ABSTRACT
Fourteen genetically different chicken Escherichia coli strains were biotyped in hens to examine if any E. coli strain at high dose can induce the E. coli peritonitis syndrome (EPS). Moreover, biotyping was performed in embryos and the median lethal dose (LD50) of three strains was determined in hens. Nine strains were obtained from femur marrow and one strain from caecum of hens that had died from EPS. One strain originated from the inflamed pericardium of a broiler and three strains from the cloaca of specified pathogen-free (SPF) broiler breeders. Strains were inoculated intratracheally into separate groups of 32 productive SPF White Leghorn (WL) hens at a dose of 7.8–9.2 log10 colony forming units (CFU) per hen and into the allantoic cavity of separate groups of 20 SPF WL embryos incubated during 14 days in a dose of 4.2–4.6 log10 CFU per embryo. The embryo test was replicated. Bone marrow and pericardium strains induced EPS, the other strains did not. Based on mortality in hens, EPS-inducing strains could be classified as very virulent (59–100% mortality), moderately virulent (38% mortality) and low virulent (6% mortality). In productive SPF WL hens, the LD50 of three very virulent strains ranged from <2.7 to 5.3 log10 CFU. Virulent and avirulent strains killed 60–95% and 0–30% of embryos, respectively. The embryo lethality test, which showed good reproducibility, did not discriminate within virulent strains, but can nevertheless be considered as a useful alternative for biotyping E. coli in productive hens.
RESEARCH HIGHLIGHTS
Even at high doses, not all E. coli strains can induce EPS.
Substantial differences in virulence exist within very virulent E. coli strains.
The embryo lethality test is a useful alternative for biotyping E. coli in laying hens.
Broiler colibacillosis may represent a source of EPS strains for layers and vice versa.
Introduction
Colibacillosis is a disease which frequently affects commercial poultry worldwide. It is caused by avian pathogenic Escherichia coli (APEC) and is regarded as the major cause of mortality in laying hens and broilers. In the latter category of birds, the most relevant form of colibacillosis is fibrinous polyserositis, which generally occurs during the second half of the grow-out period and is mainly caused by opportunistic E. coli bacteria. Important triggers of fibrinous E. coli polyserositis are mycoplasma infections (Gross, Citation1961; Fabricant & Levine, Citation1962) and infections with both field and vaccine strains of respiratory viruses such as infectious bronchitis virus (Matthijs et al., Citation2003; Matthijs et al., Citation2005), Newcastle disease virus (Van Eck & Goren, Citation1991) and avian pneumovirus (Al-Ankari et al., Citation2001).
In laying hens the prevailing colibacillosis form is the E. coli peritonitis syndrome (EPS) (Landman & Van Eck, Citation2015), which is characterized by (per)acute mortality, soaring up to 10% or more in combination with severe septicaemia and fibrinous polyserositis lesions, while salpingitis is seldomly found. EPS has been described in the USA and many European countries (Dhillon & Jack, Citation1996; Zanella et al., Citation2000; Vandekerchove et al., Citation2004a; Landman & Cornelissen, Citation2006; Raviv et al., Citation2007; Landman et al., Citation2013; Landman et al., Citation2014), but likely occurs in all continents. The economic impact of EPS is very significant due to its high incidence and the high mortality it induces. In a fairly recent study, it was shown that EPS occurs in 35% and 7% of Dutch meat reproduction and layer farms, respectively. The economic loss for the Dutch poultry farming as a whole was estimated at €3.7 million per year, resulting from hen mortality and subsequent egg production losses, reduced slaughter weight and increased costs of destruction of dead hens. The costs of antibiotic treatments further add to the economic impact of EPS (Landman & Van Eck, Citation2015).
EPS occurs after the E. coli strains of concern enter the bloodstream inducing bacteraemia. The primary port of entry is likely the respiratory tract, whilst entrance via the vaginal route may also occur (Vandekerchove et al., Citation2004b; Landman et al., Citation2013; Landman & Van Eck, Citation2017). Although the E. coli strains that induce EPS are likely primary virulent, experimental studies demonstrating this unequivocally are lacking. EPS has been induced successfully by merely inoculating E. coli strains obtained from the bone marrow of hens that died from EPS, id est without further triggers (Vandekerchove et al., Citation2004b; Landman et al., Citation2013; Landman & Van Eck, Citation2017). However, it could be argued that the large doses used in the various studies could have induced colibacillosis with any E. coli strain. Therefore, an experiment with productive White Leghorn (WL) hens was conducted in which E. coli strains originating from hens that died from EPS and from a broiler suffering from fibrinous polyserositis, were compared to strains originating from the cloaca of clinical healthy hens. Moreover, the median lethal dose (LD50) of three EPS strains obtained from bone marrow was also determined. Biotyping in adult chickens is cumbersome and costly. Consequently, in addition, we studied whether specified pathogen-free (SPF) WL embryos can be used as an alternative.
