ABSTRACT
H9N2 low-pathogenic avian influenza (LPAI) viruses have long been circulating in the world poultry industry, resulting in substantial economic losses. In addition to bird health consequences, viruses from specific lineages such as G1 and Y280 are also known to have the potential to cause a pandemic within the human population. In South Korea, after introducing inactivated H9N2 vaccines in 2007, there were no field outbreaks of H9N2 LPAI since 2009. However, in June 2020, an H9N2 virus was isolated from an outbreak in a Korean chicken farm. This strain was distinct from the predominant Korean/Y439 lineage and was believed to be part of the Y280-like lineage. Since the first case of this new H9N2 LPAI, nine more cases of field infections in poultry farms were documented through July and December of 2020. Phylogenetic analysis of the haemagglutinin (HA) and neuraminidase genes of these case isolates revealed that all strains were grouped with exotic Y280-like strains that did not previously exist in South Korea and were emerging into a new cluster. Serological assays also confirmed the existence of antibodies to Y280-like viruses in field sera collected from infected birds, and that they had seroconverted. Further analysis of the receptor-binding region in the HA protein also revealed that these isolates harboured a human-like motif that could potentially affect mammals and humans, demonstrating a possible public health risk. This is the first report of field cases caused by Y280-like H9N2 LPAI in the Korean poultry industry.
RESEARCH HIGHLIGHTS
Field outbreaks caused by Y280-like H9N2 avian influenza viruses were confirmed.
A human-like motif was found at the HA receptor-binding region of all isolates.
Introduction
Low-pathogenic avian influenza (LPAI) H9N2 viruses have been widely endemic in terrestrial gallinaceous poultry throughout the Eurasian continent since the mid-1990s (Alexander, Citation2007).
Since its first report in 1966 (Homme & Easterday, Citation1970), up to this date, there are several recognized genetic lineages of H9N2 (Guo et al., Citation2000; Pusch & Suarez, Citation2018): the G1 lineage (Guan et al., Citation2000), Korean/Y439-like lineage (Lee et al., Citation2000), Y280-like lineage (Guan et al., Citation1999), and a European lineage predominantly circulating in turkeys (Śmietanka et al., Citation2014; Reid et al., Citation2016; Blaurock et al., Citation2020). In addition to bird health consequences, the G1 and Y280-like lineages have the potential of causing a pandemic in the human population due to their biological properties, such as interspecies transmission and affinity for the human receptor-binding profile (Matrosovich et al., Citation2001; Choi et al., Citation2004; Kimble et al., Citation2010). Subsequent to the first report on H9N2 in South Korea in 1996 (Mo et al., Citation2003) the virus became endemic in the Korean poultry industry, eventually forming the distinct Korean lineage that shares close genetic relationships with the Y439-like lineage (Lee, et al., Citation2000). Although the Korean government mainly focused on stamping-out and compensation policies for disease control in the poultry sector during the LPAI H9N2 outbreaks in the late 1990s, an inactivated oil adjuvant vaccine based on the A/chicken/Korea/01310/2001 strain, which was later designated as part of Korean Clade A (Lee et al., Citation2016), was approved for use to control the endemically circulating H9N2 LPAI in 2007 (Choi et al., Citation2008; Mo et al., Citation2016). Since then, Clade A viruses were well controlled by vaccine-induced immunity until now, reducing the overall incidence of H9N2 LPAI in South Korea. No field infection cases of H9N2 LPAI were reported between 2009 and 2020 (Cho et al., Citation2020).
In June 2020, the existence of Y280-like H9N2 strains (that were distinct from previous Y439-like H9N2 strains that have been circulating since 1996) was confirmed by the government due to active surveillance in live bird markets (LBM), which were known epicentres for H9N2 LPAI in South Korea (APQA, Citation2020; Youk et al., Citation2020). Based on the descriptive characteristics of these new H9N2 strains in the report, no clinical signs or mortalities were observed in experimentally infected birds, and higher quantities of the virus shed through the oropharyngeal route compared to the cloacal route (APQA, Citation2020). No field cases involving these strains were confirmed at the time of the report.
However, new outbreaks of H9N2 started to occur in South Korean chicken farms in the summer of 2020. Beginning with a single field case in June, several more cases were reported throughout July and December, indicating the emergence of this new H9N2 LPAI. Like the governmental reports, these H9N2 isolates were different from the predominant Korean/Y439-like lineage and were believed to be part of the Y280-like lineage. This is the first documentation of field cases caused by the Y280-like H9N2 LPAI in chicken farms in South Korea. The primary focus of this report was to provide descriptions of clinical and pathological findings associated with these strains and to genetically characterize the antigenic properties of these field isolates in an attempt to investigate their current epidemiology in the field.
