ABSTRACT
To compare the therapeutic effects of iron chelators used alone or in combination with phlebotomy on iron storage disease (ISD), 66 healthy common mynahs (Acridotheres tristis) were fed an iron-loading diet (3000 ppm) for 30 days. After confirmation of ISD, the birds were randomly divided into four treatment groups; DFO: deferoxamine (100 mg/kg SC q24 h), DFP: deferiprone (oral, 75 mg/kg), DFO + F: deferoxamine (100 mg/kg SC q24 h) with phlebotomy, and DFP + F: deferiprone (oral, 75 mg/kg) with phlebotomy. In phlebotomy-treated groups, blood sampling (1% BW) was performed weekly. At 1 and 2 months after treatments, seven birds from each group were euthanized and liver iron, copper, and zinc were analysed by ICP-OES assay. After 1 month, in all treatments, the liver amount of iron, copper, and zinc was reduced (P < 0.05) and there was no significant difference between groups. In the second month, the amount of liver iron, copper, and zinc decreased more in all groups, but this change was insignificant except in the DFP + F group (P < 0.05). These results suggest that all therapeutic protocols after 1 month effectively reduce the liver iron and there is no need to continue treatment. Otherwise, it may lead to iron deficiency, especially in birds treated with DFP + P. Since deferiprone, as an inexpensive oral chelator, effectively reduces liver iron levels without causing stress in the birds, it can be recommended as a more appropriate method for the treatment of mynahs with ISD. However, further clinical studies are needed to define the most effective treatment.
RESEARCH HIGHLIGHTS
Deferiprone is an optimized method for treating iron storage disease.
The essential metals homeostasis is impaired in iron storage disease.
Introduction
Iron storage disease (ISD) is a life-threatening disease that has been described in a variety of captive wild species of birds such as mynahs (Sturnidae), toucans (Rhamphastidae), birds-of-paradise (Paradisaeidae), quetzals (Pharomachrus species), and curassow (Cracidae) (Campbell, Citation2012). Genetic and excessive dietary iron intake are postulated causes of this disease (Crissey et al., Citation2000; Campbell, Citation2012). As an adaptation to insufficiency of their dietary iron, overexpression of ferroportin-1 (Ireg-1) and divalent metal transporter 1 (DMT1) occurs in the duodenum of these species (Mete et al., Citation2005; Olias et al., Citation2011). Due to the lack of awareness, captive birds are often fed high-iron diets that are not suited to their physiology. Although the pathogenesis of ISD is poorly understood, excess iron accumulates in various soft tissues, including the liver, heart, and spleen, and leads to cell injury mediated through the production of free radicals and the Fenton reaction (Adams & Barton, Citation2010). In addition to dietary management, different treatments such as phlebotomy and iron chelating agents are recommended to normalize body iron storage and to improve organ dysfunction (Olsen et al., Citation2006b).
Besides the efficacy of treatments, the treatment of choice for patients with ISD depends on other factors such as cost, stress, and side effects of treatment. Both deferoxamine injection and phlebotomy are stressful and lead to increased excitability in birds. Bird owners are not able to perform phlebotomy on their own and, usually, birds should be transported weekly to the veterinary clinic. Since deferoxamine is not orally absorbed, it requires slow sub-cutaneous administration (Adams & Barton, Citation2010). In addition to the stress of injections, deferoxamine is a very expensive iron chelator. In contrast, deferiprone is an orally active and economical iron chelator (Eybl et al., Citation2002). Some of these methods have been applied individually or in combination for the treatment of some avian species with ISD (Seibels et al., Citation2003; Drews et al., Citation2004; Whiteside et al., Citation2004; Olsen et al., Citation2006b; Sandmeier et al., Citation2012). So far, the therapeutic effects of these methods have not been investigated in common mynahs with ISD. This study aimed to (a) investigate the changes in levels of essential metals such as iron, copper, and zinc in common mynahs with experimentally induced ISD, (b) compare the therapeutic effects of oral and parenteral chelators as well as their concomitant administration with phlebotomy in mynahs with ISD, and (c) determine whether treatments with iron chelators and phlebotomy interfere with the homeostasis of essential metal ions.