Materials and methods
E. coli strains
Origin
Characteristics of E. coli strains used for biotyping are listed in , including place and year of isolation. Nine strains originated from femur marrow of hens that died from EPS (referred to as bone marrow strains) (seven from layers and two from broiler-breeders), one strain from the caecum of a layer that also died from EPS (referred to as caecum strain), one strain from the inflamed pericardium of a broiler with fibrinous polyserositis (referred to as pericardium strain) (Van Eck & Goren, Citation1991) and three strains from the cloaca of clinical healthy SPF broiler breeders (referred to as cloaca strains).
Table 1. Characteristics of E. coli strains, which were biotyped in productive SPF White Leghorn hens and SPF White Leghorn embryos.
Isolation
Transversally cut bone physis was briefly sterilized with a flame (Blue Flame™ piezo gas burner GB-2001, Almere, Netherlands) until onset of tissue discolouration due to heat. Thereafter, a bone marrow sample was collected with a sterile loop. A sheep-blood agar plate (K004P090; Biotrading, Mijdrecht, Netherlands) was then inoculated. Cloacal swabs and caecal content samples, the latter obtained after sterilizing the outer caecum wall with a hot scalpel blade, were inoculated directly onto the mentioned medium. Agar plates were subsequently incubated overnight at 37°C under 5–10% carbon dioxide and thereafter visually inspected for colony growth and colony uniformity (purity). Cultures were assumed to be pure based on colony uniformity and identification. The pericardium strain was isolated at the former Poultry Health Centre, Doorn, Netherlands (Van Eck & Goren, Citation1991).
Identification of one colony, of which a small fraction was collected by means of a tooth pick, was performed using Matrix Assisted Laser Desorption/Ionisation Time of Flight Massa Spectrometry (MALDI-TOF MS) (Microflex; Bruker Daltonik, Bremen, Germany). The same colony was then scraped off the agar plate using a sterile loop and subsequently inoculated onto a sheep-blood agar plate which was then incubated overnight as described before. Thereafter, E. coli colonies were scraped off the plate with a swab, which was rinsed in a Protect Micro-organims Preservation System (TS/80-WH; Biotrading) following the manufacturer’s instructions. E. coli was stored between −60 and −90°C until use.
Molecular typing
E. coli strains were analysed by whole genome sequencing (WGS). The extracted DNA was used for the preparation of total DNA libraries using the Nextera XT DNA library preparation kit (Illumina, San Diego, CA, USA). 300-cycle sequence reads (2 × 150-bp paired-end) were generated using the Illumina NovaSeq 6000 system. Initial quality assessment was based on data passing the Illumina chastity filtering. Subsequently, reads containing PhiX control signal were removed using an in-house filtering protocol (Baseclear, Leiden, Netherlands). In addition, reads containing (partial) adapters were clipped (up to a minimum read length of 50 bp). The second quality assessment was based on the remaining reads using the FASTQC quality control tool version 0.11.5. De-multiplexed and adapter clipped reads were analysed using the CLC Genomics Workbench version 20.0.4. (Qiagen Aarhus A/S, Aarhus, Denmark). De novo assembly was performed using the slow mapping mode with a minimum expected contig size of 300 nucleotides. Max paired read distance allowed was 500 nucleotides. The parameters for mapping back the reads were: mismatch cost = 2; insertion cost = 3; deletion cost = 3; length fraction = 0.5 and similarity fraction = 0.8. Following de novo assembly, whole genome alignment of resulting scaffolds was performed, using CLC genomics as well. Parameters for the whole genome alignment were: minimum initial seed length = 15 with mismatches in seeds allowed; minimum alignment block length = 100 and rearranged contigs. From the resulting whole genome alignment, a nucleotide similarity matrix was constructed and subsequently a radial unrooted phylogenetic tree with neighbour joining clustering was created to demonstrate genetic diversity.
Serotyping
To determine the O- and H-serotypes the sequence data for all E. coli strains were uploaded to the Centre for Genomic Epidemiology (CGE) Serotype Finder database (Joensen et al., Citation2015).
Inocula
Preparation of inocula was performed by rolling one frozen bead (TS/80-WH; Biotrading) containing the E. coli strain of concern on a sheep-blood agar plate. After incubation for 21 ± 4 h at 37°C, a part of a colony was then scraped off using a wire and suspended in 3 ml physiological saline solution, which was vortexed for 3 s. This procedure was repeated until a concentration of 0.5 McFarland (108 colony forming units (CFU)/ml) was obtained. A volume of 0.1 ml of this suspension was mixed with 90 ml of 0.1% glucose broth (1000 ml purified water, 5 g Lab Lemco (OxoidLP0029; Oxoid, Badhoevedorp, Netherlands), 10 g bacteriological peptone (Oxoid LP0-037), 5 g NaCl (VWR 1.06404.1000; Merck Eurolab B.V., Amsterdam, Netherlands) and 1 g glucose (VWR 1.08342.1000; Merck)), which was incubated for 17 h at 37°C.
Undiluted E. coli suspensions (109 CFU/ml) and serial 10-fold dilutions in peptone physiological saline (PPS recipe number 110, BioTrading) were used as inocula, which were stored on melting ice at all times except during use. E. coli concentrations were assessed by means of bacterial counts according to international standards (ISO6887, Citation1983; ISO7402, Citation1985) immediately after administration.
