ABSTRACT
Mycoplasma anserisalpingitidis is economically the most important pathogenic Mycoplasma species of waterfowl in Europe and Asia. The lack of commercially available vaccines against M. anserisalpingitidis had prompted this study with the aim to produce temperature-sensitive (ts+) clones as candidates for an attenuated live vaccine. The production of ts+ clones was performed by N-methyl-N′-nitro-N-nitrosoguanidine (NTG)-induced mutagenesis of Hungarian M. anserisalpingitidis field isolates. The clones were administered via eye-drop and intracloacally to 33-day-old geese. Colonization ability was examined by PCR and isolation from the trachea and cloaca, while the serological response of the birds was tested by ELISA. Pathological and histopathological examinations were performed in the eighth week after inoculation. Whole-genome sequence (WGS) analysis of the selected clone and its parent strain was also performed. NTG-treatment provided three ts+ mutants (MA177/1/11, MA177/1/12, MA271). MA271 was detected at the highest rate from cloacal (86.25%) and tracheal (30%) samples, while MA177/1/12 and MA271 elicited remarkable serological responses with 90% of the birds showing seroconversion. Re-isolates of MA271 remained ts+ throughout the experiment. Based on these properties, clone MA271 was found to be the most promising vaccine candidate. WGS analysis revealed 59 mutations in the genome of MA271 when compared to its parent strain, affecting both polypeptides involved in different cellular processes and proteins previously linked to bacterial fitness and virulence. Although further studies are needed to prove that MA271 is in all aspects a suitable vaccine strain, it is expected that this ts+ clone will contribute to the control of M. anserisalpingitidis infection.
RESEARCH HIGHLIGHTS
Three M. anserisalpingitidis ts+ vaccine candidates were produced by NTG-mutagenesis.
Clone MA271 was able to colonize geese and induce a serological response.
MA271 re-isolates remained ts+ during the 8-week-long experiment.
WGS analysis revealed 59 mutations in the genome of MA271.
Introduction
Mycoplasma anserisalpingitidis (formerly Mycoplasma anserisalpingitis or M. sp. strain 1220) is the most important mycoplasma pathogen of waterfowl, both clinically and economically. As a member of the class Mollicutes, this bacterium is characterized by the lack of a cell wall, a small size, complex nutritional requirements and the ability to persist in the host, thus generating chronic infections (Razin & Jacobs, Citation1992). M. anserisalpingitidis was first isolated in 1983 from a gander with phallus inflammation in Hungary, where it has been mainly studied thenceforth, and got its novel species status as M. anserisalpingitidis in 2020 (Stipkovits et al., Citation1984; Varga et al., Citation1986; Volokhov et al., Citation2020). It has also been confirmed to be present in other European countries such as the Czech Republic, France, Germany, Poland, Russian Federation and Ukraine and it was recently described in China and Vietnam as well, assuming a possible worldwide distribution (Stipkovits et al., Citation1986; Sprygin et al., Citation2012; Gyuranecz et al., Citation2020; Grózner et al., Citation2021). M. anserisalpingitidis can be detected in asymptomatic geese and, rarely, ducks (Grózner et al., Citation2019). However, clinical manifestation of mycoplasmosis can occur during periods of excessive stress linked to crowding, inadequate housing, sexual maturation and breeding season. Inflammation of the cloaca and phallus, salpingitis and testicular atrophy are the most frequent signs of disease in infected breeders and up to 50-100% of sexually-active ganders can develop clinical disease (Stipkovits et al., Citation1986; Hinz et al., Citation1994; Stipkovits & Kempf, Citation1996). The number of fertile eggs drops significantly, the production of eggs with abnormal shells increases and embryo mortality rates may reach up to 40–60% (Dobos-Kovács et al., Citation2009). Peritonitis, airsacculitis and neurological signs can also be seen in both adult and young birds, although with a lower frequency (Stipkovits et al., Citation1993).
At present, the control of the disease relies on the improvement of housing conditions and antibiotic therapy that often also includes metaphylactic antibiotic application. Unfortunately, the quick development of multi-drug resistance in M. anserisalpingitidis has been experienced in Europe and China (Grózner et al., Citation2016; Gyuranecz et al., Citation2020). Furthermore, the increased selective pressure when using antimicrobials to treat mycoplasmosis probably also affects the drug resistance profile of other pathogenic bacteria colonizing geese. Thus, food safety and human health perspectives associated with the risk of spread of multi-host bacteria exhibiting antimicrobial resistance highlight the importance of vaccination as a long-term solution in controlling mycoplasmosis in waterfowl.
Live attenuated vaccines have been successfully used against avian mycoplasmosis over the last decades. They proved to be safe, highly efficacious and capable of inducing long-term protection (Whithear, Citation1996). Some of these attenuated vaccine strains evolved by natural selection, like the M. gallisepticum F, 6/85 and K vaccine strains (Yamamoto & Adler, Citation1958; Evans et al., Citation1992; Evans & Hafez, Citation1992; Raviv et al., Citation2008), while the M. synoviae MS1 vaccine strain was produced by spontaneous mutagenesis through in vitro passages (Dijkman et al., Citation2014). The M. gallispeticum ts-11 and M. synoviae MS-H temperature-sensitive vaccine strains were developed by chemical mutagenesis using N-methyl-N′-nitro-N-nitrosoguanidine (NTG) (Whithear et al., Citation1990a, Citation1990b, Citation1990c; Markham et al., Citation1998a, Citation1998b, Citation1998c; Morrow et al., Citation1998).
The NTG is one of the most potent mutagens, which can interact with proteins and nucleic acids causing alkylation and base-pair substitution. As a result of treatment with this mutagenic agent, clones of microbes that exhibit temperature-sensitivity may evolve and these temperature-sensitive (ts+) strains can be selected by the use of permissive culture conditions.
The aim of this study was to produce ts+ M. anserisalpingitidis clones using NTG-induced mutagenesis, and to evaluate their ability to colonize geese, elicit an adequate host immune response and eventually compare the whole-genome sequences (WGSs) of the selected clone and its parent strain.
Materials and methods
Mycoplasma anserisalpingitidis strains and culture
Three field isolates of M. anserisalpingitidis were used in this study. All three strains were isolated from domestic geese in Hungary. MYCAV177 was isolated from the phallus lymph of a gander with phallus inflammation in 2015 (03/07/2015, Cered, Hungary), MYCAV271 was also isolated from phallus lymph in 2016 (28/04/2016, Szentes, Hungary) while MYCAV342 was isolated from the trachea and serous membrane of a dead goose in 2017 (17/03/2017, Hajdúböszörmény, Hungary).
The medium used for the isolation and propagation of the organism consisted of Mycoplasma broth medium (pH 7.8) (Thermo Fisher Scientific Inc./Oxoid Inc./, Waltham, MA, USA) supplemented with 0.5% (w/v) sodium pyruvate, 0.5% (w/v) glucose, 0.005% (w/v) phenol red and 0.15% (w/v) L-arginine hydrochloride.
Phallus lymph and tissue samples were washed in 2 ml broth, incubated at 37°C overnight, filtered through a 0.65 µm pore size syringe filter (Sartorius GmbH, Goettingen, Germany) and incubated at 37°C. After acidic colour change (2 days), the cultures were inoculated onto solid Mycoplasma medium (passage 1) (Thermo Fisher Scientific Inc./Oxoid Inc./) supplemented with 0.15% (w/v) L-arginine hydrochloride and were incubated at 37°C with 5% CO2 until visible colonies appeared (4 days). Well separated colonies were picked and incubated at 37°C in 1 ml of broth (passage 2) until acidic colour change occurred (1 day). The samples were tested by four different PCR tests specific for M. anatis, M. anseris, M. anserisalpingitidis and M. cloacale, i.e. Mycoplasma species infecting waterfowl (Grózner et al., Citation2019). The presence of other, contaminant Mycoplasma species was ruled out by a universal Mycoplasma PCR system targeting the 16S/23S rRNA intergenic spacer region in Mollicutes followed by sequencing on an ABI Prism 3100 automated DNA sequencer (Applied Biosystems, Foster City, CA, USA), sequence analysis and BLAST search (https://blast.ncbi.nlm.nih.gov/Blast.cgi) (Lauerman et al., Citation1995). The strains (100 µl of the purified cultures) were propagated at 37°C in 10 ml broth (passage 3) until acidic colour change (1 day), and 1 ml aliquots of broth cultures were stored at −70°C until use.