Materials and methods
Background
At the end of June 2020, five dead layer chickens and serum samples from a commercial chicken farm (farm A) were submitted to avian diagnostics company, Avinext LTD. The average age of the dead layers was 43 weeks. Increased mortality was observed on June 22nd, with 15 birds dead in a day. In two weeks, the flock had shown a decrease of 20% in egg production performance, and peritonitis was observed in field necropsies before case submission. This flock had a previous history of high mortality due to episodes of colibacillosis when relocating the chicks to layer facilities after the rearing phase. Post-mortem examinations of the dead layers confirmed laryngeal exudates, atrophic follicles, salpingitis, and peritonitis. Based on haemagglutination inhibitions (HI) assay, the serum samples were moderately seropositive (7 log2) for avian influenza virus (AIV). Eventually, H9N2 LPAI was confirmed by reverse transcription-polymerase chain reaction (RT–PCR) assay employed for virus detection from tissue homogenates (Fouchier et al., Citation2000). Genome sequencing revealed that this specific H9N2 strain, later designated as A/chicken/Korea/1116/2020 (accession number: MW570852) belonged to the Y280-like lineage, which was distinctively different from the Y439/Korean H9N2 lineage predominant in South Korea. After receiving the first case of a Y280-like H9N2 field infection, all cases subjected to necropsies were explicitly monitored for this new type of H9N2 LPAI. Since then, nine more cases of Y280-like H9N2 field infections were received and documented throughout July and December, which involved five layer farms (farms B, F, G, H, I), a layer pullet farm (farm C), two broiler farms (farms D and E) and a broiler-breeder farm (farm J) (). All case histories were provided either directly by producers or through case application forms.
Table 1. Case summary and detection of H9N2 strains from field cases.
Post-mortem examination and laboratory analysis
Post-mortem examination was performed in all submitted cases. Gross lesions were recorded, and appropriate specimens were collected for viral, bacterial, and light microscopic examination. Liver, peritoneal and intraorbital swabs were collected and cultured in MacConkey agar, blood agar, and chocolate agar for bacterial identification. Tracheal and caecal tonsil samples were collected and homogenized in phosphate-buffered saline (PBS) for virus isolation (Swayne et al., Citation2020). Briefly, supernatants collected from tracheal and caecal tonsil homogenates were treated with antibiotics (200U penicillin, 200 g streptomycin, amphotericin B 5 μg/ml) and inoculated into 9–10-day-old specific pathogen-free (SPF) embryonated chicken eggs by the allantoic sac as per standard procedures (Spackman & Killian, Citation2020). After the virus was passed twice in eggs, the allantoic fluid was collected for haemagglutination (HA) and RT–PCR assays based on methodologies described elsewhere (Fouchier, et al., Citation2000; Dufour-Zavala, Citation2008). For screening purposes, the routine virus-specific RT–PCR assays for infectious bronchitis (IB) virus (Mardani et al., Citation2006), haemagglutination inhibition (HI) assays for Newcastle disease (ND) virus and enzyme-linked immunosorbent assays (ELISA) (IDEXX Europe, Hoofddorp, The Netherlands) for avian metapneumoviruses (aMPV) were performed. Real-time RT–PCR screening for H5 and H7 highly pathogenic avian influenza (HPAI) was also implemented (Agüero et al., Citation2007; Sidoti et al., Citation2010). Organs confirmed with gross lesions were fixed in 10% neutralized formalin, trimmed, embedded in paraffin, sectioned at 4 µm, and stained with haematoxylin–eosin (HE) for histological examination.
Molecular characterization of field isolates and phylogenetic anaylsis
Briefly, total RNA was extracted from allantoic fluid collected from SPF embryonated chicken eggs that propagated the field H9N2 isolates using the Patho Gene-spin DNA/RNA Extraction Kit (iNtRON Biotechnology Inc., Seongnam, South Korea) following the manufacturer’s instructions. The extracted viral RNA was reverse transcribed to cDNA using the PrimeScript RT–PCR kit (Takara Korea Biomedical, Seoul, South Korea) with the Uni12 5′-AGCRAAAGCAGG-3′ primer (Hoffmann et al., Citation2001). The haemagglutinin gene was amplified based on the procedural conditions described by Hoffmann et al. (Citation2001). After electrophoresis at 135 V for 25 min, the PCR product was purified from 1.5% agarose gel using the Expin gel SV kit (GeneAll Biotechnology, Seoul, South Korea) and was sequenced by Cosmogentech (GeneAll Biotechnology). The HA and neuraminidase (NA) gene nucleotide sequences of the H9N2 field isolates were aligned using the CLC sequence 7 software (Qiagen, Germantown, MD, USA). A phylogenetic tree based on the HA and NA genes was constructed using the maximum likelihood (ML) method by Mega version 10 (http://www.megasoftware.net). The H9N2 field strains were typed based on the phylogenetic analysis of the HA and NA genes by using reference strains from previous studies and GenBank (https://www.ncbi.nlm.nih.gov/genbank/). To further understand the molecular features of the field isolates, deduced amino acids of the HA gene were analysed to determine cleavage and receptor-binding sites and predict potential N-glycosylation sites (PGSs).
Serology
HI assays were performed to evaluate the antigenic relatedness of the field case isolates with Y280-like lineage H9N2 LPAI based on standard protocols (Cross, Citation2002; OIE, Citation2019). Field sera collected from affected farms were tested with the Y439 H9N2 inactivated vaccine strain A/chicken/Korea/01310/2001 (Y439 antigen) and the Y280-like H9N2 A/Chicken/Korea/1116/2020 strain (isolate from first Y280-like H9N2 case, Y280 antigen) to quantify virus neutralizing antibodies. The Y439 and Y280 antigens were standardized to 4 HA units.