Biotyping in hens
Chickens, housing and husbandry
Productive SPF WL hens obtained from Royal GD, Deventer, Netherlands (GD) were housed in separate negative-pressure high-efficiency particulate air isolators coupled to an extender with a total volume of 2.03 m3 each (TS Group Holland B.V., Buren, Netherlands). Birds were kept in the isolators until the end of the experiment. Isolator temperature was maintained at 18–20°C; relative humidity was 60–80%. Hens were exposed to 14 h of light per day. Birds were fed a standard commercial layer diet ad libitum and had free access to drinking water.
Experimental design
The experimental design is outlined in . Fourteen E. coli strains () were each biotyped in two groups of 16 egg-producing SPF WL hens housed in separate isolators (a total of 28 groups of 16 hens each). Hens were inoculated intratracheally with 1 ml E. coli suspension at the age of 37 weeks. Undiluted and 10−3 diluted E. coli suspensions (106 CFU/ml) were aimed for the inoculation of hens; however, due to a dilution error, a dilution of 10−1 (108 CFU/ml) was used instead of 10−3. Consequently, for each E. coli strain, one group received a target dose of 8.0 log10 CFU per hen and the other group a target dose of 9.0 log10 CFU per hen. After inoculation, mortality was recorded at intervals of approximately 8 h during the first four days and thereafter twice a day (between 07:00–09:00 and 10:00–13:00 at the weekend, and between 08:00–11:00 and 15:00–17:00 on other days). In order to enable the calculation of (mean) death time, the time of inoculation was recorded as well.
Table 2. Biotyping of chicken E. coli strains in productive SPF White Leghorn hens.
The experiment was ended 9 days after E. coli inoculations.
Post-mortem examination and bacteriology
Gross post-mortem examination of all dead hens was performed. Macroscopic lesions and ovarian activity (in production, disturbed [flaccid, corrugated, and leaking yellow follicles] and inactive [no yellow follicles present]) were recorded. Bacteriological examination of the femur marrow of dead hens was performed as described under E. coli strains.
After the experiment, surviving hens were used for the production of E. coli antisera, and therefore were not available for post-mortem examination and bacteriological analysis.
LD50
The LD50 of three E. coli EPS strains isolated from bone marrow (, strains 1, 3, and 6) for productive SPF WL hens was assessed in two trials as outlined in . In Trial 1, each of the three E. coli strains was inoculated into groups of hens in doses aiming at 8.0, 7.0, 6.0, and 5.0 log10 CFU per hen. A placebo (PPS) inoculated group was included. In Trial 2, strains 1 and 3, which had not induced mortality ≤50% in Trial 1, were each inoculated into groups of hens with an E. coli dosis aiming at 4.0 and 3.0 log10 CFU per hen. In both trials, per E. coli strain and dose groups consisted of 10 hens each and were housed in separate isolators. Hens were inoculated at 29 and 32 weeks of age, in Trials 1 and 2, respectively. Inoculations were performed intratracheally with a volume of 1 ml per hen. Housing and husbandry were as presented in “Biotyping in hens”. After inoculation, mortality was recorded at intervals of approximately 6 h during the first 3 days and, thereafter, twice a day as presented in “Biotyping in hens”. Gross post-mortem examination and bacteriological examination of femur marrow were performed on dead hens on the day they died and on surviving hens at the end of the trials at 7 days after inoculation.
Table 3. Assessment of the median lethal dose (LD50) of E. coli EPS strains for productive SPF White Leghorn hens.
The LD50 was calculated with the formula of Reed and Muench (Reed & Muench, Citation1938).
Biotyping in embryos
Fourteen-day-incubated SPF WL embryos obtained from GD were used for biotyping the E. coli strains in an embryo lethality assay. Eggs were incubated at 37.9°C and 53% relative humidity (Smart Combi incubator, serial number 08scom820113, Pas Reform, Zeddam, Netherlands). For each E. coli strain, 20 embryos were used. Also, two control groups each consisting of 20 embryos were included: one group of 20 embryos was placebo-inoculated with PPS, and the other group was left uninoculated. Before inoculation, eggs were candled by holding the aperture of a candling lamp against the blunt pole of the egg, after which the edge of the air chamber was marked and an area of approximately 2 cm2 of eggshell around the intended inoculation site was disinfected with a 1% iodine solution in alcohol 70%. A small pin-sized hole was then made approximately 2–3 mm below the air chamber edge with a drill (Hugo Brennenstuhl GmbH & Co Kommanditgesellschaft, Electric Engraver Signograph 25 Set, article number 1500740, Tübingen, Germany). Embryos were each inoculated with 0.2 ml of a 10−4 diluted E. coli suspension (105 CFU/ml) aiming at a dose of 4.3 log10 CFU per embryo. Inoculations were made into the allantoic cavity with a needle of 25 G (0.5 mm) × 2/8 inch (6 mm). The needle was completely inserted parallel to the long axis of the egg (Manders et al., Citation2021). All embryonated eggs were candled daily for 4 days consecutively, and daily mortality was recorded. Swab samples of dead embryos up to a maximum of five per treatment group were subjected to bacteriological analysis as described under “E. coli strains”. In cases where no mortality was observed, bacteriological examination was performed on five live embryos.