Chemical mutagenesis and selection of temperature-sensitive clones
Strains were exposed to 1-methyl-3-nitro-1-nitrosoguanidine (NTG) (TCI Europe N.V., Zwijndrecht, Belgium) using the method described by Nonomura and Imada, Citation1982), with minor modifications. The strains (200 µl of the passage 3 cultures) were propagated at 37°C in 10 ml broth until late logarithmic phase (16 h incubation). The cultures were divided into 1.5 ml aliquots, then the cells were harvested by centrifugation at 9000× g for 9 min and the supernatant was removed. The pellets were treated with 1.5 ml phosphate-buffered saline (PBS) containing NTG at the following dilutions: 200, 400, 800, 1600 and 3200 µg/ml. After thorough mixing and incubation of solutions for 30 min at 37°C protected from light, the organisms were centrifuged at 9000 × g for 9 min and the pellet was washed with 2 ml PBS three times. The washed sediment was suspended in 1.5 ml of fresh broth, mixed well, incubated at 37°C for 15 min and divided into 500 µl aliquots, two of which were frozen and stored at −70°C.
The number of colour changing units (CCU/ml) of each aliquot of the three cultures treated with different concentrations of NTG was determined at 37°C on 96-well microtitre plates using the broth microdilution method by titrating a 10-fold dilution series of the Mycoplasma suspension in quadruplicate. The plates were checked daily and the final results were read after 9 days. The highest dilution which showed colour change was considered to contain 10° CCU/ml of bacteria (Hannan, Citation2000).
All aliquots of the three cultures treated with different concentrations of NTG were inoculated onto solid Mycoplasma medium (passage 1) (Thermo Fisher Scientific Inc./Oxoid Inc./). The plates were incubated at 33°C with 5% CO2 until visible colonies appeared (5 days). Well separated colonies were picked and incubated at 33°C in 1 ml broth (passage 2) until acidic colour change (2 days). The 1 ml culture was divided into 500 µl aliquots, one of them was frozen and stored at −70°C.
The number of CCU of each clone was determined at 33°C and 39.5°C as described above. Temperature-sensitivity was defined as a minimum of 100-fold decrease in CCU at 39.5°C (non-permissive temperature) compared to 33°C (Nonomura & Imada, Citation1982). The ts+ clones (200 µl of the purified cultures) were propagated at 33°C in 20 ml broth (passage 3) until acidic colour change (2 days), and 1 ml aliquots of broth cultures were deposited in the pre-masterseed collection, stored at −70°C. One ml was further inoculated into 250 ml broth (passage 4), incubated at 33°C for 2 days and deposited in 1 ml aliquots at −70°C as masterseed stock.
Experimental birds and vaccination
Bird procedures were reviewed and approved by Government Office of Pest County Animal Ethics Committee under approval number PE/EA/688-3/2020. Forty-eight 1-day-old goslings were acquired from a commercial goose hatchery (Tranzit-Ker Zrt., Debrecen, Hungary). Upon arrival, and subsequently at 2 and 4 weeks of age, swab samples were taken from the cloaca of all birds to verify their Mycoplasma-free status. The samples were tested by four different PCR systems specific for M. anatis, M. anseris, M. anserisalpingitidis and M. cloacale, Mycoplasma species infecting waterfowl (Grózner et al., Citation2019). Universal Mycoplasma PCR was also performed to exclude the presence of other mycoplasmas (Lauerman et al., Citation1995). Tiamulin, a pleuromutilin antibiotic highly effective against avian Mycoplasma species (Grózner et al., Citation2016; Kreizinger et al., Citation2017) was administered in the drinking water (5 g/3 l) for 3 days upon arrival to further reduce the probability of the presence of any vertically transmitted Mycoplasma species.
Birds were housed in two groups separated by gender until 4 weeks of age, when birds were divided into six groups in three separate rooms. To test the colonization ability of the three vaccine candidates (MA177/1/11, MA177/1/12 and MA271 from parent strains MYCAV177 and MYCAV271), three groups of 10 birds were used. The two positive control groups (exposed to infection with the two wild parent strains) consisted of 2 × six birds, and the negative control group (treated with sterile PBS) consisted of six birds. Each group included geese and ganders in equal numbers. The birds were identified individually by foot rings.
At 33 days of age (day 0 of the experiment) the birds were vaccinated with MA177/1/11, MA177/1/12 and MA271 ts+ clones or infected with MYCAV177 and MYCAV271 wild parent strains. The infectious substances were prepared as follows: 2 ml of masterseed cultures were inoculated into 100 ml Mycoplasma broth medium (passage 5) (Thermo Fisher Scientific Inc./Oxoid Inc.). Broth cultures were incubated at 33°C for the ts+ clones and at 37°C for the wild parent strains until acidic colour change (2 days), then stored at −70°C. The cultures were thawed at room temperature immediately before administration.
The birds were inoculated via bilateral eye-drops (60 μl in each eye) and injected intracloacally with 1 ml broth culture or sterile PBS. The number of CCU was determined at 33°C and 39.5°C for the clones and at 37°C for the wild parent strains immediately after administration, and temperature-sensitivity of the clones was verified as described above.
Development of a TaqMan real-time PCR assay for detecting M. anserisalpingitidis.
The whole-genome sequences (WGSs) of 81 M. anserisalpingitidis isolates, including the ATCC BAA-2147 type strain, were determined previously in our laboratory using the Illumina NextSeq 500 next-generation sequencing platform (Illumina Inc., San Diego, CA, USA) (Kovács et al., Citation2020). Draft genomes of the isolates were assembled with the SPAdes program version 3.13.0 (Centre for Algorithmic Biotechnology, Institute of Translational Biomedicine, St. Petersburg State University, St. Petersburg, Russia) (Bankevich et al., Citation2012).
The sequences of 25 housekeeping genes were obtained from the 81 M. anserisalpingitidis WGSs, then aligned and analysed as target candidates for primer and probe design using Geneious Prime 2019.2.3 software (Biomatters Ltd., Auckland, New Zealand) (Kearse et al., Citation2012) and selected according to the following criteria: (1) melting temperature of the probe must be at least 5–10°C higher than that of the primers; (2) sequences must be species-specific. Primer design was performed using NetPrimer software (http://www.premierbiosoft.com/netprimer), and the specificity of the primers and probes was checked in silico using BLAST NT algorithm (https://blast.ncbi.nlm.nih.gov/Blast.cgi).
The specificity of the PCR assays was tested on the following avian Mycoplasma and Acholeplasma type strains: M. anatis (NCTC 10156), M. anseris (ATCC 49234), M. cloacale (NCTC 10199), M. columbinasale (ATCC 33549), M. columborale (ATCC 29258), M. gallinaceum (ATCC 33550), M. gallisepticum (ATCC 19610), M. gallopavonis (ATCC 33551), M. imitans (ATCC 51306), M. iners (ATCC 19705), M. iowae (ATCC 33552), M. meleagridis (NCTC 10153), M. pullorum (ATCC 33553), M. synoviae (NCTC 10124) and Acholeplasma laidlawii (NCTC 10116). In addition, 14 M. anserisalpingitidis, nine M. anatis, five M. cloacale and five M. anseris isolates were included.
In order to test the sensitivity of the PCR assays, tenfold dilution (in nuclease-free water) of M. anserisalpingitidis ATCC BAA-2147 type strain was used in the range of 106–100 template copy number/μl. Template copy number (corresponding to genomic equivalents (GE in the current study), as the target gene was present in a single copy in the genomes) was calculated with the help of an online tool (http://cels.uri.edu/gsc/cndna.html) based on the DNA concentration measured by Nanodrop 2000 Spectrophotometer (Thermo Fisher Scientific Inc.) and the genome size of M. anserisalpingitidis ATCC BAA-2147 (959,110 bp).