Materials and methods
Birds
In this study, 66 clinically healthy common mynahs were captured from different regions in Iran and carried to an experimental indoor aviary in the Ferdowsi University of Mashhad. The birds were housed in groups of four in cages (30 cm × 40 cm × 80 cm) under similar ambient conditions (humidity 35–55%, 12-h light cycle, and temperature 19–23°C) and had free access to deionized drinking water and controlled iron diet (Dr. Birds, Mynahplex 60, Mashhad, Iran). According to the manufacturer’s instruction, the batches of this diet contained 9 ppm iron, 2.2 ppm copper, and 22 ppm zinc.
After allowing 2 weeks for acclimation and ensuring the health of the birds, five birds were euthanatized to determine the levels of liver iron, zinc, and copper before induction of ISD.
Experimental induction of iron storage disease
For induction of ISD, all remaining birds (n = 61) received an iron loading diet containing 3000 ppm of iron for 30 days. Iron was included in the high-iron diet as inorganic iron sulphate (ferrous sulphate heptahydrate, Behdam Roshd, Mashhad, Iran). Following administration of the high-iron diet, birds were monitored for clinical signs related to iron toxicity. At the end of this period, to ensure the induction of the ISD, five birds were euthanatized for evaluation of the liver iron concentration. Liver iron concentration equal to 1882–4233 ppm was considered as ISD (Olsen et al., Citation2006a).
Treatments of the birds
The remaining 56 birds were randomly divided into four groups (n = 14): DFP group treated with deferiprone (oral, 75 mg/kg, Avicenna pharmaceutical Inc, Tehran, Iran), DFO group received deferoxamine (100 mg/kg SC q24 h, Novartis, Basel, Switzerland), DFP + F group treated with deferiprone (oral, 75 mg/kg, Avicenna pharmaceutical Inc) and phlebotomy and DFO + F group treated with deferoxamine (100 mg/kg SC q24 h, Novartis) and phlebotomy. During the therapeutic period (2 months), all treatment groups were fed a low-iron diet containing 45 ppm of iron.
In groups treated with phlebotomy (DFO + F and DFP + F), a blood volume of approximately 1% of the birds’ body weight was collected from the wing vein weekly for 2 months. In these birds, haematocrit was also monitored manually in the obtained blood samples and phlebotomy ceased if the haematocrit reached less than the lower reference limit (35%) to avoid anaemia (Campbell, Citation2012).
A suspension of deferiprone (75 mg/kg BW) in propylene glycol (1 ml) was gavaged once daily into the crop of 12-h-fasted birds in the DFP and DFP + F groups (Whiteside et al., Citation2004). The appropriate dose of deferoxamine (100 mg/kg) was injected subcutaneously in the skin covering the pectoral muscle of birds in the DFO, DFO + F groups (Olsen et al., Citation2006b). The site of injection was changed intermittently to minimize discomfort.
At the end of the first and second months of treatments, seven birds from each of the treatment groups were euthanatized for evaluation of the levels of liver iron, zinc, and copper.
Measurement of the liver concentrations of iron, copper, and zinc
Birds were euthanatized by administering ketamine/xylazine (IM, 30 mg/kg/5 mg/kg), and when unresponsive to a toe pinch and single-feather extraction, they were exsanguinated. The right lobe of the liver was collected and rinsed of blood with normal saline then placed immediately on ice for transport to the laboratory.
Firstly, the liver samples (1 g) were digested with a 3:1 volumetric mix of nitric acid to hydrochloric acid and then digestions were incubated for 24 h at 105°C (Nolte, Citation2003). The liver concentrations of iron, copper, and zinc were measured by inductively coupled plasma – optical emission spectrometry (ICP-OES, Spectro ARCOS System, Model 76004555, Kleve, Germany). Detection limits were equal to 0.7 μg/g for iron, 0.4 μg/g for copper, and 0.2 μg/g for zinc. Element levels are expressed in μg/g of fresh weight.