The embryo lethality assay was repeated a week later.
Statistical analysis
Differences in mortality were analysed using Fisher’s exact test (Statistix®, Citation2010). Differences were considered significant if P ≤ 0.05.
Ethics
This study was approved by the Animal Welfare Body of Royal GD and the Central Authority for Scientific Procedures on Animals, according to the Dutch Animal Procedure Act (Wet op de dierproeven).
Results
Molecular typing
WGS showed that the 14 E. coli strains were genetically different (). For most strains, the pairwise sequence similarity was below 95%, except for strains 3, 10, and 11 that showed a sequence similarity of 96%. Based on the E. coli MLST scheme in the Enterobase database (Zhou et al., Citation2020) the 14 strains could be distinguished into 12 different sequence types (ST). Strains 1 and 2 belonged to ST95, strain 3 to ST23, strain 4 to ST117, strain 5 to ST141, strain 6 to ST429, strain 7 to ST93, strain 8 to ST351, strain 9 to ST5420, strains 10 and 11 to ST2230, strain 12 to ST349, strain 13 to ST1914, and strain 14 to ST1276.
Figure 1. Radial unrooted phylogenetic tree based on full genome sequences of 14 E. coli strains demonstrates these strains are genetically different. Data were clustered using the neighbour joining method and E. coli chromosome as well as plasmid sequences are included. Samples are numbered according to . E. coli peritonitis syndrome (EPS), fibrinous polyserositis (FP) or no clinical signs (NCS) are indicated. Chicken type is given between brackets as layer (L), broiler (B), broiler-breeder (BB), and specified pathogen-free broiler-breeder (SPF BB).
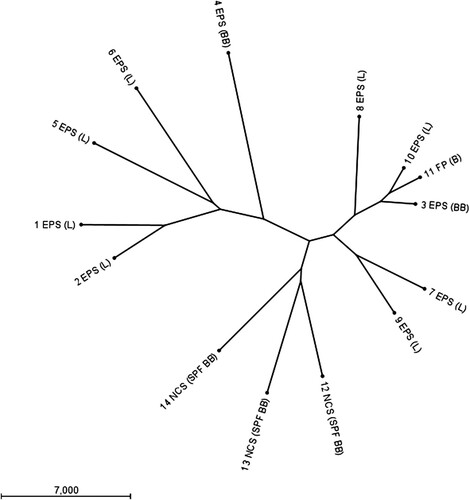
Raw sequence reads were uploaded to the National Center for Biotechnology Information Sequence Read Archive and BioProject PRJNA731634: “In vivo typing of Escherichia coli obtained from laying chickens with the E. coli peritonitis syndrome”. References to BioSample numbers for individual samples can be found in .
Serotyping
Results are presented in . Bone marrow and pericardium strains, which were isolated during different years and from flocks housed in different places in the Netherlands were predominantly of O-groups 1, 2, and 78 (8/10) in combination with H-types 7, 6 and 1, and 4, respectively. The cloaca and caecum strains had no O-groups in common with the bone marrow and pericardium strains.
Biotyping in hens
Results are outlined in . Mortality in groups inoculated with bone marrow strains ranged from 1/16 (6%; groups 17 and 18) to 16/16 (100%; groups 1, 3, and 4). Mean death time (MDT) varied from 25 to 114 h between groups. Death time of individual birds ranged from 17 to 189 h. The mortality rate seemed to be negatively correlated with MDT: mortality >50% was associated with relatively short MDTs, while mortality <50% was coupled to relatively long MDTs (except for Group 17).
The pericardium strain caused the death of 11/16 (69%) hens in both groups 21 and 22. No mortality occurred in the caecum strain-inoculated groups (groups 15 and 16), nor in the groups inoculated with the cloaca strains (groups 23–28), except for group 28 in which one hen died.
Mortality in groups inoculated with the same E. coli strain, but with different doses, did not differ significantly (P > 0.08).
Additive mortalities of the two groups of hens inoculated with the same E. coli strain, are given in . Based on mortality, the strains could be divided into three virulence classes: very virulent strains that induced 59–100% mortality, one moderately virulent strain that caused 38% mortality (bone marrow strain 5) and avirulent, and low virulent strains inducing 0–6% mortality. Seven of the nine bone marrow strains studied (numbers 1–4, 6, 7, and 10) and the pericardium strain (strain 11) belong to the first class, while the caecum strain (avirulent strain 8), the cloaca strains (avirulent strains 12 and 13 and low virulent strain 14) and one bone marrow strain (low virulent strain 9) belong to the third class. Mortalities in the three virulence classes were significantly different from each other (P < 0.03).
Table 4. Biotyping of chicken E. coli strains in productive SPF White Leghorn hens and SPF White Leghorn embryos.