Statistical analysis
Two-way analysis of variance (2-way ANOVA), along with Tukey’s multiple-comparison tests, were applied for diagnostic data analysis including field sera titres using Excel and GraphPad Prism software (v.8.1; GraphPad Software, La Jolla, CA, USA). Proportions of birds positive for H9N2 antibodies were determined by Fisher’s exact test.
Results
Clinical and post-mortem examinations
A list of clinical signs reported by producers and veterinarians is provided in . Clinical signs such as mortality and respiratory signs, including coughing, sneezing, and gasping, were observed in chicken flocks of various ages and types. Signs of reduced egg production were observed in four out of six layer farms (farms A, B, F, I) that submitted cases. Among them, reductions in egg production of over 20% and 10% were observed in farms A and B (). Broiler-breeder farm J reported a more severe 27% decrease in egg production. Noticeable mortalities were mainly observed in broiler farms, with around 1000 birds dead in a single day at farm D and 200 birds dead for four consecutive days (800 birds in total) in farm F prior to case submission. No clinical signs were reported in layer pullets. At gross examinations, while nearly all flocks had colibacillosis, other lesions varied among farms. Peritonitis (farms A and B), hepatic lipidosis (farm A), and hepatic necrosis (farm B) were the primary gross lesions associated with layer cases. Various lesions such as laryngeal plugs and caecal haemorrhage were observed in pullet chickens received from farm C. Cases of broiler birds from farm D, and E were primarily associated with severe respiratory lesions in the forms of airsacculitis and caseous plugs in the bronchi (). Besides H9N2 LPAI, other pathogens such as Escherichia coli (all cases), fowl pox virus, and infectious bronchitis virus (cases from broiler farms only), were confirmed from laboratory examinations. Histopathological lesions observed by microscopic examinations are displayed in . No co-infection with HPAI was detected in any of the cases.
Figure 1. Individual egg production rate changes of farms affected by the new H9N2 field isolates. Time points of field sera and necropsy sample collection are indicated by arrows.
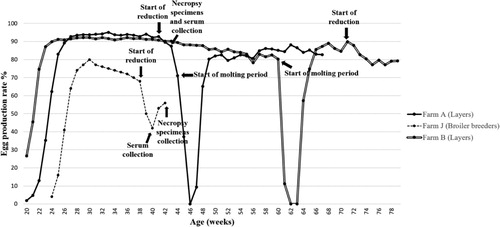
Figure 2. Lesions from post-mortem examinations of new H9N2 Y280-like LPAI cases in South Korea. (a) Fatty liver haemorrhagic syndrome (FLHS). (b) Necrotic and vacuolated hepatocytes. (c) Primary bronchi with caseous plugs (arrow). (d) Lymphocyte infiltration and cell necrosis in the mucosa with vacuoles containing cell debris in the trachea of an infected broiler. Loss of cilia was observed. The tracheal sample was positive for infectious bronchitis (IB) virus and H9N2 avian influenza (AI) virus by RT-PCR.
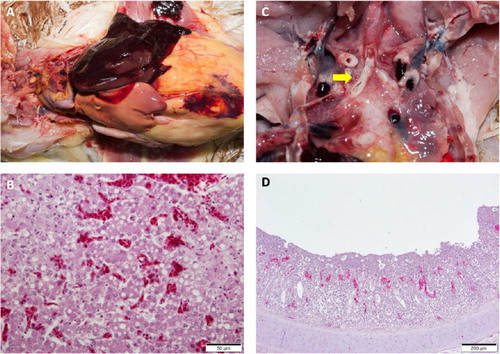
Molecular characterization and phylogeny of H9N2 field isolates
The phylogenetic analysis results based on the HA and NA gene nucleotide sequences from H9N2 isolates are presented in and (accession numbers: MW570860-MW570867, MW570852-MW570859). The eight H9N2 strains isolated from this study did not share close similarities with the previously prevalent H9N2 Korean/Y439-like lineage in South Korea. Instead, they were grouped with Y280-like H9N2 strains, forming a newly emerging group. The HA and NA nucleotide sequences of the field isolates from the newly created cluster showed the closest similarities with the Chinese Y280 A/chicken/Shandong/1844/2019 strain (HA homogeneity: 98.6–99.0%; NA homogeneity: 98.8–99.1%). To further understand the molecular properties relative to pathogenicity and interspecies transmission, the deduced amino acids of the field isolates were compared with Chinese Y280-like strains, A/chicken/Shandong/1844/2019, A/duck/Hong Kong/Y280/97, and the G1 lineage strain A/quail/Hong Kong/G1/97, (). All H9N2 field isolates harboured the same amino acid sequence motif of PSRSSR/G at the HA cleavage site (HACS), a typical LPAI motif identical with the A/chicken/Shandong/1844/2019 strain. An A316S substitution was also commonly detected in the cleavage site of all field isolates. Conserved PGSs (N-X-S/T) of the H9N2 field isolates are shown in . Six conserved PGSs were found at positions 11 (NST), 64 (NPS), 123 (NVS), 280 (NTT), 287 (NVS) and 295 (NCS). The A/chicken/Korea/1793/2020 strain possessed an additional PGS at position 127 (NGT). Interestingly, leucine at position 216, a human-like motif, was found at the left edge of the binding pocket of all field isolates (Matrosovich et al., Citation2001).