In order to evaluate the performance of the designed assay in mixed infections, the DNA of the M. anserisalpingitidis type strain (106 GE) mixed in a ratio of 1:1 with M. anseris, M. anatis and M. cloacale type strains was tested.
Background information of strains used for the development of the M. anserisalpingitidis-specific TaqMan real-time PCR assay are provided in Supplementary Table 1.
PCR identification, re-isolation and temperature-sensitivity testing of M. anserisalpingitidis strains
Tracheal and cloacal swab samples were collected weekly from all birds for 8 weeks post-vaccination. Each swab was washed and incubated in 1 ml PBS for 1 h at room temperature before further processing.
Half (0.5 ml) of the PBS solution was centrifuged for 9 min at 9000 × g to harvest the cells and DNA was extracted with the ReliaPrep gDNA Tissue Miniprep System (Promega Inc., Madison, WI, USA) according to the manufacturers’ instructions. DNA samples were tested by the developed M. anserisalpingitidis-specific TaqMan real-time PCR assay. The remaining 0.5 ml of PBS solution was inoculated into 1 ml Mycoplasma broth medium (Thermo Fisher Scientific Inc./Oxoid Inc./), filtered through a 0.65 µm pore size syringe filter (Sartorius GmbH) then incubated at 33°C (for the samples from birds vaccinated with the ts+ clones) or 37°C (for the samples from birds infected with the parent strains or treated with PBS), and examined twice a day for 9 days or until the appearance of acidic colour change indicative of M. anserisalpingitidis growth.
One M. anserisalpingitidis-positive culture derived from each ts+ clone-vaccinated group was selected weekly, inoculated onto solid Mycoplasma medium (Thermo Fisher Scientific Inc./Oxoid Inc./) and incubated at 33°C with 5% CO2 until visible colonies appeared (5 days). Well-separated colonies were picked and incubated at 33°C in 1 ml Mycoplasma broth medium (Thermo Fisher Scientific Inc./Oxoid Inc./) until acidic colour change (2 days). The number of CCU was determined at 33°C and 39.5°C and temperature-sensitivity of the clones was evaluated as described above.
Blood collection and serological testing
Blood samples were collected from the brachial wing vein from all birds 4, 6 and 8 weeks post-vaccination. Sera were separated from the blood samples and stored at −20°C until further processing.
An indirect enzyme-linked immunosorbent assay (ELISA) designed for the detection of M. anserisalpingitidis-infection was developed for the serological monitoring of the experimental birds. Whole bacterial cells (mixture of five different M. anserisalpingitidis field isolates in PBS) were used as coating antigens. M. anserisalpingitidis cell suspension was lysed at 1:1 ratio in a mixture of 10% 2-beta-mercaptoethanol (Serva, Heidelberg, Germany), 20% glycerol (pH 6.8) (Reanal, Budapest, Hungary), and 4% sodium dodecyl sulphate (SDS) (Tomtec Plastics, Budapest, Hungary). The denaturation of the suspension was performed in a 96°C water bath for 4 min (Dénes et al., Citation2003). Antigen was diluted at 1:80 ratio in PBS (pH 7.2), and 200 µl aliquots were measured into the wells of the polystyrene 96-well flat-bottomed hydrophilic ELISA plate (TOMTEC Calibsorp, Tomtec Plastics). After an overnight incubation at 10°C, the plates were washed three times with washing buffer (Diavet Ltd., Budapest, Hungary). Blocking was performed by 200 µl of 1% skim milk solution (Sigma-Aldrich Inc., St. Louis, MO, USA). After incubation at 37°C for 90 min, three washing steps with the washing buffer followed. Serum samples were diluted in PBS at a 1:40 ratio, and 200 µl measured into the wells. The plates were incubated at 37°C for 90 min, then washed three times. As a next step, 200 µl goat anti-duck IgG (H + L) horseradish peroxidase (HRP) conjugate (SeraCare Life Sciences Inc., Milford, MA, USA) diluted at 1:3200 in PBS was measured into the wells. After 90 min incubation at 37°C the plates were washed as described above. The enzyme activity was visualized by the addition of 100 µl of tetramethylbenzidine (TMB) (Diavet Ltd.). After incubation for 10 min, the reaction was stopped by the addition of 100 µl of 2.5 M H2SO4 (Diavet Ltd.) solution. Evaluation was done with a Multiskan FC reader (Thermo Fisher Scientific Inc.) using a 450 nm optical filter. Each plate contained a negative control (serum sample of Mycoplasma-free, 4-week-old goose) and a positive control (serum sample of 3-year-old breeding goose infected with M. anserisalpingitidis). Each sample was tested in duplicate in antigen-coated wells and in single in non-coated wells. The optical density (OD) value of the antigen-uncoated well was subtracted from the mean of the OD values of antigen-coated wells (Terato et al., Citation2016; Waritani et al., Citation2017). Samples with OD values 1.4–1.6 times higher than the OD value of the negative control were considered ambiguous, while samples showing more than 1.6-fold increase in the OD value compared to the negative control were considered positive.
Clinical, gross pathological, and histopathological examination of the birds and PCR testing of tissue samples
Geese were monitored twice daily for clinical signs associated with M. anserisalpingitidis infection during the experiment. At the end of the 8th week geese were euthanized and detailed pathological examination was performed. Tissue samples (nasal cavity, trachea, air sac, lung, heart, liver, spleen, kidney, small intestine (jejunum), cloaca, bursa of Fabricius, gonads (testicle or ovary), oviduct, brain, and in one case tarsal joint) were collected for histological examination and fixed in 10% neutral buffered formalin. As the ganders were immature, the size of the phallus made it possible to submit it only for PCR testing and not for histology. Four μm thick sections of formalin-fixed and paraffin-embedded tissue samples were stained with haematoxylin and eosin and examined by light microscopy. Swabs from all pathological lesions were streaked onto Columbia blood and Coliform agar plates (Biolab Zrt., Budapest, Hungary) for bacterial culture. To detect M. anserisalpingitidis, tissue samples were collected from the following organs of each goose: air sac, lung, liver, spleen, bursa of Fabricius, testicle and phallus or ovary and oviduct. Samples were stored at −20°C until further processing. After homogenization in PBS, DNA was extracted with the ReliaPrep gDNA Tissue Miniprep System (Promega Inc.) according to the manufacturers’ instructions. DNA samples were subjected to M. anserisalpingitidis-specific TaqMan real-time PCR assay.
Whole-genome sequencing of the parent strain MYCAV271 and its ts+ clone MA271 and comparison of their WGSs
Whole genome sequences of the parent strain MYCAV271 and its ts+ clone MA271 were determined using next-generation sequencing technologies. Whole-genome sequencing was performed on Oxford Nanopore MinION Mk1C (Oxford Nanopore Technologies Ltd, Oxford, UK) instrument and Illumina NextSeq 500 platform (Illumina Inc., San Diego, CA, USA) with TG NextSeq 500/550 High Output Kit v2.
First, the quality of the raw sequence reads was checked with the FastQC software version 0.11.8 (https://www.bioinformatics.babraham.ac.uk/projects/fastqc/), and the short reads from the Illumina sequencing were trimmed and paired with Geneious Prime version 2019.2.3. The whole genomes were assembled from the Oxford Nanopore sequencing with Flye software version 2.8.3 (Kolmogorov et al., Citation2019) and corrected using the prepared Illumina reads in Geneious Prime version 2019.2.3 by mapping the reads to the genome. The circularity of the genomes was checked in silico using the Geneious Prime software by searching for overlapping sequences at the 5′ and 3′ end of the genomes.
The completed whole genomes were annotated with the NCBI Prokaryotic Genome Annotation Pipeline (PGAP) software (Tatusova et al., Citation2016) and RAST server (Aziz et al., Citation2008), and aligned with the Geneious Prime version 2019.2.3 and MAUVE software version 20150226 (Darling et al., Citation2004). Single nucleotide polymorphisms (SNPs) were extracted from the alignment for further analysis. The mutations found with Geneious Software were used as cross-reference for the SNPs found by MAUVE.