Results of post-mortem examination and bacteriological analysis of the 221 hens that died during the experiment are presented in . Ninety-nine percent of the hens (219/221) had a productive or degenerated ovary, two hens (1%) had an inactive ovary. Post-mortem lesions were dominated by peritonitis (184/221 = 83%) and airsacculitis (212/221 = 96%). Pneumonia (121/221 = 55%), pericarditis (130/221 = 59%) and perihepatitis (112/221 = 51%) also occurred frequently. Salpingitis was observed incidentally, in just four birds (2%).
E. coli was isolated from the bone marrow of 208/221 (94%) dead birds, and, in the vast majority, numerous E. coli colonies were obtained (194/208 = 93%).
LD50
Results of the assessment of the LD50 of three E. coli EPS strains obtained from bone marrow (, strains 1, 3, and 6) are presented in . The LD50 of strain 1 (serogroup O1), strain 3 (serogroup O78), and strain 6 (serogroup O2) were <2.7 (Trial 2), 3.8 (Trial 2) and 5.3 log10 CFU (Trial 1), respectively.
Mean death times after inoculation with 8.0–8.2 log10 CFU per hen ranged from 19 to 40 h between E. coli strains (, Trial 1). Generally, decreasing E. coli doses led to increasing MDTs ().
Lesions in hens that died following E. coli inoculation and E. coli reisolations from bone marrow of these birds, were quantitatively and qualitatively similar to those observed in the biotyping experiment (). All these hens (128/128) had active or degenerated ovaries. In Trial 1, 17/120 (14%) birds that had been E. coli-inoculated survived. Thirteen of these surviving hens (13/17 = 76%) showed lesions at post-mortem examination, consisting of ovary degeneration, peritonitis, airsacculitis, pneumonia, and perihepatitis. These lesions occurred in various combinations. In Trial 2, 15/40 (38%) birds survived. Three of the surviving birds (20%) demonstrated lesions, namely pericarditis and/or airsacculitis. All placebo inoculated hens (Trial 1) survived and did not show lesions at post-mortem examination. Bacteriological examination of bone marrow of surviving hens, including placebo inoculated birds, all yielded negative results in both trials.
Biotyping in embryos
Results are presented in . Mortality did not occur in placebo (PPS)-inoculated and in non-inoculated embryos. The bone marrow and pericardium strains induced mortality ranging from 13/20 (65%) to 19/20 (95%) in Trial 1, and from 12/20 (60%) to 18/20 (90%) in Trial 2. Mortality rates of embryos inoculated with bone marrow strains or the pericardium strain did not differ significantly within and between trials. The caecum and cloaca strains caused mortality ranging from 0/20 (0%) to 6/20 (30%) in Trial 1, and from 0/20 (0%) to 5/20 (25%) in Trial 2. The mortality induced with the latter strains differed significantly from that caused by bone marrow and pericardium strains (P < 0.03), except E. coli strain 12 in Trial 1. Mortality occurred predominantly during the second day after inoculation (day 16 of incubation) in all cases and decreased rapidly thereafter. By 4 days after inoculation (18 days of incubation) mortality had stopped almost completely. MDT was between 44 and 58 h in both trials and was independent of the origin of the E. coli strain. Although mortality in Trial 2 was somewhat lower compared to Trial 1, reproducibility of results was considered good, as no statistical differences existed between mortality of embryos in both trials inoculated with the same E. coli strain (P > 0.09).
Table 5. Biotyping of chicken E. coli strains in SPF White Leghorn embryos.
Bacteriological analysis of groups of embryos each inoculated with one of the 14 E. coli strains, revealed numerous E. coli colonies in all groups in all five embryos examined, including the groups in which no mortality occurred (groups inoculated with E. coli strains 8 and 14). Bacteria were not isolated from placebo- inoculated and uninoculated embryos.
Comparison of biotyping in hens with biotyping in embryos
allows a convenient comparison of results obtained in hens with those obtained in embryos. The bone marrow and pericardium strains, which were all classified as virulent based on mortality rates of 6–100% in hens, induced 60–95% mortality in embryos. Classification of virulent E. coli strains as very virulent, moderately virulent, and low virulent strains based on mortality in hens, was not possible based on mortalities in embryos.
The caecum strain (strain 8) and two cloaca strains (strains 12 and 13), which were classified as avirulent based on their inability to induce mortality in hens, caused 0–30% mortality in embryos. One cloaca strain (strain 14), which caused 3% (1/32) mortality in hens, and therefore was considered to be of low virulence, did not kill any of the embryos.
Discussion
Until now, it was unknown if the ability of E. coli to induce EPS was restricted to particular strains or merely the result of high inoculation doses. Here we show that EPS cannot be induced by all E. coli strains as strains obtained from the caecum and cloaca failed to induce the disease. Moreover, it appears that significant differences exist in the ability to induce EPS. Based on mortality in the productive layers of the biotyping experiment, only bone marrow strains and the pericardium strain were shown to be virulent. Virulent strains could be divided into very virulent, moderately virulent, and low virulent strains. Even within the very virulent strains, substantial differences in LD50 were found (<2.7–5.3 log10 CFU).
