ABSTRACT
Coccidiosis, caused by Eimeria species parasites, remains a major threat to poultry production, undermining economic performance and compromising welfare. The recent characterization of three new Eimeria species that infect chickens has highlighted that many gaps remain in our knowledge of the biology and epidemiology of these parasites. Concerns about the use of anticoccidial drugs, widespread parasite drug resistance, the need for vaccines that can be used across broiler as well as layer and breeder sectors, and consumer preferences for “clean” farming, all point to the need for novel control strategies. New research tools including vaccine delivery vectors, high throughput sequencing, parasite transgenesis and sensitive molecular assays that can accurately assess parasite development in vitro and in vivo are all proving helpful in the ongoing quest for improved cost-effective, scalable strategies for future control of coccidiosis.
Eimeria, cause of the disease coccidiosis
Eimeria are protozoan parasites that can cause coccidiosis in all livestock and poultry, in addition to many other non-production species. Most Eimeria species are strictly limited to a single host-species, although examples such as Eimeria innocua from domestic turkeys can also replicate in grey partridge and bobwhite quail (Vrba & Pakandl, Citation2015). Seven Eimeria species have long been recognized to infect chickens, appearing on every continent where chickens are farmed (Clark et al., Citation2016). Additionally, three cryptic Eimeria genotypes have recently been identified as new species (Cantacessi et al., Citation2008; Blake et al., Citation2021). At least five Eimeria species infect turkeys, although their taxonomy remains a topic of debate (Vrba & Pakandl, Citation2014; El-Sherry et al., Citation2015). While mammalian coccidiosis is a notable problem, disease in chickens is commonly considered to be most significant (Blake, Knox, et al., Citation2020).
Coccidiosis in chickens can manifest as a haemorrhagic disease, usually associated with E. tenella, E. necatrix or E. brunetti, or malabsorptive disease caused by E. maxima, E. acervulina, E. mitis or E. praecox (Reid et al., Citation2014). All seven species can be harmful, with E. necatrix and E. tenella considered to be most pathogenic (Long et al., Citation1976; Williams et al., Citation2009). Eimeria acervulina, E. maxima and E. tenella are usually most prevalent (Haug et al., Citation2008; Kumar et al., Citation2014; Clark, et al., Citation2016; Hauck et al., Citation2019). Coccidiosis is most common when husbandry is poor, and is exacerbated by factors such as high stocking density, poor litter quality and high humidity.
Impact
Eimeria can affect chicken production and welfare. The nominal financial economic cost of coccidiosis in chickens was recently estimated to have exceeded UK £10.4 billion worldwide in 2016 (equivalent to ∼ €12 billion or US $14.5 billion), including costs of morbidity, mortality and control (Blake, Knox, et al., Citation2020). Morbidity, including poor bodyweight gain and food conversion ratios, represented the biggest cost component, varying from 51% to 90% of the total cost between countries. The wider costs of Eimeria infection are difficult to quantify. Eimeria contribute to enteric dysbiosis, influencing microbiome diversity and structure (Macdonald et al., Citation2017), and can also influence carriage of foodborne zoonotic pathogens including Salmonella Typhimurium and Campylobacter jejuni (Baba et al., Citation1982; Macdonald et al., Citation2019). Eimeria infection is well recognized as a major risk factor for necrotic enteritis, caused by Clostridium perfringens, independently estimated to cost the poultry production industry more than US$ 6 billion (Wade & Keyburn, Citation2015). The welfare consequences of coccidiosis are also considerable. In addition to the direct results of disease, the consequent diarrhoea reduces litter quality, contributing to footpad dermatitis and negatively impacting on overall welfare and technical performance (Abd El-Wahab et al., Citation2012; de Jong et al., Citation2014).
Current approaches available for control of coccidiosis
Modern production systems for chickens rely on control of Eimeria. Good husbandry is important, but frequently insufficient without routine chemoprophylaxis or vaccination. Several sulphonamides were used as anticoccidial drugs from the 1940s, followed by the introduction of a series of synthetic and then ionophore products (). Diclazuril was the last anticoccidial drug to be introduced in 1990, and no new active ingredients are currently close to market. Now, increasing public and legislative pressure is discouraging use of chemoprophylaxis in many production systems. Several anticoccidial drugs have been banned in areas such as the European Union (Squadrone et al., Citation2008), and demand for “No antibiotics, ever” products in the USA, where ionophores are regulated as antibiotics, is driving real reduction.