Figure 3. Phylogenetic analysis of the H9N2 Y280-like field isolates based on the nucleotide sequences of the haemagglutinin (HA) gene. The tree was constructed by the maximum likelihood (ML) method with Mega version 10. Black dots indicate H9N2 field isolates from this study. The A/chicken/Shandong/1844/2019 strain is indicated by a white dot. The representative strains for the Y280-like lineage, the Y439/Korean lineage and the G1 lineage are indicated by a black triangle, diamond and square, respectively. The strains from the Y439/Korean lineage and Y280-like lineage are shown on the right. Sequence data of the new H9N2 field isolates were submitted to GenBank under the accession numbers MW570860-MW570867.
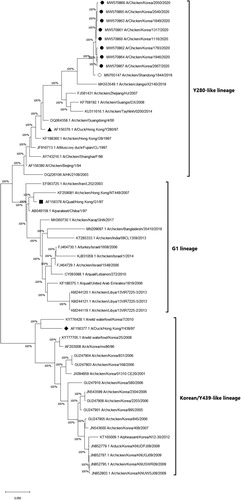
Figure 4. Phylogenetic analysis of the H9N2 Y280-like field isolates based on the nucleotide sequences of the neuraminidase (NA) gene. The tree was constructed by the maximum likelihood (ML) method with Mega version 10. Black dots indicate H9N2 field isolates from this study. The A/chicken/Shandong/1844/2019 strain is indicated by a white dot. The representative strains for the Y280-like lineage, Y439/Korean lineage and G1 lineage are indicated by a black triangle, diamond and a reversed white triangle, respectively. The NA gene of the A/chicken/Korea/580/2006 strain (black square) is known to have the highest sequence identity with the Korean live bird market H3N2-like lineage, not with H9N2 strains (Lee et al., Citation2012). Sequence data of the new H9N2 field isolates were submitted to GenBank under the accession numbers MW570852-MW570859.
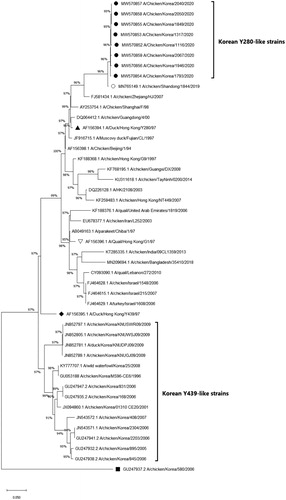
Table 2. Cleavage site and receptor-binding pocket of H9N2 field isolates and reference strains.
Table 3. Potential N-glycosylation sites (PGS) of the H9N2 field isolates and reference strains.
Serology of H9N2 field sera
Results of the HI assays conducted to determine the antigenic relatedness between the H9N2 field serum and the following reference strains, A/Chicken/Korea/1116/2020 (Y280 antigen) and A/chicken/Korea/01310/2001 (Y439 antigen), along with the number of sera tested, are presented in . A HI test using constant antigen (4 HA units of Y280 and Y439 virus antigen) and varying serum (field sera from affected farms) was employed to evaluate antibody titres. The average difference in HI titres between the two antigens varied from 0.7 log2 to 4.8 log2, showing some cross-reactivity. The average HI titres were generally higher when testing the field sera with the Y280 antigen compared to the Y439 antigen (mean titre: 7.8 log2 vs. 5.7 log2).
Table 4. Characteristics of field sera collected from infected farms.
Discussion
First documented in the 1990s, the poultry adapted Y280-like lineage of H9N2 has spread widely throughout the Eurasian continent, becoming endemic in multiple regions, especially in countries geographically close to South Korea (Pusch & Suarez, Citation2018; Peacock et al., Citation2019). Since Y280-like strains were first isolated by active governmental surveillance from Korean LBMs in June 2020, field case studies revealed that, starting from the first case in the same month, they have already become widespread in Korean chicken farms in various provinces, affecting multiple types of susceptible flocks. From June through December, Y280-like strains were isolated from four main provinces, Gyeonggi (northwestern), Chungbuk (central), Gyeongbuk (southeastern), and Chungnam (southwestern), indicating even distribution of virus spread. Such spatial–temporal patterns of epizootics indicated the recent introduction of the new Y280-like H9N2 strains. Most birds from affected farms showed overt signs of AIV infection, such as severe respiratory signs, drops in egg production, and mortality. Affected layer and breeder farms experienced egg production losses ranging from −10% to −27%, which persisted for around two to four weeks (). Interestingly, flocks from breeder farm J that experienced the most significant decrease in egg production turned out to be negative for H9N2 LPAI in RT–PCR assays but showed markedly high HI titres for the Y280-like virus, with most of the serum samples ranging from 10 log2 to 12 log2 (). A possible explanation behind this phenomenon would be that the virus may have been cleared from the flocks at the point when organic specimens were collected during post-mortem examinations, but the birds have already seroconverted against the field Y280-like strains. The sampling timings further support this assumption, as the field sera were collected at the peak of reduced egg production, while necropsy samples for viral RNA detection were submitted at the timepoint after egg production was clearly recovering. Gross lesions from post-mortem examinations varied among farms, although significant lesions in the respiratory tract, such as caseous plugs in the bronchi and airsacculitis, were mainly observed in infected broilers. However, clinical and lesion characteristics were not distinctive from previous H9N2 LPAI field cases in South Korea. Phylogenetic analysis of the HA and NA genes revealed that all the H9N2 strains isolated from this study were grouped into the Y280-like lineage and shared the highest similarities with the A/chicken/Shandong/1844/2019 strain, a recently isolated Y280 H9N2 strain from Chinese chicken farms, suggesting the emergence of a new cluster. The H9N2 strains from the new cluster did not group with any other previously isolated Korean strains, indicating that they are unlikely to be reassortant viruses between previously circulating Korean H9N2 viruses. On the contrary, the new isolates shared the highest nucleotide identities with Y280-like strains currently circulating in China. Moreover, the new Korean H9N2 