Statistical analysis
Statistical analysis was performed by SPSS software (version 21). The distribution of the obtained data was assessed by Kolmogorov–Smirnov test. The distribution of data was not normal except for body weight. Liver iron, copper, and zinc concentrations in the pre- and post-iron loading times were compared with the Wilcoxon test. Comparison of these parameters between treatments was performed by the Kruskal Wallis test. Comparison of parameters between the first and second months of treatments was performed with the Wilcoxon signed-rank test. Results for body weight were analysed using repeated measure ANOVA followed by the Bonferroni post hoc test. P < 0.05 was considered as the significance level.
Results
Comparison of the liver concentrations of iron, copper, and zinc before and after induction of ISD
After induction of ISD, the liver concentrations of iron and zinc significantly (P < 0.05) increased while the copper concentration significantly (P < 0.05) decreased when compared to pre-iron load time ().
Figure 1. Comparison of the liver iron following different treatments. DFP: deferiprone; DFP + F: deferiprone and phlebotomy; DFO: deferoxamine; DFO + F: deferoxamine and phlebotomy. † Significant difference between treatments and post-iron loading time (P < 0.05), ‡ Significant difference between treatments and both pre- and post-iron loading times (P < 0.05), * Significant difference between the first and second month of treatments (P < 0.05). No significant differences present among treatments.
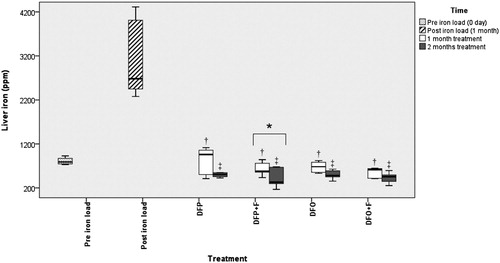
Figure 2. Comparison of the liver zinc following different treatments. DFP: deferiprone; DFP + F: deferiprone and phlebotomy; DFO: deferoxamine; DFO + F: deferoxamine and phlebotomy. † Significant difference between treatments and post-iron loading time (P < 0.05), * Significant difference between the first and second month of treatments (P< 0.05). No significant differences present among treatments.
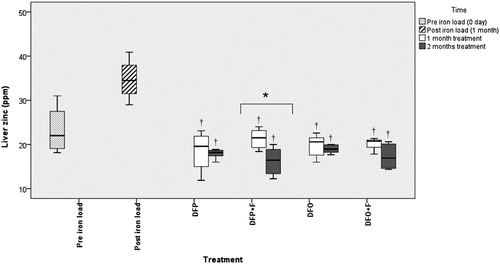
Figure 3. Comparison of the liver copper following different treatments. DFP: deferiprone; DFP + F: deferiprone and phlebotomy; DFO: deferoxamine; DFO + F: deferoxamine and phlebotomy. ‡ Significant difference between treatments and pre-iron loading time (P < 0.05), * Significant difference between the first and second month of treatments (P < 0.05). No significant differences present among treatments.
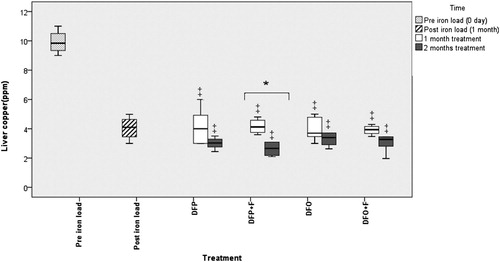
Comparison of the liver concentrations of iron, copper, and zinc during the first month of treatment
The iron and zinc levels in all treatments were significantly lower than in the post-iron load group (P < 0.05). The liver copper level in different treatments was significantly lower (P < 0.05) than in the pre-iron load group. However, there were no significant differences in the iron, zinc, and copper concentrations between treatments ().
Comparison of the liver concentrations of iron, copper, and zinc during the second month of treatment
During the second month of treatment, no significant difference was observed in the liver iron, copper, and zinc concentrations between groups. The decreasing trend of liver iron continued, and liver iron concentration in all treatments was significantly lower when compared to both pre- and post-iron load groups (P < 0.05). In all treatments, the liver concentration of copper was significantly lower compared to the pre-iron load group (P < 0.05) while the liver concentration of zinc was significantly lower compared to post-iron load group (P < 0.05) ().