Figure 1. Expansion of global chicken production and products used to control Eimeria. Figure adapted from Reid (Citation1990). The number of chickens produced each year downloaded from FAOSTAT (Citation2021). The coloured boxes indicate the periods when new sulphonamide (‘Sulpha', orange), synthetic (green) and ionophore (red) drugs were introduced to control Eimeria in chickens (Reid, Citation1990; Chapman, Citation1997). The hollow box indicates the period during which no new anticoccidial drugs have been introduced. Blue arrows indicate the introduction of the first non-attenuated (wild-type) and attenuated anticoccidial vaccines. *Introduction of a ban on antimicrobial growth promoters in Sweden (Wierup, Citation2001). #Introduction of a ban on anticoccidial drugs such as amprolium as prophylactic food additives in the European Union (Squadrone et al., Citation2008). Colour online.
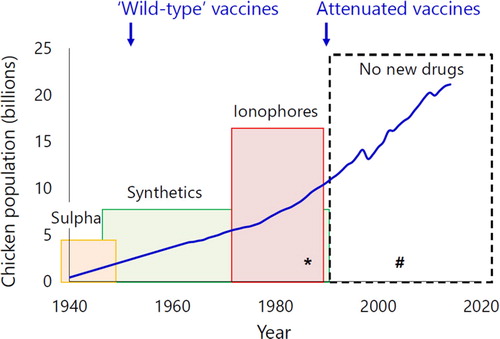
Alternative approaches to control Eimeria include use of live parasite vaccines (). The first anticoccidial vaccines included live, fully virulent Eimeria oocysts representing one or more species (reviewed by Williams, Citation2002). The Eimeria used in these vaccines are considered “wild-type” (i.e. unmodified since collection from the field), although the long period of time since isolation for some has prompted use of the name “non-attenuated”, given the likelihood for divergence from modern field populations. Live vaccines are relatively complex, requiring multiple Eimeria species and, in some cases, strains because immunity induced by Eimeria infection is parasite species/strain-specific. Strain-specific immunity has been described for E. acervulina, E. maxima, E. mitis and E. tenella (Blake, Worthing, et al., Citation2020), although only E. maxima has required more than one strain in some vaccine formulations. These wild-type or non-attenuated live vaccines can be highly effective and relatively cheap to produce, but risk compromising flock performance and occurrence of clinical disease if managed incorrectly (Blake, Knox, et al., Citation2020; Shirley et al., Citation2005). Non-attenuated vaccines are used widely across much of North America, as well as in parts of Africa and Asia. Recognizing the risks posed by non-attenuated vaccines, a second generation of live-attenuated vaccines was first developed in the 1980s, using Eimeria lines modified by selection for precocious (i.e. abbreviated) development to attenuate pathogenicity while retaining immunogenicity (Shirley et al., Citation2005). Attenuated vaccines are used extensively in much of Europe, as well as parts of Africa, Asia and Australasia, primarily with layer and breeder stock owing to their relative cost and limited productive capacity (Blake, Knox, et al., Citation2020).
Strategies for the use of live anticoccidial vaccines are evolving rapidly. Demand for “No antibiotics, ever” poultry products in the USA is encouraging vaccination, using non-attenuated anticoccidial vaccines in place of chemoprophylaxis. The widespread occurrence of anticoccidial drug resistance is also driving demand for alternatives. Recent studies have demonstrated that multiple rounds of vaccination using drug-susceptible live vaccine formulations can reduce drug resistance in Eimeria field populations, likely replacing or hybridizing with resident strains and permitting a subsequent return to chemoprophylaxis (Chapman & Jeffers, Citation2015). The relative risk posed by use of non-attenuated vaccines can be managed using a bioshuttle approach, where vaccinated chicks receive grower and finisher diets containing anticoccidial drugs to limit parasite recycling and prevent vaccine-associated safety issues (Kimminau & Duong, Citation2019). Combined, these new management strategies to control coccidiosis have resulted in vaccination of ∼35% of all broilers sold in the USA over the last five years (US industry data, unpublished). Previously, anticoccidial vaccine uptake was negligible in the US broiler sector, as remains the case in Europe today.
In addition to drugs and vaccines, a wide range of natural herbs and other botanicals or their extracts, organic acids, immunomodulators and complex carbohydrates, probiotics and prebiotics are now being developed to control coccidiosis (Khater et al., Citation2020). Genetic mapping strategies are also being applied to inform selective breeding of elite commercial chicken lines towards improved resistance against infection and pathology (Hamzic et al., Citation2015; Boulton et al., Citation2018).