field isolates may have directly originated from the A/chicken/Shandong/1844/2019 strain, suggesting the incursion of Y280-like strains from China. The close genetic relationship between the strains isolated from our study and the A/chicken/Shandong/1844/2019 strain became more evident when analysing the HA cleavage site, receptor-binding site (RBS), and PGSs. All field isolates and A/chicken/Shandong/1844/2019 possessed the same PSRSSR/G motif positioned between the HA1 and HA2 cleavage site, which was different from other reference H9N2 strains (). The PSRSSR/G motif in the cleavage site was previously determined in Y280-like viruses currently circulating in China (Song et al., Citation2019). All isolates from this study remarkably harboured the A316S substitution at the cleavage site, which was known to strengthen the virulence of H9N2 in chickens (Suzuki, Citation2005). The possibility of increased viral virulence due to this residue may indicate why high mortality was found in some farms infected with these field strains. Moreover, these strains also shared the same six PGS motifs with the Shandong strain, with strain A/chicken/Korea/1793/2020 (H9N2) possessing an additional seventh PGS (). Although PSGs in the HA region are known to have a specific impact on viral virulence of AIVs, like contributing to the evasion of the host immune system, no particular PSG motif has been identified in H9N2 viruses that is directly correlated to adaptation in gallinaceous species (Perez et al., Citation2003; Arnold et al., Citation2007; Tate et al., Citation2014). Another notable finding relating to the virus host range was the 216-Leu (L216) motif found at the left edge of the binding pocket of the HA protein () of all field isolates. Previous reports showed that this human-like motif at the RBS could potentially affect host specificity because it has a higher binding affinity for the α2,6-linked sialic acid receptors, similar to human influenza viruses (Rogers et al. Citation1983; Weis et al. Citation1988, Matrosovich et al. Citation2001). These findings suggest that the isolates may have the potential ability to infect mammalian species, including humans, demonstrating a possible public health risk. Overall, the genetic analysis results from this study coincide with a recent study indicating that Y280-like strains circulating in Korean LBMs are likely direct descendants of the A/chicken/Shandong/1844/2019 strain, without further reassortments with any other influenza viruses (Youk et al., Citation2021). Virus-neutralizing antibodies to the new Y280-like H9N2 virus were detected in field serum samples collected from affected chicken farms. The field sera reacted to the Y280 antigen with high virus neutralizing titres, indicating that infected flocks had seroconverted to Y280-like field viruses. However, the same field sera collected from Y280 virus-infected flocks also strongly reacted to the Y439 antigen with similar titres. This was not surprising as most affected farms implemented vaccination programmes using the widely distributed inactivated Y439-like H9N2 vaccines (). It seemed likely that these vaccines were accountable for the existence of neutralizing antibodies for Y439-like viruses in the field sera collected from Y280-like virus-infected flocks and that these vaccine-induced antibodies had reacted to the Y439 antigen. Further evidence of vaccine-induced immunity confounding with serological test results for Y280-like viruses could be found in a case involving an affected farm with no vaccination programmes for Y439-like H9N2 viruses. When evaluating the field sera collected from farm C that did not vaccinate against Y439-like H9N2 AIV, the average HI titre when testing with the Y280 antigen was noticeably and significantly higher compared to the Y439 antigen (mean titre: 6.8 log2 vs. 1.9 log2). Based on these serological findings and post-mortem and clinical examinations, it seemed evident that, even for birds carrying neutralizing antibodies for Y439-like viruses, it did not help much in reducing transmission of Y280-like viruses. From a diagnostic point of view, as serological cross-reactions in field cases between the two lineages of H9N2 are likely to be common, it is strongly recommended that standard antigens for both Y439 and Y280-like lineages should be prepared in laboratory settings for proper detection of Y280-like field isolates.
H9N2 viruses from the diverse Y280 lineage have long circulated in China, since the 1990s, and repetitive introduction of these strains to neighbouring countries or regions has long been a concern (Pusch & Suarez, Citation2018; Peacock et al., Citation2019). However, all the strains that caused past H9N2 outbreaks in South Korea were of the indigenous Korean Y439-like lineage origin. Even the incidence of these strains fell sharply over time after introducing inactivated H9N2 vaccines, in conjunction with strengthened control measures to prevent HPAI (Choi et al., Citation2008; Mo et al., Citation2016). It was not until recently that surveillance programmes identified that the non-indigenous H9N2 strains from the Y280-like lineage circulated in Korean LBMs (Heo et al., Citation2021, Youk et al., Citation2021). These LBM Y280-like strains would likely have spilled over into Korean industrial poultry populations, causing field infections nationwide. From a geographical perspective, this does not seem surprising, as spill-over cases from this lineage from China were recently reported in the Primorsky region of Russia and in Japan during 2019 and 2018, respectively, both regions being close to South Korea (Shibata et al., Citation2018; Sharshov et al., Citation2019) However, in the case of Japan, the viruses were isolated from imported poultry meat.