In the present study, E. coli inoculations were performed by the intratracheal route, resulting in mortality rates ranging from 6% to 100% accompanied by severe and widespread EPS lesions in dead birds when EPS bone marrow strains or the pericardium strain were inoculated at a dose of 7.8–9.2 log10 CFU per hen. In contrast, Cox et al. (Citation2021) searching for an appropriate challenge model to examine the efficacy of their vaccine against egg layer peritonitis in chickens, could induce neither mortality nor colibacillosis lesions in mature SPF WL hens following intratracheal inoculation at a dose of 9 log10 CFU E. coli per hen. The three E. coli challenge strains they used were isolated from organs of dead birds with colibacillosis (a turkey and chickens) and belonged to serogroups O1, O2, and O78. The discrepancy between our results and those of Cox et al. (Citation2021) is likely due to substantial differences in virulence between the E. coli strains used in both studies. The strains applied by Cox et al. (Citation2021) were of (very) low virulence as intravenous inoculation of 8 log10 CFU per bird caused neither mortality nor pathology. Therefore, these strains can by no means be considered as representatives of EPS strains.
Translocation of intestinal bacteria, such as E. coli, to mesenteric lymphnodes, spleen, liver, kidney, and cardiac blood as early as 5 min after death has been reported in mice (Heimesaat et al., Citation2012). These bacteria might be isolated instead of the virulent bacteria responsible for the disease when performing bacteriological analysis of internal organs of dead animals, which might have happened to the strains used by Cox et al. (Citation2021). Published data on the translocation of intestinal bacteria to bone marrow after death are lacking in chickens. However, in humans, the presence of gut-associated bacteria was demonstrated in the bone marrow of decomposing bodies, which were sampled at ≥27 days post-mortem (Damann et al., Citation2015), while in pigs internal bioerosion of femora consistent with exposure to enteric putrefactive bacteria was found at ≥6 months post-mortem (White & Booth, Citation2014). Most likely, intestinal bacteria reach bone marrow at a late stage of decomposition due to the physical protection of organic substrates, as bones are the slowest decomposing body tissue (Javan et al., Citation2019). Therefore, in case of mortality due to septicaemia, bacteriological analysis of marrow of bones distant from the body cavity, such as the femur, should be preferentially performed.
The embryo lethality test enabled a clear distinction between virulent and avirulent strains, which could not be attributed to differences in replication ability of these two classes of strains in embryos, as both dead and surviving embryos yielded abundant E. coli colonies. This observation is in agreement with the findings of Rezaee et al. (Citation2021), who showed that no significant differences in E. coli load existed between virulent and avirulent strains in yolk and liver of chicken embryos after inoculation by the allantoic route at day 12 of incubation.
The embryo lethality test did not discriminate within the virulent strains. This is likely due to the limited immune competence of the embryo compared to the adult hen. Nevertheless, the embryo lethality test can be considered as a useful alternative for biotyping E. coli in productive hens. In this regard, the results of the embryo lethality tests performed by Nolan et al. (Citation1992) and by Gibbs and Wooley (Citation2003) showed a fairly good correlation with those of a chicken lethality test and are in agreement with our results. Powell and Finkelstein (Citation1966) however, found no association between enteropathogenicity of E. coli strains and virulence in chicken embryos. A likely explanation may be that the E. coli strains examined were of human origin, while biotyping was done in a distant species.
The E. coli isolated from a broiler with fibrinous polyserositis (strain 11 = E. coli 506) was able to induce EPS in layer birds, indicating that colibacillosis in broilers may represent a source of EPS strains for layer birds. However, the reverse is also a possibility.
E. coli 506 has been used during the past decades in a number of experiments. This strain was classified as very virulent non-opportunistic in 8-day-old commercial broilers (Velkers et al., Citation2005) and productive layers (present study), as low virulent non-opportunistic in 7-day-old chickens of a slow-growing broiler line (Ask et al., Citation2006), and as opportunistic in 34-day-old commercial broilers (Matthijs et al., Citation2003). In view of the foregoing, virulence qualifications of E. coli should always be made in conjunction with breed, age, and the presence of egg production in the birds involved.
Virulent E. coli strains isolated during different years and at different geographic locations were predominantly of serogroups O1, O2, and O78, which rank among common serogroups previously associated with disease (O1, O2, O18, O35, O36, O78, and O111) (Heller & Drabkin, Citation1977; Barbieri et al., Citation2015). Virulent and avirulent strains had no O-serogroups in common. However, an increasing number of other serogroups are associated with disease in chickens (Blanco et al., Citation1998; Zhao et al., Citation2005) (strains 7 and 9 present study), questioning the usefulness of serogroup as a marker for virulence. Also, serogroups are of hardly any value in epidemiological studies as genetic differences occur within serogroups.
WGS analysis was done to demonstrate that all strains used in our study were genetically distinct. Further molecular analyses of EPS-inducing strains and avirulent strains are subjects of ongoing research.