Knowledge gaps, challenges and opportunities
Access to scalable and cost-effective vaccines that can be deployed across different countries and poultry sectors remains a significant challenge. The recent dramatic increase in anticoccidial vaccine use in US broiler production has provided evidence that existing live vaccines can be scaled up and provide an alternative to anticoccidial drugs. The vaccines being used are non-attenuated and cost ∼0.5 pence (UK) per dose. However, the live-attenuated parasite lines used in European vaccines are considerably less fecund, hindering up-scaling, and currently cost between 3 and 8 pence per dose in the UK (Blake, Knox, et al., Citation2020). Disruption in the supply of specific pathogen-free chickens used in vaccine production can also create bottlenecks in vaccine availability. In the absence of change to regulatory and consumer requirements, alternatives are still required. Considerable progress has been made towards identification of immunoprotective antigens that can be used in recombinant or vectored vaccines, but none has made it to market (Blake et al., Citation2017). One major constraint in deployment of such antigen-specific vaccines is an appropriate and effective delivery system. Several possible vectors for oral administration, including Bacillus, Salmonella, transgenic Eimeria and yeasts such as Saccharomyces cerevisiae, are currently in development and could be appropriate (Hoelzer et al., Citation2018; Pastor-Fernández et al., Citation2020). Extension of these technologies to improve vaccines for turkeys will also be required. Establishment of effective oral vaccine vector systems can also support vaccination against other key pathogens of poultry.
Another major challenge remains our limited understanding of genetic diversity and population structure for Eimeria. Remarkably little is known about naturally existing diversity beyond a few well-established markers such as the rRNA genes and cytochrome C oxidase subunit I (COI) (Blake et al., Citation2015). Improving understanding of existing genetic diversity and mechanisms of genome evolution may become fundamental to safeguard efficacy of the remaining anticoccidial drugs and future recombinant vaccines. New sequencing technologies, including the high-throughput Illumina platform and long-read systems such as PacBio and Oxford Nanopore, are quickly becoming cheaper and more accessible. Application of these tools to Eimeria will improve our knowledge of species occurrence, diversity and evolution (Blake et al., Citation2015; Clark et al., Citation2016; Hinsu et al., Citation2018; Hauck et al., Citation2019). The opacity of Eimeria population genetics has recently been emphasized by the characterization of three new Eimeria species that infect chickens. Previously identified as operational taxonomic units (OTUs) x, y and z (Cantacessi et al., Citation2008), recent studies of morphology, pathology, genetics and phylogeny suggest these parasites warrant distinct species status and they have been named Eimeria lata, Eimeria nagambie and Eimeria zaria (Blake et al., Citation2021). It is notable that all three new species escape immune inhibition in chickens vaccinated with current anticoccidial vaccines from Europe and Australia, indicating a new coccidiosis risk as producers reduce their reliance on routine use of broad-spectrum anticoccidial drugs and rely much more on species-specific vaccines. The development of new and modified live parasite vaccines including these new species may therefore be required.
Most Eimeria are host- and tissue-specific in their development and this has hindered their study in in vitro systems (Marugan-Hernandez et al., Citation2020), complicating fundamental biological as well as commercial applications. Primary cultures of avian cells have been used to propagate the E. tenella life cycle, but the efficiency of this is far too low for purposes such as vaccine production. Replication in non-avian cell lines fails to support completion of the full life cycle. As new technologies and cell lines become available in vitro culture is being re-investigated (e.g. Bussiere et al., Citation2018). Improvements to in vitro culture offer opportunities to enhance biological understanding and could eventually contribute to vaccine production. Alongside this optimism, improved quantitative assays to measure parasite replication/gene expression and assess the longitudinal development of different life cycle stages in vitro offer opportunities to screen anticoccidial treatments before moving to studies in chickens (Marugan-Hernandez et al., Citation2020). New assays are also available for use in vivo, including sensitive species-specific quantitative PCR to measure parasite replication with higher throughput and greater reproducibility than traditional measures using microscopy (Nolan et al., Citation2015). Development of a tool kit for transient and stable genetic modification of Eimeria has complemented these advances, supporting studies of fundamental biology and vaccine development (Pastor-Fernández et al., Citation2019).