The poultry-adapted H9N2 viruses from the Y280-like lineage have long been a concern in the poultry industry and arose as a public health concern for humans. Human-adapted influenza viruses are known to have a high affinity for the 2,6-linked sialic acid receptors that are common in the upper respiratory tract in humans, and some isolates that belong to the Y280-like lineage carry mutations that can lead to a higher binding affinity to these receptors (Peiris et al., Citation2001; Li et al., Citation2003). Multiple cases of human infections of Y280-like H9N2 AI have been reported in the past (Butt et al., Citation2005; Huang et al., Citation2015). Moreover, due to their frequent contact with domestic poultry, poultry personnel at farms and LBMs are considered to be at high risk of infection with AI, and seroprevalence to Y280-like viruses in poultry workers was previously reported (Huang et al., Citation2013; Ma et al., Citation2018). All these facts together suggest that stronger surveillance programmes are imperative to contain these viruses that can pose an increasing threat to poultry and public health. Conclusively, based on the findings from our report, it was evident that although the inactivated H9N2 vaccines have worked effectively as intended against the predominant strains from the Y439-like/Korean lineage for years since their introduction in 2007, they did not exhibit efficient cross-protectivity against the Y280-like field strains. Furthermore, as it seems unlikely that the current H9N2 vaccines could protect against future Y280-like H9N2 AI outbreaks, developing specific vaccines against this lineage should be considered.
Disclosure statement
No potential conflict of interest was reported by the authors.
Additional information
Funding
References
- Alexander, D.J. (2007). An overview of the epidemiology of avian influenza. Vaccine, 25, 5637–5644.
- Agüero, M., Miguel, E.S., Sánchez, A., Gómez-Tejedor, C. & Jiménez-Clavero, M.A. (2007). A fully automated procedure for the high-throughput detection of avian influenza virus by real-time reverse transcription–polymerase chain reaction. Avian Diseases, 51, 235–241.
- APQA. (2020). Avian influenza surveillance in the Republic of Korea. In 2020 GFTADs Regional Expert Group Meeting for the Diseases of Poultry in Asia and the Pacific: Animal and Plant Quarantine Agency of Republic of Korea. 8–10.
- Arnold, J.N., Wormald, M.R., Sim, R.B., Rudd, P.M. & Dwek, R.A. (2007). The impact of glycosylation on the biological function and structure of human immunoglobulins. Annual Review of Immunology, 25, 21–50.
- Blaurock, C., Scheibner, D., Landmann, M., Vallbracht, M., Ulrich, R., Böttcher-Friebertshäuser, E., Mettenleiter, T.C. & Abdelwhab, E.M. (2020). Non-basic amino acids in the hemagglutinin proteolytic cleavage site of a European H9N2 avian influenza virus modulate virulence in turkeys. Scientific Reports, 10, 1–14.
- Butt, K.M., Smith, G.J., Chen, H., Zhang, L.J., Leung, Y.C., Xu, K.M., Lim, W., Webster, R.G., Yuen, K.Y., Peiris, J.M. & Guan, Y. (2005). Human infection with an avian H9N2 influenza A virus in Hong Kong in 2003. Journal of Clinical Microbiology, 43, 5760–5767.
- Cho, H.K., Kang, Y.M., Kim, H.M., Lee, C.H., Kim, D.Y., Choi, S.H., Lee, M.H. & Kang, H.M. (2020). Sales and immunogenicity of commercial vaccines to H9N2 low pathogenic avian influenza virus in Korea from 2007 to 2017. Vaccine, 38, 3191–3195.
- Choi, J.G., Lee, Y.J., Kim, Y.J., Lee, E.K., Jeong, O.M., Sung, H.W., Kim, J.H. & Kwon, J.H. (2008). An inactivated vaccine to control the current H9N2 low pathogenic avian influenza in Korea. Journal of Veterinary Science, 9, 67–74.
- Choi, Y.K., Ozaki, H., Webby, R.J., Webster, R.G., Peiris, J.S., Poon, L., Butt, C., Leung, Y.H.C. & Guan, Y. (2004). Continuing evolution of H9N2 influenza viruses in Southeastern China. Journal of Virology, 78, 8609–8614.
- Cross, G. (2002). Hemagglutination inhibition assays. Seminars in Avian and Exotic Pet Medicine, 11, 15–18.
- Dufour-Zavala, L. (2008). A laboratory manual for the isolation, identification, and characterization of avian pathogens: American Association of Avian Pathologists.
- Fouchier, R.A., Bestebroer, T.M., Herfst, S., Van Der Kemp, L., Rimmelzwaan, G.F. & Osterhaus, A.D. (2000). Detection of influenza A viruses from different species by PCR amplification of conserved sequences in the matrix gene. Journal of Clinical Microbiology, 38, 4096–4101.