Nucleotide sequence accession numbers
The whole-genome sequence of MYCAV271 was submitted to GenBank under accession number CP083178, while MA271 can be accessed under CP082234.
Results
Temperature-sensitive clones
The number of colonies decreased in proportion with the increasing NTG concentration used for the treatment of the strains (). Altogether, 78 single colonies were picked from the agar plates inoculated with cultures treated with 200, 400 and 800 µg/ml NTG. No colonies were detected after treatment with NTG concentrations of 1600 and 3200 µg/ml. Each propagated colony was tested for temperature-sensitivity. Three clones showed ts+ phenotype after repeating the titration three times. Two ts+ clones, named MA177/1/11 and MA177/1/12 were derived from MYCAV177 treated with 200 µg/ml NTG solution while clone MA271 was derived from MYCAV271 treated with 200 µg/ml NTG solution. No ts+ mutant was found among the MYCAV342-derived clones. Viable counts of the three ts+ clones at 33°C and 39.5°C are included in .
M. anserisalpingitidis-specific TaqMan real-time PCR assay
From the 25 housekeeping genes analysed in the study, DNA-directed RNA polymerase subunit alpha (rpoA) was found to be suitable for the design of the primers and probe. The sequences of the designed primers and probe were: forward primer 5′- GCA CAC TTT ATG GTT ATA GG -3′, reverse primer 5′- TAC AGA AAG ATT TAA TTG GTT GA -3′ and probe 5′-FAM- TCT GCG AAA ACA TCA ACT TTC AT -BHQ-1-3′. The size of the amplicon was 123 bp.
Table 1. Viable count (CCU/ml) of the strains MYCAV177, MYCAV271 and MYCAV342 determined at 37°C by broth microdilution method after treatment with different concentrations of N-methyl-N′-nitro-N-nitrosoguanidine (NTG).
Table 2. Viable count (CCU/ml) of the parent strains, ts+ clones and their re-isolates determined by broth microdilution method at different time-points (post-NTG: immediately after NTG-treatment, day 0: immediately after the administration to birds, week 1–8: after re-isolation).
The PCR assay was carried out in 12 μl total volume, containing 2 μl target DNA sample, 1.5 10X AmpliTaq Gold Buffer (Thermo Fisher Scientific Inc.), 1.5 μl MgCl2 (25 mM, Thermo Fisher Scientific Inc.), 0.5 μl dNTP (10 mM, Qiagen), 0.5 μl of each primer (10 pmol/μl), 0.25 μl of the probe (10 pmol/μl), 0.1 μl AmpliTaq Gold DNA polymerase (5 U/μl; Thermo Fisher Scientific Inc.) and nuclease-free water.
Thermocycling parameters were 95°C for 10 min, followed by 40 cycles at 95°C for 15 s, 58°C for 1 min. The PCR assay was optimized on a Bio-Rad CFX96 Touch Real-Time PCR Detection System (Bio-Rad Inc., Hercules, CA, USA) with the CFX Maestro software version 1.1 (Bio-Rad Inc.).
According to the sensitivity testing, the minimum GE detectable in the samples was 101. Specificity testing did not demonstrate cross-amplification with any other examined Mycoplasma/Acholeplasma strain.
Colonization of the respiratory tract and cloaca
Results of the M. anserisalpingitidis-specific TaqMan real-time PCR assay and isolation from the cloacal and tracheal swab samples of each bird can be found in detail in Supplementary Tables 2 and 3. Data are summarized in .
Table 3. Number of M. anserisalpingitidis-positive birds determined by PCR and/or isolation from the cloaca (CL) and trachea (TR) at 1–8 weeks (w1-8) post-vaccination by the MA177/1/11, MA177/1/12 and MA271 ts+ clones, or post-infection by the MYCAV177 and MYCAV271 wild strains.
After vaccination with the MA177/1/11 clone, M. anserisalpingitidis was detected from the cloaca at the 3rd week in 2/10 birds, while it could be detected from the trachea at the 1st week in 1/10 and at the 7th week in 2/10 birds (). In case of vaccination with the clone MA177/1/12, M. anserisalpingitidis could be recovered at all test time-points from the cloaca of 7–10/10 birds. The presence of the organism in the trachea was detected sporadically with a maximum of 4/10 positive birds at the end of the experiment (). In case of birds vaccinated with the MA271 clone, M. anserisalpingitidis could be detected at all examined time-points from the cloaca of 7–9/10 birds, and from the trachea of 1–8/10 birds ().
After the infection with MYCAV177, M. anserisalpingitidis could be detected at all examined time-points from the cloaca of 5–6/6 birds, while it could be detected from the trachea at the 1st week in 4/6 and at the 7th week in 5/6 birds, and sporadically in between (). In case of the MYCAV271-infection, M. anserisalpingitidis was detected at all time-points from the cloaca of all birds (5/5, as one bird from this group was culled 1 day after the vaccination due to causes unrelated to the experiment, as revealed during pathological examinations), while it could be found in the trachea of 2–5/5 geese during the first 7 weeks (). No M. anserisalpingitidis could be detected by isolation or PCR from the cloaca or trachea of the PBS-treated birds at any time-point.
When the weekly re-isolation was successful from the cloaca of at least one bird the ts+ phenotype of each clone was determined. Clone MA177/1/11 was re-isolated successfully from the cloaca only once (at the 3rd week), while re-isolation of clone MA177/1/12 and MA271 was successful every week. The number of CCU of the re-isolates determined at 33°C and 39.5°C by broth microdilution method is shown in .
Serological monitoring
Results of the M. anserisalpingitidis-specific indirect ELISA testing of serum samples are presented in detail in Supplementary Table 4. Data are summarized in . In the MA177/1/11-vaccinated group, ambiguous serological responses were detected 4 weeks after vaccination, then two birds became seropositive by the 6th week and two additional geese were considered seropositive at the 8th week post-vaccination. Most of the birds vaccinated with ts+ clones MA177/1/12 and MA271 showed a specific immune response by the 4th week post-vaccination (number of seropositive birds: 7–8/10). However, a lower number of seropositive birds was detected (n = 4–5/10) at the 6th week post-vaccination, with a slight increase by the end of the experiment (n = 6/10) (). After the infection with MYCAV177, geese showed a serologic response in 3–4/6 cases, and none of the birds was seronegative for the entire duration of the experiment. All samples from MYCAV271 wild strain infected birds were positive at all time-points. No seropositive birds were detected in the PBS-treated control group ().
Table 4. Results of the serological monitoring of serum samples by indirect M. anserisalpingitidis ELISA from birds at 4-, 6- and 8-weeks (w4, w6, w8) post-inoculation of the ts+ clones MA177/1/11, MA177/1/12 and MA271, of the wild strains MYCAV177 and MYCAV271 and of phosphate-buffered saline (PBS) (negative control group).
Clinical, gross pathological, and histopathological examination and PCR testing of tissue samples
During the experiment, neither respiratory nor venereal clinical signs associated with mycoplasmosis could be observed in any of the birds. A day after the vaccination, one bird from the MYCAV271-infected positive control group was culled due to causes unrelated to the experiment (pathological examination revealed generalized Staphylococcus spp. infection, no Mycoplasma was detected from the trachea or cloaca). This bird was therefore excluded from the evaluation.
Gross air sac lesions were observed at necropsy in three birds vaccinated with clone MA177/1/11. Fibrin deposition on the pericardium or unilateral fibrinous rhinitis was seen in two additional geese from the same group. Airsacculitis was observed in four birds vaccinated with clone MA177/1/12, accompanied by peritonitis (n = 1), catarrhal lungs (n = 1), and arthritis in the hock joint (n = 2) as additional findings. Among the birds vaccinated with clone MA271, only one goose had airsacculitis, while two MYCAV177-infected, and three MYCAV271-infected birds showed inflammation in the air sacs. In the negative control group, only one bird exhibited small-scale fibrin deposition in the thoracic air sacs. No other bacteria were isolated from the respiratory and joint lesions.