Conclusions
More than 72 billion chickens were produced globally in 2019, emphasizing their major contribution to the production of protein for human consumption. Production systems for chickens are increasingly moving towards drug-free and/or extensive programmes in much of Europe and North America, while production is intensifying rapidly in many low- and middle-income countries. These changes are raising a series of new challenges to control of pathogens such as Eimeria species parasites. Recent developments in technical resources, public perceptions and legislative controls are changing our understanding of Eimeria and the ways in which we attempt to control them. It is likely that the coming decade will witness further significant changes, with new challenges and opportunities for innovation.
Disclosure statement
No potential conflict of interest was reported by the authors.
References
- Abd El-Wahab, A., Visscher, C.F., Wolken, S., Reperant, J.-M., Beineke, A., Beyerbach, M. & Kamphues, J. (2012). Foot-pad dermatitis and experimentally induced coccidiosis in young turkeys fed a diet without anticoccidia. Poultry Science, 91, 627–635.
- Baba, E., Fukata, T. & Arakawa, A. (1982). Establishment and persistence of Salmonella typhimurium infection stimulated by Eimeria tenella in chickens. Research in Veterinary Science, 33, 95–98.
- Blake, D.P., Clark, E.L., Macdonald, S.E., Thenmozhi, V., Kundu, K., Garg, R., Jatau, I.D., Ayoade, S., Kawahara, F., Moftah, A., Reid, A.J., Adebambo, A.O., Álvarez Zapata, R., Srinivasa Rao, A.S.R., Thangaraj, K., Banerjee, P.S., Dhinakar-Raj, G., Raman, M. & Tomley, F.M. (2015). Population, genetic, and antigenic diversity of the apicomplexan Eimeria tenella and their relevance to vaccine development. Proceedings of the National Academy of Sciences, 112, E5343–E5350.
- Blake, D.P., Knox, J., Dehaeck, B., Huntington, B., Rathinam, T., Ravipati, V., Ayoade, S., Gilbert, W., Adebambo, A.O., Jatau, I.D., Raman, M., Parker, D., Rushton, J. & Tomley, F.M. (2020). Re-calculating the cost of coccidiosis in chickens. Veterinary Research, 51, 115.
- Blake, D.P., Pastor-Fernández, I., Nolan, M.J. & Tomley, F.M. (2017). Recombinant anticoccidial vaccines – a cup half full? Infection, Genetics and Evolution, 55, 358–365.
- Blake, D.P., Vrba, V., Xia, D., Jatau, I., Spiro, S., Nolan, M., Underwood, G., & Tomley, F.M. (2021). Genetic and biological characterisation of three cryptic Eimeria operational taxonomic units that infect chickens (Gallus gallus domesticus). International Journal for Parasitology. doi:10.1016/j.ijpara.2020.12.004
- Blake, D.P., Worthing, K. & Jenkins, M.C. (2020). Exploring Eimeria genomes to understand population biology: recent progress and future opportunities. Genes, 11, 1103. doi:10.3390/genes11091103.
- Boulton, K., Nolan, M.J., Wu, Z., Psifidi, A., Riggio, V., Harman, K., Bishop, S.C., Kaiser, P., Abrahamsen, M.S., Hawken, R., Watson, K.A., Tomley, F.M., Blake, D.P. & Hume, D.A. (2018). Phenotypic and genetic variation in the response of chickens to Eimeria tenella induced coccidiosis. Genetics Selection Evolution, 50, 63.
- Bussière, F.I., Niepceron, A., Sausset, A., Esnault, E., Silvestre, A., Walker, R.A., Smith, N.C., Quéré, P. & Laurent, F. (2018). Establishment of an in vitro chicken epithelial cell line model to investigate Eimeria tenella gamete development. Parasites & Vectors, 11, 44.
- Cantacessi, C., Riddell, S., Morris, G.M., Doran, T., Woods, W.G., Otranto, D. & Gasser, R.B. (2008). Genetic characterization of three unique operational taxonomic units of Eimeria from chickens in Australia based on nuclear spacer ribosomal DNA. Veterinary Parasitology, 152, 226–234.
- Chapman, H.D. (1997). Biochemical, genetic and applied aspects of drug resistance in Eimeria parasites of the fowl. Avian Pathology, 26, 221–244.
- Chapman, H.D. & Jeffers, T.K. (2015). Restoration of sensitivity to salinomycin in Eimeria following 5 flocks of broiler chickens reared in floor-pens using drug programs and vaccination to control coccidiosis. Poultry Science, 94, 943–946.