- Guan, Y., Shortridge, K.F., Krauss, S., Chin, P.S., Dyrting, K.C., Ellis, T.M., Webster, R.G. & Peiris, M. (2000). H9N2 influenza viruses possessing H5N1-like internal genomes continue to circulate in poultry in southeastern China. Journal of Virology, 74, 9372–9380.
- Guan, Y., Shortridge, K.F., Krauss, S. & Webster, R.G. (1999). Molecular characterization of H9N2 influenza viruses: were they the donors of the “internal” genes of H5N1 viruses in Hong Kong? Proceedings of the National Academy of Sciences, 96, 9363–9367.
- Guo, Y., Krauss, S., Senne, D.A., Mo, I.P., Lo, K.S., Xiong, X.P., Norwood, M., Shortridge, K.F., Webster, R.G. & Guan, Y. (2000). Characterization of the pathogenicity of members of the newly established H9N2 influenza virus lineages in Asia. Virology, 267, 279–288.
- Heo, G.B., Kye, S.J., Sagong, M., Lee, E.K., Lee, K.N., Lee, Y.N., Choi, K.S., Lee, M.N. & Lee, Y.J. (2021). Genetic characterization of H9N2 avian influenza virus previously unrecognized in Korea. Journal of Veterinary Science, 22.
- Hoffmann, E., Stech, J., Guan, Y., Webster, R.G. & Perez, D.R. (2001). Universal primer set for the full-length amplification of all influenza A viruses. Archives of Virology, 146, 2275–2289.
- Homme, P. & Easterday, B. (1970). Avian influenza virus infections. I. Characteristics of influenza A/Turkey/Wisconsin/1966 virus. Avian Diseases, Feb 1, 66–74.
- Huang, R., Wang, A.R., Liu, Z.H., Liang, W., Li, X.X., Tang, Y.J., Miao, Z.M. & Chai, T.J. (2013). Seroprevalence of avian influenza H9N2 among poultry workers in Shandong Province, China. European Journal of Clinical Microbiology & Infectious Diseases, 32, 1347–1351.
- Huang, Y., Li, X., Zhang, H., Chen, B., Jiang, Y., Yang, L., Zhu, W., Hu, S., Zhou, S., Tang, Y. & Xiang, X. (2015). Human infection with an avian influenza A (H9N2) virus in the middle region of China. Journal of Medical Virology, 87, 1641–1648.
- Kimble, B., Nieto, G.R. & Perez, D.R. (2010). Characterization of influenza virus sialic acid receptors in minor poultry species. Virology Journal, 7, 365.
- Lee, C.H., Byun, S.H., Lee, Y.J. & Mo, I.P. (2012). Genetic evolution of the H9N2 avian influenza virus in Korean poultry farms. Virus Genes, 45, 38–47.
- Lee, C.W., Song, C.S., Lee, Y.J., Mo, I.P., Garcia, M., Suarez, D.L. & Kim, S.J. (2000). Sequence analysis of the hemagglutinin gene of H9N2 Korean avian influenza viruses and assessment of the pathogenic potential of isolate MS96. Avian Diseases, 44, 527–535.
- Lee, D.H., Fusaro, A., Song, C.S., Suarez, D.L. & Swayne, D.E. (2016). Poultry vaccination directed evolution of H9N2 low pathogenicity avian influenza viruses in Korea. Virology, 488, 225–231.
- Li, K.S., Xu, K.M., Peiris, J.S.M., Poon, L.L.M., Yu, K.Z., Yuen, K.Y., Shortridge, K.F., Webster, R.G. & Guan, Y. (2003). Characterization of H9 subtype influenza viruses from the ducks of southern China: a candidate for the next influenza pandemic in humans? Journal of Virology, 77, 6988–6994.
- Ma, M.J., Zhao, T., Chen, S.H., Xia, X., Yang, X.X., Wang, G.L., Fang, L.Q., Ma, G.Y., Wu, M.N., Qian, Y.H. & Dean, N.E. (2018). Avian influenza A virus infection among workers at live poultry markets, China, 2013–2016. Emerging Infectious Diseases, 24, 1246.
- Mardani, K., Browning, G., Ignjatovic, J. & Noormohammadi, A. (2006). Rapid differentiation of current infectious bronchitis virus vaccine strains and field isolates in Australia. Australian Veterinary Journal, 84, 59–62.
- Matrosovich, M.N., Krauss, S. & Webster, R.G. (2001). H9N2 influenza A viruses from poultry in Asia have human virus-like receptor specificity. Virology, 281, 156–162.
- Mo, I.P., Bae, Y.J., Lee, S.B., Mo, J.S., Oh, K.H., Shin, J.H., Kang, H.M. & Lee, Y.J. (2016). Review of avian influenza outbreaks in South Korea from 1996 to 2014. Avian Diseases, 60, 172–177.
- Mo, I.P., Song, C.S., Kim, K.S. & Rhee, J.C. (2003). An occurrence of non-highly pathogenic avian influenza in Korea. Avian Diseases, 47, 379–383.
- OIE. (2019). Chapter 3.3.4 Avian influenza (infection with avian influenza viruses). In Manual for Diagnostic Tests and Vaccines for Terrestrial Animals (pp. 830–831). Paris: OIE.
- Peacock, T.P., James, J., Sealy, J.E. & Iqbal, M. (2019). A global perspective on H9N2 avian influenza virus. Viruses, 11, 620.