Almost all hens (99.4%) that died in the present study due to infection with strains that caused EPS had an active or recently active (degenerated) ovary, which underlines the (per)acute nature of EPS and previous results (Landman et al., Citation2013; Landman et al., Citation2014). In 93% of these hens E. coli was isolated from bone marrow, mostly in abundant quantities.
In conclusion, here we show that EPS-inducing E. coli strains can be highly virulent without the need for any trigger, and may thus cause severe primary colibacillosis. However, E. coli strains obtained from clinically healthy birds did not.
Acknowledgements
We thank Stichting AviNed, Nieuwegein, Netherlands for funding the biotyping of E. coli in hens and embryos, and Vaxxinova Research & Development GmbH, Münster, Germany for financially supporting the LD50 trials. We also thank H. Bataille, T. T. M. Manders, H. van de Sande, M. Schellekens and W. Vije for their technical assistance.
Disclosure statement
No potential conflict of interest was reported by the authors.
References
- Al-Ankari, A.R., Bradbury, J.M., Naylor, C.J., Worthington, K.J., Payne-Johnson, C. & Jones, R.C. (2001). Avian pneumovirus infection in broiler chicks inoculated with Escherichia coli at different time intervals. Avian Pathology, 30, 257–267.
- Ask, B., Van der Waaij, E.H., Stegeman, J.A. & Van Arendonk, J.A. (2006). Genetic variation among broiler genotypes in susceptibility to colibacillosis. Poultry Science, 85, 415–421.
- Barbieri, N.L., De Oliveira, A.L., Tejkowski, T.M., Pavanelo, D.B., Matter, L.B., Pinheiro, S.R., Vaz, T.M., Nolan, L.K., Logue, C.M., De Brito, B.G. & Horn, F. (2015). Molecular characterization and clonal relationships among Escherichia coli strains isolated from broiler chickens with colisepticemia. Foodborne Pathogens and Disease, 12, 74–83.
- Blanco, J.E., Blanco, M., Mora, A., Jansen, W.H., García, V., Vázquez, M.L. & Blanco, J. (1998). Serotypes of Escherichia coli isolated from septicaemic chickens in Galicia (northwest Spain). Veterinary Microbiology, 61, 229–235.
- Cox, G.J.M., Griffith, B., Reed, M., Sandstrom, J.D., Peterson, M.P., Emery, D. & Straub, D.E. (2021). A vaccine to prevent egg layer peritonitis in chickens. Avian Diseases, 65, 198–204.
- Damann, F.E., Williams, D.E. & Layton, A.C. (2015). Potential use of bacterial community succession in decaying human bone for estimating postmortem interval. Journal of Forensic Science, 60, 844–850.
- Dhillon, A.S. & Jack, O.K. (1996). Two outbreaks of colibacillosis in commercial caged layers. Avian Diseases, 40, 742–746.
- Fabricant, J. & Levine, P.P. (1962). Experimental production of complicated chronic respiratory disease infection (“air sac” disease). Avian Diseases, 6, 13–23.
- Gibbs, P.S. & Wooley, R.E. (2003). Comparison of the intravenous chicken challenge method with the embryo lethality assay for studies in avian colibacillosis. Avian Diseases, 47, 672–680.
- Gross, W.B. (1961). The development of “air sac disease”. Avian Diseases, 5, 431–439.
- Heimesaat, M.M., Boelke, S., Fischer, A., Haag, L.M., Loddenkemper, C., Kühl, A.A., Göbel, U.B. & Bereswill, S. (2012). Comprehensive postmortem analyses of intestinal microbiota changes and bacterial translocation in human flora associated mice. PLoS One, 7, e40758.
- Heller, E.D. & Drabkin, N. (1977). Some characteristics of pathogenic E. coli strains. The British Veterinary Journal, 133, 572–578.
- ISO6887. (1983). Microbiology – General guidance for the preparation of dilutions for microbiological examination. Geneva: International Standard Organisation.
- ISO7402. (1985). Microbiology – General guidance for the enumeration of Enterobacteriaceae without resuscitation – MPN technique and colony count technique. Geneva: International Standard Organisation.
- Javan, G.T., Finley, S.J., Tuomisto, S., Hall, A., Benbow, M.E. & Mills, D. (2019). An interdisciplinary review of the thanatomicrobiome in human decomposition. Forensic Science, Medicine and Pathology, 15, 75–83.
- Joensen, K.G., Tetzschner, A.M., Iguchi, A., Aarestrup, F.M. & Scheutz, F. (2015). Rapid and easy in silico serotyping of Escherichia coli isolates by use of whole-genome sequencing data. Journal of Clinical Microbiology, 53, 2410–2426.
- Landman, W.J.M., Buter, G.J., Dijkman, R. & Van Eck, J.H.H. (2014). Molecular typing of avian pathogenic Escherichia coli colonies originating from outbreaks of E. coli peritonitis syndrome in chicken flocks. Avian Pathology, 43, 345–356.
- Landman, W.J.M. & Cornelissen, R.A. (2006). [Escherichia coli salpingitis and peritonitis in layer chickens: an overview]. Tijdschrift voor Diergeneeskunde, 131, 814–822.