There was no significant difference in the liver iron, copper, and zinc between the first and second months of treatments except in the DFP + F group.
Alteration of the birds’ body weights following different treatments
In the last days of the iron-loading period, most birds exhibited clinical signs such as lethargy, anorexia, and weight loss and two birds died. At necropsy, all birds showed ascites, hepatomegaly, and cardiomegaly.
Table 1. Comparison of the body weights (mean ± SE) following different treatments.
The treatment had no significant effect on the body weight, but the time of sampling, and also treatment and the sampling time interactions (treatment × time), had a significant effect (P < 0.05) on the body weight ( In post-iron loading time, body weight decreased significantly (P < 0.05) and after that it showed an increasing trend in all treatments. After 1 month of treatment, body weight significantly increased only in the DFP treatment when compared to post-iron load time (P < 0.05). After 2 months of treatment, body weight in all treatments except in DFO + F significantly increased (P < 0.05) and reached the range of pre-iron load time.
Discussion
Although mynahs are highly susceptible to iron storage disease, to the authors’ knowledge, little information exists about the different treatment protocols for this species. The first aim of this study was the induction of the ISD in common mynah and the investigation of change in liver iron storage in experimentally induced ISD. After 1 month of intake of a high-iron diet (3000 ppm), the birds exhibited clinical signs and necropsy findings that were previously described in ISD (Cork, Citation2000; Sheppard & Dierenfeld, Citation2002). The liver iron concentration in post-iron load time ranged from 2363–4163 ppm that was considerably higher than pre-iron load time (3.22–4.81 ppm). Similar liver iron levels have been reported in starlings with experimentally induced ISD (Crissey et al., Citation2000; Olsen et al., Citation2006a).
Diet modification is the first therapeutic strategy suggested for the treatment of ISD (Olsen et al., Citation2006a). The effectiveness of diet modification alone was not evaluated in our study; if this method is used alone a rapid decline in the liver iron level would not have occurred. After a 16-week treatment, a small reduction of the liver iron was observed for starlings with experimentally induced ISD that were fed only the low-iron diet (Olsen et al., Citation2006b). Since ISD is a life-threatening disease, a rapid reduction of iron concentration is essential in the treatment of clinical cases. Therefore, other treatments, such as phlebotomy, chelator agents as well as adding phytates and tannins to the diet, are considered in conjunction with diet modification to achieve further and faster reductions in tissue iron.
The second purpose of this study was to compare the effectiveness of oral and parenteral chelation therapies in the treatment of mynahs with ISD. In this study, deferoxamine and deferiprone led to the effective reduction of liver iron in mynahs with ISD. No significant difference was found between groups treated with these chelators. There are few studies about the therapeutic effects of iron chelators in birds with ISD (Olsen et al., Citation2006b; Whiteside et al., Citation2004; Sandmeier et al., Citation2012). Deferiprone has been found to effectively reduce the liver iron content in White Leghorn chickens, domestic pigeons, and hornbills with ISD (Whiteside et al., Citation2004; Sandmeier et al., Citation2012). So far, the therapeutic effects of deferoxamine have only been investigated in European starlings with ISD in which after 16 weeks of treatment with deferoxamine, non-haem liver iron concentrations decreased to safe levels (Olsen et al., Citation2006b). Our results indicated that deferoxamine reduced liver iron levels in mynahs faster than in starlings; the reason for this difference is unclear and needs further research.
The comparison of the effects of chelators on the amount of tissue iron in iron-loaded mice revealed that treatment with deferoxamine and deferiprone significantly and equally decreased the liver, heart, and kidney iron levels (Eybl et al., Citation2002). Phlebotomy is known as a standard treatment for haemochromatosis, and recently its effectiveness has been reported in starlings with experimental ISD (Olsen et al., Citation2006b). Hence, a combination of chelation therapy and phlebotomy can be considered as a novel option for the rapid removal of excess liver iron in birds with ISD.