Histopathological examination revealed microscopic lesions in all groups (Supplementary Table 5). Mild catarrhal rhinitis and tracheitis with lymphohistiocytic infiltration in the lamina propria could be observed in birds from all groups, except the negative control group. In the air sacs, lympho-histiocytic infiltration and lymphoid follicle proliferation with or without moderate-to-severe fibrinous inflammation was detected in all groups except the negative control geese. Moderate lymphoid follicle proliferation was detected in the lung samples of all groups, although the number of foci was lower in negative control birds. Catarrhal inflammation was also detected in the lung of 3–5 birds from the three vaccinated groups. Mild lymphocytic depletion of the spleen could be observed in 11 birds in various groups. Three birds in the MA271-vaccinated group also showed focal lymphocyte necrosis in the spleen. In the bursa of Fabricius, mild lymphocytic depletion could be detected in four birds from the MA271-vaccinated group and in one goose from the MYCAV177-infected group. In the oviduct of two geese from MA271-vaccinated, and one goose from MA177/1/12-vaccinated group, proliferation of lymphocytes, histiocytes and granulocytes was observed. No histopathological lesions were detected in the brain, small intestine (jejunum), cloaca and testicles of the birds in any of the groups.
Results of the M. anserisalpingitidis-specific TaqMan real-time PCR assay in the tissue samples is shown in detail in Supplementary Table 6. Data are summarized in .
Table 5. M. anserisalpingitidis-specific PCR results of the different organ samples of geese vaccinated by the ts+ clones MA177/1/11, MA177/1/12 and MA271, or infected by the wild strains MYCAV177 and MYCAV271.
In the MA177/1/11-vaccinated group only four samples (from different organs of different birds) showed M. anserisalpingitidis-positivity by PCR. In case of the geese vaccinated by the MA177/1/12 and MA271 clones, or infected by the MYCAV177 or MYCAV271 wild strains, tissue samples from the bursa of Fabricius and the genital tract showed a higher proportion of positivity (33–80%) (). Samples from the negative control group were negative with the M. anserisalpingitidis-specific PCR.
Whole-genome sequencing of the parent strain MYCAV271 and its ts+ clone MA271 and comparison of their WGSs
The Oxford Nanopore sequencing resulted in 43,686 reads with a mean length of 5775.7 bp (minimum 104 bp, maximum 99,511 bp) and a mean PHRED score of 17.8 for the MYCAV271 parent strain, and 57,811 reads with a mean length of 7831.9 bp (minimum 158 bp, maximum 114,252 bp) and a mean PHRED score of 18.5 for its MA271 ts+ clone. The Illumina sequencing yielded 10,287,635 paired end reads for MYCAV271 with the mean length of 104.9 bp (minimum 35 bp, maximum 151 bp) and a mean PHRED score of 35.4, while there were 26,645,430 paired end reads for MA271 with a mean length of 150 bp (minimum 150 bp, maximum 150 bp) and a mean PHRED score of 36.7. The length of the assembled genome of MYCAV271 was 916,347 bps and it was 916,353 bps for MA271. The GC content was 26.8% for both the MYCAV271 parent strains and its MA271 ts+ clone. The mean coverage of the Illumina reads was 512.4 × for the MYCAV271 parent strain and 2345.5 × for the MA271 clone.
The circular nature of the genomes was confirmed in silico by overlapping sequences at the 5′ and 3′ end of the genomes. The MAUVE alignment divided the genomes into two locally collinear blocks (LCBs); however, these followed the same arrangement in both genomes, which also confirms the completeness and circularity of the genomes.
Results of the WGS-analysis and comparison between the ts+ clone MA271 and its parent strain MYCAV271 can be found in Supplementary Table 7. The search with MAUVE software combined with Geneious as a cross-referencing step found a total of 51 SNPs in the genome of the MA271 clone when compared to its parent strain MYCAV271. The majority (42/51) of these SNPs were guanine (G) to adenine (A) transitions. Indel events were searched by Geneious software, which revealed two deletions and six insertions in the WGS of the MA271 clone. Most of the mutations were non-synonymous, resulting in alterations of the encoded proteins or hypothetical proteins in the form of amino acid substitution (n = 37), amino acid insertion (n = 1), truncation (n = 1) or frame-shift (n = 7). Two non-synonymous SNPs were found in the phosphotransferase system (PTS): one in the coding region of the N-acetylglucosamine-specific component (IIA, IIB, IIC component) and another one in the trehalose-specific component (IIB, IIC component). Besides these, non-synonymous SNPs were also detected in the genes encoding “outer surface protein of unknown function, cellobiose operon” and neutral endopeptidase O. The number of synonymous mutations was 10, while only three SNPs were found in non-coding intergenic regions.
Discussion
Control of avian Mycoplasma infection comprises three general aspects: prevention, medication and vaccination (Kleven, Citation2008). Prevention is difficult in geese flocks as the birds are kept in production for 3–4 years on open pastures in contact with wild birds (Sawicka-Durkalec et al., Citation2021). Mycoplasmas can hardly be eliminated from infected flocks by antibiotic treatment and medication should not be applied for longer periods in order to prevent the development and spread of antibiotic resistance. Therefore, the intensive goose production industry is clearly in need of an effective M. anserisalpingitidis vaccine. The advantage of attenuated live vaccines over inactivated vaccines is that the organism can multiply in the host and thus continuously stimulate the immune system both locally (mucosal immunity) and systemically creating long-lasting immunity (Greenberg et al., Citation1974; Kleven, Citation2008). Moreover, some of these vaccines are able to spread horizontally to unvaccinated birds, possibly creating a more efficient vaccination strategy (Levisohn and Kleven, Citation2000). This study therefore aimed to produce and evaluate ts+ M. anserisalpingitidis clones as attenuated live vaccine candidates.
In vaccinated birds, colonization of the attenuated live vaccine strain might prevent superinfection with virulent strains, manifestation of clinical disease, vertical transmission of the agent and, in some cases, it might be able to displace wild-type strains already present (Levisohn and Kleven, Citation2000). Characterization of the colonization ability of the vaccine candidates is therefore crucial.
To investigate the colonization ability of the M. anserisalpingitidis vaccine candidates, a M. anserisalpingitidis-specific TaqMan real-time PCR assay and isolation from cloacal and tracheal swab samples of each bird were used. Interestingly, in some cases, M. anserisalpingitidis could be isolated from PCR-negative samples (Supplementary Tables 2 and 3), despite the fact that molecular methods are supposed to be more sensitive than culture. Indeed, TaqMan real-time PCR is considered to be a reliable test with high sensitivity and reproducibility. Conversely, the outcome of isolation depends on several factors and, in contrast to PCR, requires the presence of viable bacteria in the sample. A possible explanation for the discrepancy in our findings could be due to the fact that the detection limit of the developed TaqMan real-time PCR assay is 101 template copy number/μl, while, under ideal conditions, the presence of a single viable organism in the sample may be sufficient for successful Mycoplasma isolation. Therefore, in some cases, culture may yield a positive result along with PCR-negativity. For easier data interpretation, the results of PCR testing and culture are summarized in and evaluated in this merged form.
The ts+ clone MA177/1/11 showed low colonization ability, as it could be detected from the cloaca only at the 3rd week in 20% of the swab samples originating from vaccinated birds (two positive cloacal samples / 80 total cloacal samples = 2.5% of the total cloacal samples). In contrast, the other two clones were detected at all test time-points and were able to colonize all of the vaccinated birds. Clones MA177/1/12 and MA271 were detectable in 70–100% of the cloacal samples week-by-week. Clone MA177/1/12 was present in 76.25% (61 positive cloacal samples/80 total cloacal samples) of the total cloacal samples, while clone MA271 showed the best colonization ability, as it was detectable in 86.25% (69 positive samples/80 total cloacal samples) during the 8-week-long experiment.