- Landman, W.J.M., Heuvelink, A. & Van Eck, J.H.H. (2013). Reproduction of the Escherichia coli peritonitis syndrome in laying hens. Avian Pathology, 42, 157–162.
- Landman, W.J.M. & Van Eck, J.H.H. (2015). The incidence and economic impact of the Escherichia coli peritonitis syndrome in Dutch poultry farming. Avian Pathology, 44, 370–378.
- Landman, W.J.M. & Van Eck, J.H.H. (2017). The efficacy of inactivated Escherichia coli autogenous vaccines against the E. coli peritonitis syndrome in layers. Avian Pathology, 46, 658–665.
- Manders, T.T.M., Matthijs, M.G.R., Veraa, S., Van Eck, J.H.H. & Landman, W.J.M. (2021). Success rates of inoculation of the various compartments of embryonated chicken eggs at different incubation days. Avian Pathology, 50, 61–77.
- Matthijs, M.G.R., Van Eck, J.H.H., De Wit, J.J., Bouma, A. & Stegeman, J.A. (2005). Effect of IBV-H120 vaccination in broilers on colibacillosis susceptibility after infection with a virulent Massachusetts-type IBV strain. Avian Diseases, 49, 540–545.
- Matthijs, M.G.R., Van Eck, J.H.H., Landman, W.J.M. & Stegeman, J.A. (2003). Ability of Massachusetts-type infectious bronchitis virus to increase colibacillosis susceptibility in commercial broilers: a comparison between vaccine and virulent field virus. Avian Pathology, 32, 473–481.
- Nolan, L.K., Wooley, R.E., Brown, J., Spears, K.R., Dickerson, H.W. & Dekich, M. (1992). Comparison of a complement resistance test, a chicken embryo lethality test, and the chicken lethality test for determining virulence of avian Escherichia coli. Avian Diseases, 36, 395–397.
- Powell, C.J., Jr. & Finkelstein, R.A. (1966). Virulence of Escherichia coli strains for chick embryos. Journal of Bacteriology, 91, 1410–1417.
- Raviv, Z., Ferguson-Noel, N., Laibinis, V., Wooten, R. & Kleven, S.H. (2007). Role of Mycoplasma synoviae in commercial layer Escherichia coli peritonitis syndrome. Avian Diseases, 51, 685–690.
- Reed, L.J. & Muench, H. (1938). A simple method of estimating fifty percent end points. American Journal of Epidemiology, 27, 493–497.
- Rezaee, M.S., Liebhart, D., Hess, C., Hess, M. & Paudel, S. (2021). Bacterial infection in chicken embryos and consequences of yolk sac constitution for embryo survival. Veterinary Pathology, 58, 71–79.
- Statistix®. (2010). User’s manual Statistix 8.2 for Windows [Computer software]. Retrieved from www.statistix.com.
- Van Eck, J.H.H. & Goren, E. (1991). An Ulster 2C strain-derived Newcastle disease vaccine: vaccinal reaction in comparison with other lentogenic Newcastle disease vaccines. Avian Pathology, 20, 497–507.
- Vandekerchove, D., De Herdt, P., Laevens, H. & Pasmans, F. (2004a). Colibacillosis in caged layer hens: characteristics of the disease and the aetiological agent. Avian Pathology, 33, 117–125.
- Vandekerchove, D., Herdt, P.D., Laevens, H., Butaye, P., Meulemans, G. & Pasmans, F. (2004b). Significance of interactions between Escherichia coli and respiratory pathogens in layer hen flocks suffering from colibacillosis-associated mortality. Avian Pathology, 33, 298–302.
- Velkers, F.C., Te Loo, A.J., Madin, F. & Van Eck, J.H.H. (2005). Isopathic and pluralist homeopathic treatment of commercial broilers with experimentally induced colibacillosis. Research in Veterinary Science, 78, 77–83.
- White, L. & Booth, T.J. (2014). The origin of bacteria responsible for bioerosion to the internal bone microstructure: results from experimentally-deposited pig carcasses. Forensic Science International, 239, 92–102.
- Zanella, A., Alborali, G.L., Bardotti, M., Candotti, P., Guadagnini, P.F., Martino, P.A. & Stonfer, M. (2000). Severe Escherichia coli O111 septicaemia and polyserositis in hens at the start of lay. Avian Pathology, 29, 311–317.
- Zhao, S., Maurer, J.J., Hubert, S., De Villena, J.F., McDermott, P.F., Meng, J., Ayers, S., English, L. & White, D.G. (2005). Antimicrobial susceptibility and molecular characterization of avian pathogenic Escherichia coli isolates. Veterinary Microbiology, 107, 215–224.
- Zhou, Z., Alikhan, N.F., Mohamed, K., Fan, Y., Agama Study, G. & Achtman, M. (2020). The EnteroBase user’s guide, with case studies on Salmonella transmissions, Yersinia pestis phylogeny, and Escherichia core genomic diversity. Genome Research, 30, 138–152.