In this study, although a greater rate of iron loss was found for the birds treated with a combination of chelation therapy and phlebotomy when compared to those treated only with chelators, this difference was not significant. To date, no study has been performed to compare the effects of oral and parenteral chelators as well as their concomitant administration with phlebotomy in birds with ISD. Olsen et al. (Citation2006b) showed that deferoxamine is more efficient than phlebotomy in the mobilization of iron in starlings with ISD (Olsen et al., Citation2006b).
According to our results, induction of ISD significantly increased the liver concentration of zinc while the copper concentration decreased significantly. Since we don’t have information about the amount of zinc and copper in the natural diet of these birds, perhaps any effect in this study was due to dietary change.
However, by our results, a lower hepatic copper concentration has been reported in starlings fed the high-iron diet for 16 weeks when compared with those fed the controlled iron diet (Crissey et al., Citation2000). This may be attributed to the competition of divalent cations for tissue absorption sites. In iron overload, occupying more binding sites probably reduces copper binding in the liver (Harvey, Citation2008).
There are conflicting reports regarding the influence of high iron intake on the hepatic level of copper in rats. No significant alteration was observed in the copper content of the liver and spleen in rats that received iron either per os or parenterally (Vayenas et al., Citation1998). Although a low hepatic level of copper has been reported in some studies (Klevay, Citation2001; Ha et al., Citation2017), Kumagai et al. (Citation1993) reported that the copper content of the liver increased significantly in pregnant rats that received a high iron diet (Kumagai et al., Citation1993). Since the absorption of copper is more efficient in pregnant rats than non-pregnant rats, they suggested that this inconsistency of findings may be due to the physiological difference of rats (Kumagai et al., Citation1993).
There is no information about the influence of high iron intake on the zinc content in the liver of birds. However, an insignificant increase of zinc has been reported in the plasma of leghorn chickens and domestic pigeons after 30-day treatment with Fe dextran (25 mg/kg, i.v.) (Whiteside et al., Citation2004). In agreement with our findings, a high level of zinc has been reported in the liver and spleen of rats receiving high iron diets (Vayenas et al., Citation1998; Yasutake & Hirayama, Citation2004). Yasutake and Hirayama (Citation2004) demonstrated that, following a transient decrease at the early phase of iron overload, the levels of liver zinc and metallothionein increased significantly (Yasutake & Hirayama, Citation2004). Zinc is stored mainly in the liver bound to metallothionein and/or ferritin which are, respectively, a cytosolic chelator of heavy metals and an iron-storage protein (Himoto & Masaki, Citation2018; Yasutake & Hirayama, Citation2004). Therefore, the zinc retention in the iron-overloaded liver may be attributed to an abundance of metallothionein and ferritin (Vayenas et al., Citation1998).
Finally, this study was conducted to determine whether treatments with iron chelators and phlebotomy interfere with the homeostasis of essential metal ions. We found that, despite the effective reduction of iron, administration of iron chelators, either alone or in combination with phlebotomy, was not without side effects. All therapeutic protocols resulted in a significant reduction of copper content of liver in mynahs with ISD when compared to healthy birds. In all treatments, a slight reduction was also observed in zinc levels when compared to healthy birds. A significant reduction of plasma zinc level was reported in White Leghorn chickens and domestic pigeons which were treated with deferiprone for 1 month (Whiteside et al., Citation2007).
To the authors' knowledge, so far, the effects of deferoxamine on the storage of zinc and copper in birds are not well documented. In agreement with our results, successful chelation therapy using deferiprone to remove copper ions in rats with copper intoxication has been reported. After chelation therapy, iron and zinc concentrations also significantly decreased (Amiri & Rahimipour, Citation2015).