M. anserisalpingitidis was not continuously detectable by PCR in the cloaca of certain birds (Supplementary Table 2), suggesting changes in the size of the M. anserisalpingitidis cloacal population during the 8-week experiment. Similar curves of detection were observed on group level in birds inoculated with the parent strains or clones MA177/1/12 and MA271, indicating similar colonization ability of the ts+ clones to their parent strains (). M. anserisalpingitidis positivity of samples reached its maximum at the 1st week in the MYCAV271-infected group, 2nd week in the MYCAV177-infected group, and 3rd week in the MA271-vaccinated group and did not decline till the end of the 8-week study. The MA177/1/12 clone was also detectable in a high proportion of samples throughout the experiment with a peak at the 4th week and an increase at the end of the study. Among the ts+ clones, MA271 showed the highest ability to colonize the trachea, as it was detectable in 30% of the total samples (24 positive samples/80 total trachea samples) during the 8-week-long experiment (MA177/1/11: 3.75%; MA177/1/12: 12.5%).
Figure 1. Percentage of M. anserisalpingitidis-positive birds at 1–8 weeks post-vaccination by the ts+ clone MA177/1/11, MA177/1/12 and MA271, and post-infection by the parent strains MYCAV177 and MYCAV271 by PCR and/or isolation from cloacal swab samples.
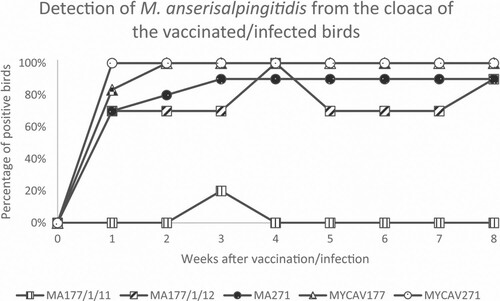
A beneficial feature of the temperature-sensitive mutant M. synoviae (MS-H) and M. gallisepticum (ts-11) vaccine strains is that they initially replicate (and elicit an immune response) in the upper respiratory tract rather than at core body temperature (Whithear et al., Citation1990a, Citation1990b, Citation1990c; Markham et al., Citation1998a, Citation1998b, Citation1998c; Morrow et al., Citation1998). Temperature-sensitivity can also be used as a phenotypic marker for differentiating the attenuated mutants from wild strains. However, temperature-sensitivity is not a stable characteristic, as ts+ mutants can lose this feature in vitro by culturing at non-permissive temperature, and in vivo in the vaccinated flock as well. Despite the loss of ts+ phenotype, these mutants usually do not revert their pathogenicity, as NTG causes multiple linked mutations in addition to temperature-sensitivity and these contribute to the attenuation (Noormohammadi et al., Citation2003). Hence, temperature-sensitivity seems to be a suitable selection marker for the high degree of attenuation in the case of M. anserisalpingitidis as well, although M. anserisalpingitidis most likely begins its growth in the cloacal region, at a higher temperature.
The determination of the number of CCU at two different temperatures by broth microdilution method yielded inconsistent results for MA177/1/12, as only the re-isolates originating from samplings on the 2nd and 3rd weeks showed ts+ phenotype among the weekly isolated clones. The sole cloacal re-isolate of MA177/1/11 (sample from the 3rd week) showed ts+ phenotype, while re-isolates of clone MA271 remained temperature-sensitive for the whole experiment.
ELISA test for serological monitoring of the birds vaccinated with the MA177/1/11 clone showed a weak serological response throughout the experiment, as only 40% of vaccinated birds showed seropositivity. In contrast, birds vaccinated with clones MA177/1/12 or MA271 had similar, remarkable serological responses, with 90% of the birds showing seroconversion in these two groups, and only one bird per group remaining seronegative at all examined time-points during the experiment. The number of seropositive birds showed a decrease from week 4 to week 6 and then slightly increased by week 8 (). Based on the number of seropositive samples, no distinction can be made in the extent of the serological response elicited by these two clones. Nevertheless, it is important to note that the protection against avian mycoplasmas is not necessarily correlated with the level of serum antibodies (Whithear, Citation1996).
Figure 2. Percentage of seropositive birds at 4-, 6- and 8-weeks post-vaccination by the ts+ clone MA177/1/11, MA177/1/12 and MA271, and post-infection by the parent strains MYCAV177 and MYCAV271 by indirect M. anserisalpingitidis ELISA.
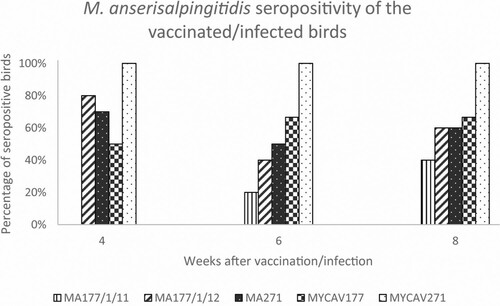
No clinical signs were seen after the administration of the parent strains or the derived clones. Gross pathological lesions could be observed in the air sacs of some birds from all groups including one bird from the negative control group as well, and histopathological changes were detected in the air sacs and lungs of the birds of all infected groups. Nevertheless, Mycoplasma could not be detected in the air sacs or lungs of birds, except in four cases with mild pathological changes. Other pathogens and/or environmental factors could have contributed to the development of these lesions, but chronic mycoplasmosis with low number of bacteria in the lesions cannot be ruled out either. Further studies must be performed to evaluate whether the observed lesions are caused by other factors, are tolerable side effects of immunization (as clinical signs were not observed) or the vaccine candidates were not sufficiently attenuated.
No gross pathological lesions were detected in the genitals, while the clones and the parent strains were detected from many samples of the genital tract (phallus and oviduct) and also the bursa of Fabricius (). The highest proportion of positive samples detected in these organs (60–70%) among the vaccinated birds originated from the ts+ clone MA271-inoculated group (). Accordingly, histopathological changes of lymphocytic depletion of the bursa of Fabricius were overrepresented in MA271-vaccinated birds and lympho-histiocytic proliferation in the oviduct also occurred in this group. The bursa of Fabricius, which is the dorsal diverticulum of the cloaca, is well-known as a primary lymphoid organ in fowl and a major channel through which environmental antigens stimulate the immune system. Several studies suggested that the resistance against avian mycoplasmas is mediated by the bursal-dependent lymphoid cells (Whithear, Citation1996).
Figure 3. Percentage of M. anserisalpingitidis-positive tissue samples originating from the genital tract and the bursa of Fabricius of geese vaccinated by the ts+ clone MA177/1/11, MA177/1/12 and MA271, and infected by the parent strains MYCAV177 and MYCAV271.
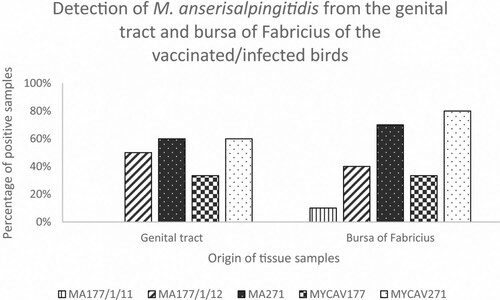
In the case of well-studied avian mycoplasmas, the local immune response appears to be of greater importance for immunity to infection than the systemic response. In general, the association between local antibodies and immunity is much stronger than with serum antibody levels (Morrow et al., Citation1998). Thus, the high incidence of the MA271 clone in the genital tract is a desirable outcome of the vaccination. Also, eye-associated lymphoid tissue including the Harderian gland, as well as the mucosa-associated lymphoid tissue on the eye surface, in the lachrymal gland and in the lachrymal duct, are exposed to antigenic stimulation via eye-drop administered vaccines (Morrow et al., Citation1998). In addition, the vaccine travels down the lachrymal duct and stimulates the nasal-associated lymphoid tissue and a further proportion is deposited in the choana and upper region of the trachea, from which M. anserisalpingitidis can be isolated sporadically. The intra-cloacal route combined with eye-drops can be therefore a reliable way of administration to deliver effective doses of the vaccine to local immune-target sites of all vaccinated birds.