- Clark, E.L., Macdonald, S.E., Thenmozhi, V., Kundu, K., Garg, R., Kumar, S., Ayoade, S., Fornace, K.M., Jatau, I.D., Moftah, A., Nolan, M.J., Sudhakar, N.R., Adebambo, A.O., Lawal, I.A., Álvarez Zapata, R., Awuni, J.A., Chapman, H.D., Karimuribo, E., Mugasa, C.M., Namangala, B., Rushton, J., Suo, X., Thangaraj, K., Srinivasa Rao, A.S.R., Tewari, A.K., Banerjee, P.S., Dhinakar Raj, G., Raman, M., Tomley, F.M. & Blake, D.P. (2016). Cryptic Eimeria genotypes are common across the southern but not northern hemisphere. International Journal for Parasitology, 46, 537–544.
- de Jong, I., Gunnink, H. & van Harn, J. (2014). Wet litter not only induces footpad dermatitis but also reduces overall welfare, technical performance, and carcass yield in broiler chickens. Journal of Applied Poultry Research, 23, 51–58.
- El-Sherry, S., Ogedengbe, M.E., Hafeez, M.A., Sayf-Al-Din, M., Gad, N. & Barta, J.R. (2015). Sequence-based genotyping clarifies conflicting historical morphometric and biological data for 5 Eimeria species infecting turkeys. Poultry Science, 94, 262–272.
- FAOSTAT. (2021). Food and Agriculture Organization of the United Nations FAOSTAT database.
- Hamzić, E., Buitenhuis, B., Hérault, F., Hawken, R., Abrahamsen, M.S., Servin, B., Elsen, J.-M., Pinard - van der Laan, M.-H. & Bed’Hom, B. (2015). Genome-wide association study and biological pathway analysis of the Eimeria maxima response in broilers. Genetics Selection Evolution, 47, 91.
- Hauck, R., Carrisosa, M., McCrea, B.A., Dormitorio, T. & Macklin, K.S. (2019). Evaluation of next-generation amplicon sequencing to identify Eimeria spp. of chickens. Avian Diseases, 63, 577–583.
- Haug, A., Gjevre, A.G., Thebo, P., Mattsson, J.G. & Kaldhusdal, M. (2008). Coccidial infections in commercial broilers: epidemiological aspects and comparison of Eimeria species identification by morphometric and polymerase chain reaction techniques. Avian Pathology, 37, 161–170.
- Hinsu, A.T., Thakkar, J.R., Koringa, P.G., Vrba, V., Jakhesara, S.J., Psifidi, A., Guitian, J., Tomley, F.M., Rank, D.N., Raman, M., Joshi, C.G. & Blake, D.P. (2018). Illumina next generation sequencing for the analysis of Eimeria populations in commercial broilers and indigenous chickens. Frontiers in Veterinary Science, 5, 176.
- Hoelzer, K., Bielke, L., Blake, D.P., Cox, E., Cutting, S.M., Devriendt, B., Erlacher-Vindel, E., Goossens, E., Karaca, K., Lemiere, S., Metzner, M., Raicek, M., Collell Suriñach, M., Wong, N.M., Gay, C. & Van Immerseel, F. (2018). Vaccines as alternatives to antibiotics for food producing animals. Part 2: new approaches and potential solutions. Veterinary Research, 49, 70.
- Khater, H.F., Ziam, H., Abbas, A., Abbas, R., Raza, M., Hussain, K., Younis, E.Z., Radwan, I.T., & Selim, A. (2020). Avian coccidiosis: recent advances in alternative control strategies and vaccine development. Agrobiological Records, 1, 11–25.
- Kimminau, E.A. & Duong, T.T. (2019). Longitudinal response of commercial broiler operations to bio-shuttle administration. Journal of Applied Poultry Research, 28, 1389–1397.
- Kumar, S., Garg, R., Moftah, A., Clark, E.L., Macdonald, S.E., Chaudhry, A.S., Sparagano, O., Banerjee, P.S., Kundu, K., Tomley, F.M. & Blake, D.P. (2014). An optimised protocol for molecular identification of Eimeria from chickens. Veterinary Parasitology, 199, 24–31.
- Long, P.L., Joyner, L., Millard, B. & Norton, C. (1976). A guide to laboratory techniques used in the study and diagnosis of avian coccidiosis. Folia Veterinaria Latina, 6, 201–217.