Zinc deficiency is not uncommon in patients undergoing chelation therapy (Sultan et al., Citation2015; Erdoğan et al., Citation2013). It has been suggested that hyperzincuria contributes to zinc deficiency in patients (Erdoğan et al., Citation2013). Since chelators have a strong affinity for divalent cations such as iron, zinc, copper, and aluminium, treatment of ISD with these chelators can induce mineral deficiency. Although it is best to evaluate the level of minerals while birds are being treated with iron chelators, there are some limitations. Liver biopsy is an aggressive procedure and is not recommended, especially in small birds. Measurement of plasma/serum copper levels can be useful in suspected cases of copper deficiency, but it is often insensitive for accurate diagnosis of zinc deficiency. Neutrophil zinc has been reported to be more sensitive to zinc status than erythrocyte and plasma/serum zinc, but testing efficacy is limited, because conventional assays require a large amount of blood (Rucker et al., Citation2008). Therefore, further investigation is needed to find an appropriate approach for evaluating zinc status.
Conclusion
These results suggest that the use of all therapeutic protocols after 1 month effectively reduces the amount of iron in the liver and there is no need to continue treatment. Otherwise, continued treatment may lead to the deficiency of essential metals, especially in the birds treated with the combination of deferiprone and phlebotomy.
Because deferiprone, as an inexpensive oral chelator, effectively reduces liver iron levels in the birds, it can be recommended as a more appropriate method for the treatment of mynahs with ISD.
Although oral treatment is less stressful than weekly bleeding of birds and daily injections of deferoxamine, catching a bird on a daily basis to give oral medication is also stressful. The next logical step in this research is to determine if deferiprone could be given in drinking water or food, eliminating the need for bird handling.
Ethics approval
All animal experiments were performed in strict accordance with the guidelines approved by the Animal Ethics Committee of the Ferdowsi University of Mashhad, Iran (IR.UM.REC.1400.030).
Disclosure statement
No potential conflict of interest was reported by the authors.
Additional information
Funding
References
- Adams, P.C. & Barton, J.C. (2010). How I treat hemochromatosis. Blood, 116, 317–325.
- Amiri, A. & Rahimipour, M. (2015). Comparative effects of deferasirox and deferiprone in the treatment of copper intoxication in rats. Toxin Reviews, 34, 173–176.
- Campbell, T.W. (2012). Hematology of birds. In M.A. Thrall, G. Weiser, R. Allison & T. Campbell (Eds.), Veterinary Hematology and Clinical Chemistry (pp. 252–191). Ames, IA: Wiley.
- Cork, S.C. (2000). Iron storage disease in birds. Avian Pathology, 29, 7–12.
- Crissey, S.D., Ward, A.M., Block, S.E. & Maslanka, M.T. (2000). Hepatic iron accumulation over time in European starlings (Sturnus vulgaris) fed two levels of iron. Journal of Zoo and Wildlife Medicine, 31, 491–496.
- Drews, A.V., Redrobe, S.P. & Patterson-Kane, J.C. (2004). Successful reduction of hepatocellular hemosiderin content by dietary modification in toco toucans (Ramphastos toco) with iron-storage disease. Journal of Avian Medicine and Surgery, 18, 101–105.
- Erdoğan, E., Canatan, D., Örmeci, A.R., Vural, H. & Aylak, F. (2013). The effects of chelators on zinc levels in patients with thalassemia major. Journal of Trace Elements in Medicine and Biology, 27, 109–111.
- Eybl, V., Kotyzová, D., Kolek, M., Koutenský, J. & Nielsen, P. (2002). The influence of deferiprone (L1) and deferoxamine on iron and essential element tissue level and parameters of oxidative status in dietary iron-loaded mice. Toxicology Letters, 128, 169–175.
- Ha, J.H., Doguer, C. & Collins, J.F. (2017). Consumption of a high-iron diet disrupts homeostatic regulation of intestinal copper absorption in adolescent mice. American Journal of Physiology-Gastrointestinal and Liver Physiology, 313, G353–G360.
- Harvey, J.W. (2008). Iron metabolism and its disorders. In J.J. Kaneko, J.W. Harvey & M.L. Bruss (Eds.), Clinical Biochemistry of Domestic Animals 6th edn (p. 262). New York: Elsevier Academic.
- Himoto, T. & Masaki, T. (2018). Associations between zinc deficiency and metabolic abnormalities in patients with chronic liver disease. Nutrients, 10, 88.