Based on the ability to rapidly colonize a high proportion of geese, the consistent temperature-sensitivity and the elicited serological response, the clone MA271 was selected for further investigations as the most promising vaccine candidate. Analysis of WGS data revealed several mutations in the genome of the clone MA271 when compared to its parent strain MYCAV271. In addition to numerous hypothetical proteins, NTG-mutagenesis affected the coding regions of polypeptides involved in a wide range of cellular processes such as cell division, synthesis and hydrolysis reactions, regulation of osmotic changes and active transport, different defensive mechanisms or energy production processes. In attenuated live vaccine strains, genes encoding proteins which might be linked to bacterial fitness and virulence are usually worthy of special attention. As such, N-acetylglucosamine- and trehalose-specific components of the PTS system, the “outer surface protein of unknown function, cellobiose operon” and neutral endopeptidase O coding regions were affected by the NTG-mutagenesis. In pathogenic microbes, virulence factors are strictly regulated by the nutrient availability to the cell. The bacterial PTS catalyzes the phosphorylation of incoming sugar substrates concomitant with their translocation across the cell membrane (Deutscher et al., Citation2006). A connection between the PTS and the virulence of certain pathogens was suggested due to a regulatory mechanism enabling bacteria to increase their fitness by optimizing growth rates in natural environments providing different nutrients (Stülke and Hillen, Citation1999; Trappetti et al., Citation2017). Cellobiose metabolism is also linked to the virulence properties in numerous bacterial pathogens. The putative cellobiose operon enables uptake of cellobiose and allows growth when cellobiose is provided as the sole carbon source in minimal medium (Hasan et al., Citation2021). The neutral endopeptidase O (PepO) is a metallopeptidase, which has broad substrate specificity for several oligopeptides and plays an important role in turning off peptide signalling events at the cell surface. PepO can be involved in regulating the expression of numerous secreted and surface-associated virulence factors (Brouwer et al., Citation2018). It has also been described that proteolysis of short signalling peptides by PepO might contribute to biofilm formation of other bacteria (Wilkening et al., Citation2016).
MA271 was patent deposited at Leibniz-Institut DSMZ-Deutsche Sammlung von Mikroorganismen und Zellkulturen GmbH, Germany under ID number DSM 33810 and patented under number: P 21 00357 at Hungarian Intellectual Property Office. Nevertheless, further studies are needed to confirm that MA271 is, in all aspects, a safe and effective live M. anserisalpingitidis vaccine strain, which could contribute to the improvement of the waterfowl industry as well as the advancement of human and bird health.
Supplemental Material
Download MS Excel (58.1 KB)Acknowledgements
The linguistic accuracy of the manuscript was kindly revised by Károly Erdélyi.
Disclosure statement
No potential conflict of interest was reported by the author(s).
Additional information
Funding
References
- Aziz, R.K., Bartels, D., Best, A.A., DeJongh, M., Disz, T., Edwards, R.A., Formsma, K., Gerdes, S., Glass, E.M., Kubal, M., Meyer, F., Olsen, G.J., Olson, R., Osterman, A.L., Overbeek, R.A., McNeil, L.K., Paarmann, D., Paczian, T., Parrello, B., Pusch, G.D., Reich, C., Stevens, R., Vassieva, O., Vonstein, V., Wilke, A. & Zagnitko, O. (2008). The RAST server: rapid annotations using subsystems technology. BMC Genomics, 9, 75.
- Bankevich, A., Nurk, S., Antipov, D., Gurevich, A.A., Dvorkin, M., Kulikov, A.S., Lesin, V.M., Nikolenko, S.I., Pham, S., Prjibelski, A.D., Pyshkin, A.V., Sirotkin, A.V., Vyahhi, N., Tesler, G., Alekseyev, M.A. & Pevzner, P.A. (2012). SPAdes: a new genome assembly algorithm and its applications to single-cell sequencing. Journal of Computational Biology, 19, 455–477.
- Brouwer, S., Cork, A.J., Ong, C.Y., Barnett, T.C., West, N.P., McIver, K.S. & Walker, M.J. (2018). Endopeptidase PepO regulates the SpeB cysteine protease and is essential for the virulence of invasive M1T1 Streptococcus pyogenes. Journal of Bacteriology, 200, e00654–17.
- Darling, A.C.E., Mau, B., Blattner, F.R. & Perna, N.T. (2004). Mauve: multiple alignment of conserved genomic sequence with rearrangements. Genome Research, 14, 1394–1403.
- Dénes, B., Tenk, M., Tekes, L., Varga, I., Ferenczné, I.P. & Stipkovits, L. (2003). Recognition of multiple Mycoplasma bovis antigens by monoclonal antibodies. Hybridoma and Hybridomics, 22, 11–16.
- Deutscher, J., Francke, C. & Postma, P.W. (2006). How phosphotransferase system-related protein phosphorylation regulates carbohydrate metabolism in bacteria. Microbiology and Molecular Biology Reviews, 70, 939–1031.
- Dijkman, R., Feberwee, A., van Kasteren, T. & Landman, W. (2014). Development and validation of a PCR test to differentiate between Mycoplasma synoviae vaccine strain MS1 and M. synoviae field isolates. 20th congress of the International Organization for Mycoplasmology (IOM), Blumenau, Brazil.
- Dobos-Kovács, M., Varga, Z., Czifra, G. & Stipkovits, L. (2009). Salpingitis in geese associated with Mycoplasma sp. strain 1220. Avian Pathology, 38, 239–243.
- Evans, R.D. & Hafez, Y.S. (1992). Evaluation of a Mycoplasma gallisepticum strain exhibiting reduced virulence for prevention and control of poultry mycoplasmosis. Avian Diseases, 36, 197–201.
- Evans, R.D., Hafez, Y.S. & Schreurs, C.S. (1992). Demonstration of the genetic stability of a Mycoplasma gallisepticum strain following in vivo passage. Avian Diseases, 36, 554–560.
- Greenberg, H., Helms, C.M., Brunner, H. & Chanock, R.M. (1974). Asymptomatic infection of adult volunteers with a temperature sensitive mutant of Mycoplasma pneumoniae. Proceedings of the National Academy of Sciences of the United States of America, 71, 4015–4019.
- Grózner, D., Kovács, ÁB, Wehmann, E., Kreizinger, Z., Bekő, K., Mitter, A., Sawicka, A., Jánosi, S., Tomczyk, G., Morrow, C.J., Bányai, K. & Gyuranecz, M. (2021). Multilocus sequence typing of the goose pathogen Mycoplasma anserisalpingitidis. Veterinary Microbiology, 254, 108972.
- Grózner, D., Kreizinger, Z., Sulyok, K.M., Rónai, Z., Hrivnák, V., Turcsányi, I., Jánosi, S. & Gyuranecz, M. (2016). Antibiotic susceptibility profiles of Mycoplasma sp. 1220 strains isolated from geese in Hungary. BMC Veterinary Research, 12, 170.
- Grózner, D., Sulyok, K.M., Kreizinger, Z., Rónai, Z., Jánosi, S., Turcsányi, I., Károlyi, H.F., Kovács, ÁB, Kiss, M.J., Volokhov, D. & Gyuranecz, M. (2019). Detection of Mycoplasma anatis, M. anseris, M. cloacale and Mycoplasma sp. 1220 in waterfowl using species-specific PCR assays. PLoS One, 14, e0219071.
- Gyuranecz, M., Mitter, A., Kovács, ÁB, Grózner, D., Kreizinger, Z., Bali, K., Bányai, K. & Morrow, C.J. (2020). Isolation of Mycoplasma anserisalpingitidis from swan goose (Anser cygnoides) in China. BMC Veterinary Research, 16, 178.
- Hannan, P.C. (2000). Guidelines and recommendations for antimicrobial minimum inhibitory concentration (MIC) testing against veterinary Mycoplasma species. International Research Programme on Comparative Mycoplasmology. Veterinary Research, 31, 373–395.
- Hasan, M.K., Dhungel, B.A. & Govind, R. (2021). Characterization of an operon required for growth on cellobiose in Clostridioides difficile. Microbiology, 167, 8.
- Hinz, K.H., Pfützner, H. & Behr, K.P. (1994). Isolation of mycoplasmas from clinically healthy adult breeding geese in Germany. Journal of Veterinary Medicine Series B, 41, 145–147.