- Macdonald, S.E., Nolan, M.J., Harman, K., Boulton, K., Hume, D.A., Tomley, F.M., Stabler, R.A., & Blake, D.P. (2017). Effects of Eimeria tenella infection on chicken caecal microbiome diversity, exploring variation associated with severity of pathology. PLoS One, 12, e0184890.
- Macdonald, S.E., van Diemen, P.M., Martineau, H., Stevens, M.P., Tomley, F.M., Stabler, R.A., & Blake, D.P. (2019). Impact of Eimeria tenella coinfection on Campylobacter jejuni colonization of the chicken. Infection & Immunity, 87, e00772-18.
- Marugan-Hernandez, V., Jeremiah, G., Aguiar-Martins, K., Burrell, A., Vaughan, S., Xia, D., Randle, N. & Tomley, F. (2020). The growth of Eimeria tenella: characterization and application of quantitative methods to assess sporozoite invasion and endogenous development in cell culture. Frontiers in Cellular and Infection Microbiology, 10, 579833.
- Nolan, M.J., Tomley, F.M., Kaiser, P. & Blake, D.P. (2015). Quantitative real-time PCR (qPCR) for Eimeria tenella replication – implications for experimental refinement and animal welfare. Parasitology International, 64, 464–470.
- Pastor-Fernández, I., Kim, S., Marugan-Hernandez, V., Soutter, F., Tomley, F.M. & Blake, D.P. (2020). Vaccination with transgenic Eimeria tenella expressing Eimeria maxima AMA1 and IMP1 confers partial protection against high-level E. maxima challenge in a broiler model of coccidiosis. Parasites & Vectors, 13, 343.
- Pastor-Fernández, I., Pegg, E., Macdonald, S.E., Tomley, F.M., Blake, D.P. & Marugan-Hernandez, V. (2019). Laboratory growth and genetic manipulation of Eimeria tenella. Current Protocols in Microbiology, 53, e81.
- Reid, A.J., Blake, D.P., Ansari, H.R., Billington, K., Browne, H.P., Bryant, J., Dunn, M., Hung, S.S., Kawahara, F., Miranda-Saavedra, D., Malas, T.B., Mourier, T., Naghra, H., Nair, M., Otto, T.D., Rawlings, N.D., Rivailler, P., Sanchez-Flores, A., Sanders, M., Subramaniam, C., Tay, Y.-L., Woo, Y., Wu, X., Barrell, B., Dear, P.H., Doerig, C., Gruber, A., Ivens, A.C., Parkinson, J., Rajandream, M.-A., Shirley, M.W., Wan, K.-L., Berriman, M., Tomley, F.M. & Pain, A. (2014). Genomic analysis of the causative agents of coccidiosis in domestic chickens. Genome Research, 24, 1676–1685.
- Reid, W.M. (1990). History of avian medicine in the United States. X. Control of coccidiosis. Avian Diseases, 34, 509–525.
- Shirley, M.W., Smith, A.L. & Tomley, F.M. (2005). The biology of avian Eimeria with an emphasis on their control by vaccination. Advances in Parasitology, 60, 285–330.
- Squadrone, S., Mauro, C., Ferro, G.L., Amato, G. & Abete, M.C. (2008). Determination of amprolium in feed by a liquid chromatography-mass spectrometry method. Journal of Pharmaceutical and Biomedical Analysis, 48, 1457–1461.
- Vrba, V. & Pakandl, M. (2014). Coccidia of turkey: from isolation, characterisation and comparison to molecular phylogeny and molecular diagnostics. International Journal for Parasitology, 44, 985–1000.
- Vrba, V. & Pakandl, M. (2015). Host specificity of turkey and chicken Eimeria: controlled cross-transmission studies and a phylogenetic view. Veterinary Parasitology, 208, 118–124.
- Wade, B. & Keyburn, A. (2015). The true cost of necrotic enteritis. World Poultry, 31, 16–17.
- Wierup, M. (2001). The Swedish experience of the 1986 year ban of antimicrobial growth promoters, with special reference to animal health, disease prevention, productivity, and usage of antimicrobials. Microbial Drug Resistance, 7, 183–190.
- Williams, R.B. (2002). Fifty years of anticoccidial vaccines for poultry (1952–2002). Avian Diseases, 46, 775–802.
- Williams, R.B., Marshall, R.N., Pages, M., Dardi, M. & del Cacho, E. (2009). Pathogenesis of Eimeria praecox in chickens: virulence of field strains compared with laboratory strains of E. praecox and Eimeria acervulina. Avian Pathology, 38, 359–366.