- Peiris, J.S., Guan, Y., Markwell, D., Ghose, P., Webster, R.G. & Shortridge, K.F. (2001). Cocirculation of avian H9N2 and contemporary “human” H3N2 influenza A viruses in pigs in southeastern China: potential for genetic reassortment? Journal of Virology, 75, 9679–9686.
- Perez, D.R., Lim, W., Seiler, J.P., Yi, G., Peiris, M., Shortridge, K.F. & Webster R, G. (2003). Role of quail in the interspecies transmission of H9 influenza A viruses: molecular changes on HA that correspond to adaptation from ducks to chickens. Journal of Virology, 77, 3148–3156.
- Pusch, E.A. & Suarez, D.L. (2018). The multifaceted zoonotic risk of H9N2 avian influenza. Veterinary Sciences, 5, 82.
- Reid, S.M., Banks, J., Ceeraz, V., Seekings, A., Howard, W.A., Puranik, A., Collins, S., Manvell, R., Irvine, R.M. & Brown, I.H. (2016). The detection of a low pathogenicity avian influenza virus subtype H9 infection in a turkey breeder flock in the United Kingdom. Avian Diseases, 60, 126–131.
- Rogers G, N., Paulson J, C., Daniels R, S., Skehel J, J., Wilson I, A. & Wiley D, C. (1983). Single amino acid substitutions in influenza haemagglutinin change receptor binding specificity. Nature, 304, 76–78.
- Sharshov, K., Kurskaya, O., Sobolev, I., Leonov, S., Kabilov, M., Tatyana, A., Alekseev, A., Derko, A., Saito, T., Uchida, Y., Mine, J. & Shestopalov, A. (2019). First detection of a G1-like H9N2 virus in Russia, 2018. Korean Journal of Veterinary Research, 59, 37–42.
- Shibata, A., Hiono, T., Fukuhara, H., Sumiyoshi, R., Ohkawara, A., Matsuno, K., Okamatsu, M., Osaka, H. & Sakoda, Y. (2018). Isolation and characterization of avian influenza viruses from raw poultry products illegally imported to Japan by international flight passengers. Transboundary and Emerging Diseases, 65, 465–475.
- Sidoti, F., Rizzo, F., Costa, C., Astegiano, S., Curtoni, A., Mandola, M.L., Cavallo, R. & Bergallo, M. (2010). Development of real time RT-PCR assays for detection of type A influenza virus and for subtyping of avian H5 and H7 hemagglutinin subtypes. Molecular Biotechnology, 44, 41.
- Śmietanka, K., Minta, Z., Świętoń, E., Olszewska, M., Jóźwiak, M., Domańska-Blicharz, K., Wyrostek, K., Tomczyk, G. & Pikuła, A. (2014). Avian influenza H9N2 subtype in Poland characterization of the isolates and evidence of concomitant infections. Avian Pathology, 43, 427–436.
- Song, Y., Zhang, Y., Chen, L., Zhang, B., Zhang, M., Wang, J. & Jiang, T. (2019). Genetic characteristics and pathogenicity analysis in chickens and mice of three H9N2 avian influenza viruses. Viruses, 11, 1127.
- Spackman, E., & Killian, M. L. (2020). Avian influenza virus isolation, propagation, and titration in embryonated chicken eggs. In E. Spackman, & J. M. Walker (Eds.), Animal Influenza Virus (pp. 149–164). New York: Humana.
- Suzuki, Y. (2005). Sialobiology of influenza: molecular mechanism of host range variation of influenza viruses. Biological and Pharmaceutical Bulletin, 28, 399–408.
- Swayne, D., Suarez, D. & Sims, L. (2020). Influenza. In D. Swayne, M. Boulianne, C. Logue, L. McDougald, V. Nair, D. Suarez, S.D. Wit, T. Grimes, D. Johnson, M. Kromm, T. Prajitno, I. Rubinoff & G. Zavala (Eds.), Diseases of Poultry 14 ed. (pp. 210–256). Hoboken, NJ: John Wiley.
- Tate, M.D., Job, E.R., Deng, Y.M., Gunalan, V., Maurer-Stroh, S. & Reading, P.C. (2014). Playing hide and seek: how glycosylation of the influenza virus hemagglutinin can modulate the immune response to infection. Viruses, 6, 1294–1316.
- Weis, W., Brown J, H., Cusack, S., Paulson J, C., Skehel J, J. & Wiley D, C. (1988). Structure of the influenza virus haemagglutinin complexed with its receptor, sialic acid. Nature, 333, 426–431.
- Youk, S.S., Lee, D.H., Jeong, J.H., Pantin-Jackwood, M.J., Song, C.S. & Swayne, D.E. (2020). Live bird markets as evolutionary epicentres of H9N2 low pathogenicity avian influenza viruses in Korea. Emerging Microbes & Infections, 9, 616–627.
- Youk, S., Cho, A.Y., Lee, D.H., Jeong, S., Kim, Y., Lee, S., Kim, T.H., Pantin-Jackwood, M.J. & Song, C.S. (2021). Detection of newly introduced Y280-lineage H9N2 avian influenza viruses in live bird markets in Korea. Transboundary and Emerging Diseases, 1–5.