- Kearse, M., Moir, R., Wilson, A., Stones-Havas, S., Cheung, M., Sturrock, S., Buxton, S., Cooper, A., Markowitz, S., Duran, C., Thierer, T., Ashton, B., Meintjes, P. & Drummond, A. (2012). Geneious Basic: an integrated and extendable desktop software platform for the organization and analysis of sequence data. Bioinformatics, 28, 1647–1649.
- Kleven, S.H. (2008). Control of avian mycoplasma infections in commercial poultry. Avian Diseases, 52, 367–374.
- Kolmogorov, M., Yuan, J., Lin, Y. & Pevzner, P.A. (2019). Assembly of long, error-prone reads using repeat graphs. Nature Biotechnology, 37, 540–546.
- Kovács, ÁB, Kreizinger, Z., Forró, B., Grózner, D., Mitter, A., Marton, S., Bali, K., Sawicka, A., Tomczyk, G., Bányai, K. & Gyuranecz, M. (2020). The core genome multi-locus sequence typing of Mycoplasma anserisalpingitidis. BMC Genomics, 21, 403.
- Kreizinger, Z., Grózner, D., Sulyok, K.M., Nilsson, K., Hrivnák, V., Benčina, D. & Gyuranecz, M. (2017). Antibiotic susceptibility profiles of Mycoplasma synoviae strains originating from Central and Eastern Europe. BMC Veterinary Research, 13, 342.
- Lauerman, L.H., Chilina, A.R., Closser, J.A. & Johansen, D. (1995). Avian mycoplasma identification using polymerase chain reaction amplicon and restriction fragment length polymorphism analysis. Avian Diseases, 39, 804–811.
- Levisohn, S. & Kleven, S.H. (2000). Avian mycoplasmosis (Mycoplasma gallisepticum). Revue Scientifique et Technique, 19, 425–442.
- Markham, J.F., Morrow, C.J., Scott, P.C. & Whithear, K.G. (1998a). Safety of a temperature-sensitive clone of Mycoplasma synoviae as a live vaccine. Avian Diseases, 42, 677–681.
- Markham, J.F., Morrow, C.J. & Whithear, K.G. (1998b). Efficacy of a temperature-sensitive Mycoplasma synoviae live vaccine. Avian Diseases, 42, 671–676.
- Markham, J.F., Scott, P.C. & Whithear, K.G. (1998c). Field evaluation of the safety and efficacy of a temperature-sensitive Mycoplasma synoviae live vaccine. Avian Diseases, 42, 682–689.
- Morrow, C.J., Markham, J.F. & Whithear, K.G. (1998). Production of temperature-sensitive clones of Mycoplasma synoviae for evaluation as live vaccines. Avian Diseases, 42, 667–670.
- Nonomura, I. & Imada, Y. (1982). Temperature-sensitive mutant of Mycoplasma synoviae. I. Production and selection of a nonpathogenic but immunogenic clone. Avian Diseases, 26, 763–775.
- Noormohammadi, A.H., Jones, J.F., Harrigan, K.E. & Whithear, K.G. (2003). Evaluation of the non-temperature-sensitive field clonal isolates of the Mycoplasma synoviae vaccine strain MS-H. Avian Diseases, 47, 355–360.
- Raviv, Z., Callison, S.A., Ferguson-Noel, N. & Kleven, S.H. (2008). Strain differentiating real-time PCR for Mycoplasma gallisepticum live vaccine evaluation studies. Veterinary Microbiology, 129, 179–187.
- Razin, S. & Jacobs, E. (1992). Mycoplasma adhesion. Journal of General Microbiology, 138, 407–422.
- Sawicka-Durkalec, A., Kursa, O., Bednarz, L. & Tomczyk, G. (2021). Occurrence of Mycoplasma spp. in wild birds: phylogenetic analysis and potential factors affecting distribution. Scientific Reports, 11, 17065.
- Sprygin, A., Volokhov, D.V., Irza, V.N. & Drygin, V. (2012). Detection and genetic identification of Mycoplasma sp.1220 in geese in the Russian Federation and Ukraine. Sel’skokhoz Yaistvennaya Biologiya, 2, 87–95.
- Stipkovits, L., Glavits, R., Ivanics, E. & Szabo, E. (1993). Additional data on Mycoplasma disease of goslings. Avian Pathology, 22, 171–176.
- Stipkovits, L. & Kempf, I. (1996). Mycoplasmoses in poultry. Revue Scientifique et Technique, 15, 1495–1525.
- Stipkovits, L., Varga, Z., Czifra, G. & Dobos-Kovacs, M. (1986). Occurrence of mycoplasmas in geese affected with inflammation of the cloaca and phallus. Avian Pathology, 15, 289–299.
- Stipkovits, L., Varga, Z., Dobos-Kovács, M. & Santha, M. (1984). Biochemical and serological examination of some Mycoplasma strains of goose origin. Acta Veterinaria Hungarica, 32, 117–125.
- Stülke, J. & Hillen, W. (1999). Carbon catabolite repression in bacteria. Current Opinion in Microbiology, 2, 195–201.
- Tatusova, T., DiCuccio, M., Badretdin, A., Chetvernin, V., Nawrocki, E.P., Zaslavsky, L., Lomsadze, A., Pruitt, K.D., Borodovsky, M. & Ostell, J. (2016). NCBI prokaryotic genome annotation pipeline. Nucleic Acids Research, 44, 6614–6624.
- Terato, K., Do, C., Chang, J. & Waritani, T. (2016). Preventing further misuse of the ELISA technique and misinterpretation of serological antibody assay data. Vaccine, 34, 4643–4644.
- Trappetti, C., McAllister, L.J., Chen, A., Wang, H., Paton, A.W., Oggioni, M.R., McDevitt, C.A. & Paton, J.C. (2017). Autoinducer 2 signaling via the phosphotransferase fruA drives galactose utilization by Steptococcus pneumoniae, resulting in hypervirulence. mBio, 8, e02269.
- Varga, Z., Stipkovits, L., Dobos-Kovács, M. & Sántha, M. (1986). Investigation of goose mycoplasmas. Archiv für Experimentelle Veterinärmedizin, 40, 105–108.
- Volokhov, D.V., Grózner, D., Gyuranecz, M., Ferguson-Noel, N., Gao, Y., Bradbury, J.M., Whittaker, P., Chizhikov, V.E., Szathmary, S. & Stipkovits, L. (2020). Mycoplasma anserisalpingitidis sp. nov., isolated from European domestic geese (Anser anser domesticus) with reproductive pathology. International Journal of Systematic and Evolutionary Microbiology, 70, 2369–2381.
- Waritani, T., Chang, J., McKinney, B. & Terato, K. (2017). An ELISA protocol to improve the accuracy and reliability of serological antibody assays. MethodsX, 4, 153–165.
- Whithear, K.G. (1996). Control of avian mycoplasmoses by vaccination. Revue Scientifique et Technique, 15, 1527–1553.
- Whithear, K.G., Soeripto & Ghiocas, E. (1990a). Efficacy of the ts-11 attenuated Mycoplasma gallisepticum vaccine strain. IOM Letters, 1, 361–362.
- Whithear, K.G., Soeripto, Harrigan, K.E. & Ghiocas, E. (1990b). Immunogenicity of a temperature sensitive mutant Mycoplasma gallisepticum vaccine. Australian Veterinary Journal, 67, 168–174.
- Whithear, K.G., Soeripto, Harringan, K.E. & Ghiocas, E. (1990c). Safety of temperature sensitive mutant Mycoplasma gallisepticum vaccine. Australian Veterinary Journal, 67, 159–165.
- Wilkening, R.V., Chang, J.C. & Federle, M.J. (2016). Pepo, a CovRS-controlled endopeptidase, disrupts Streptococcus pyogenes quorum sensing. Molecular Microbiology, 99, 71–87.
- Yamamoto, R. & Adler, H.E. (1958). Characterization of pleuropneumonia-like organisms of avian origin. Antigenic analysis of seven strains and their comparative pathogenicity for birds. The Journal of Infectious Diseases, 102, 143–152.