression. Journal of Virology, 67, 548–552.
- Schlottau, K., Forth, L., Angstwurm, K., Höper, D., Zecher, D., Liesche, F., Hoffmann, B., Kegel, V., Seehofer, D., Platen, S., Salzberger, B., Liebert, U.G., Niller, H.-H., Schmidt, B., Matiasek, K., Riemenschneider, M.J., Brochhausen, C., Banas, B., Renders, L., Moog, P., Wunderlich, S., Seifert, C.L., Barreiros, A., Rahmel, A., Weiss, J., Tappe, D., Herden, C., Schmidt-Chanasit, J., Schwemmle, M., Rubbenstroth, D., Schlegel, J., Pietsch, C., Hoffmann, D., Jantsch, J., Beer, M. (2018). Fatal encephalitic Borna disease virus 1 in solid-organ transplant recipients. New England Journal of Medicine, 379, 1377–1379.
- Seto, F. (1981, September 1). Early development of the avian immune system. Poultry Science, 60, 1981–1995.
- Stitz, L., Bilzer, T. & Planz, O. (2002). The immunopathogenesis of Borna disease virus infection. Frontiers in Bioscience, 7, 541–555.
- Stitz, L., Nöske, K., Planz, O., Furrer, E., Lipkin, W.I. & Bilzer, T. (1998). A functional role for neutralizing antibodies in Borna disease: influence on virus tropism outside the central nervous system. Journal of Virology, 72, 8884–8892.
- Stitz, L., Soeder, D., Deschl, U., Frese, K. & Rott, R. (1989). Inhibition of immune-mediated meningoencephalitis in persistently Borna disease virus-infected rats by cyclosporine A. Journal of Immunology, 143, 4250–4256.
- Watanabe, M., Lee, B.J., Kamitani, W., Kobayashi, T., Taniyama, H., Tomonaga, K. & Ikuta, K. (2001). Neurological diseases and viral dynamics in the brains of neonatally Borna disease virus-infected gerbils. Virology, 282, 65–76.
- Watanabe, M., Lee, B.J., Yamashita, M., Kamitani, W., Kobayashi, T., Tomonaga, K. & Ikuta, K. (2003). Borna disease virus induces acute fatal neurological disorders in neonatal gerbils without virus- and immune-mediated cell destructions. Virology, 310, 245–253.
- Wüst, E.M. (2017). Untersuchungen zur vertikalen Übertragung des Parrot Bornavirus bei Psittaziden (Dissertation). Justus Liebig University Gießen.