- Klevay, L.M. (2001). Iron overload can induce mild copper deficiency. Journal of Trace Elements in Medicine and Biology, 14, 237–240.
- Kumagai, H., Kawashima, R. & Yano, H. (1993). Effects of excessive amounts of dietary iron on pregnant rats: iron, copper, zinc, manganese and selenium statuses in mothers and fetuses. Animal Science and Technology, 64, 780–789.
- Mete, A., Jalving, R., van Oost, B.A., van Dijk, J.E. & Marx, J.J. (2005). Intestinal over-expression of iron transporters induces iron overload in birds in captivity. Blood Cells, Molecules, and Diseases, 34, 151–156.
- Nolte, J. (2003). ICP Emission Spectrophotometry: A Practical Guide. Weinheim: Wiley-VCH. p. 336.
- Olias, P., Weiss, A.T.A., Gruber, A.D. & Klopfleisch, R. (2011). Iron storage disease in red deer (Cervus elaphus elaphus) is not associated with mutations in the HFE gene. Journal of Comparative Pathology, 145, 207–213.
- Olsen, G.P., Russell, K.E., Dierenfeld, E., Falcon, M.D. & Phalen, D.N. (2006a). Impact of supplements on iron absorption from diets containing high and low iron concentrations in the European starling (Sturnus vulgaris). Journal of Avian Medicine and Surgery, 20, 67–73.
- Olsen, G.P., Russell, K.E., Dierenfeld, E. & Phalen, D.N. (2006b). A comparison of four regimens for treatment of iron storage disease using the European starling (Sturnus vulgaris) as a model. Journal of Avian Medicine and Surgery, 20, 74–79.
- Rucker, R.B., Fascetti, A.J. & Keen, C.L. (2008). Trace minerals. In J.J. Kaneko, J.W. Harvey & M.L. Bruss (Eds.), Clinical Biochemistry of Domestic Animals 6th edn (pp. 663–694). New York: Elsevier Academic.
- Sandmeier, P., Clauss, M., Donati, O.F., Chiers, K., Kienzle, E. & Hatt, J.M. (2012). Use of deferiprone for the treatment of hepatic iron storage disease in three hornbills. Journal of the American Veterinary Medical Association, 240, 75–81.
- Seibels, B., Lamberski, N., Gregory, C.R., Slifka, K. & Hagerman, A.E. (2003). Effective use of tea to limit dietary iron available to starlings (Sturnus vulgaris). Journal of Zoo and Wildlife Medicine, 34, 314–316.
- Sheppard, C. & Dierenfeld, E. (2002). Iron storage disease in birds: speculation on etiology and implications for captive husbandry. Journal of Avian Medicine and Surgery, 16, 192–197.
- Sultan, S., Irfan, S.M., Kakar, J. & Zeeshan, R. (2015). Effect of iron chelator desferrioxamine on serum zinc levels in patients with beta thalassemia major. The Malaysian Journal of Pathology, 37, 35–38.
- Vayenas, D.V., Repanti, M., Vassilopoulos, A. & Papanastasiou, D.A. (1998). Influence of iron overload on manganese, zinc, and copper concentration in rat tissues in vivo: study of liver, spleen, and brain. International Journal of Clinical & Laboratory Research, 28, 183–186.
- Whiteside, D.P., Barker, I.K., Conlon, P.D., Tesoro, A., Thiessen, J.J., Mehren, K.G., Jacobs, R.M. & Spino, M. (2007). Pharmacokinetic disposition of the oral iron chelator deferiprone in the domestic pigeon (Columba livia). Journal of Avian Medicine and Surgery, 21, 121–129.
- Whiteside, D.P., Barker, I.K., Mehren, K.G., Jacobs, R.M. & Conlon, P.D. (2004). Clinical evaluation of the oral iron chelator deferiprone for the potential treatment of iron overload in bird species. Journal of Zoo and Wildlife Medicine, 35, 40–49.
- Yasutake, A. & Hirayama, K. (2004). Effects of iron overload on hepatic and renal metallothionein levels in rats. Journal of Health Science, 50, 372–378.