ABSTRACT
Highly pathogenic (HP) avian influenza viruses (AIVs) of the clade 2.3.4.4 goose/Guangdong/1996 H5 lineage continue to be a problem in poultry and wild birds in much of the world. The recent incursion of a H5N1 clade 2.3.4.4b HP AIV from this lineage into North America has resulted in widespread outbreaks in poultry and consistent detections of the virus across diverse families of birds and occasionally mammals. To characterize the pathobiology of this virus in mallards (Anas platyrhynchos), which are a primary reservoir of AIV, a challenge study was conducted with 2-week-old birds. The 50% bird infectious dose was determined to be < 2 log10 50% egg infectious doses (EID50) and all exposed ducks, including ducks co-housed with inoculated ducks, were infected. Infection appeared to be subclinical for 58.8% (20/34) of the ducks, one duck was lethargic, about 20% developed neurological signs and were euthanized, and 18% developed corneal opacity. The mallards shed virus by both the oral and cloacal routes within 24–48 h post-infection. Oral shedding substantially decreased by 6–7 days post-infection, but 65% of the ducks continued to shed virus cloacally through 14 days post-exposure (DPE) for the direct inoculates and 13 DPE for contact-exposed ducks. Based on the high transmissibility, high virus shed titres, and mild-to-moderate disease, mallards could serve as efficient reservoirs to amplify and disseminate recent North American clade 2.3.4.4b viruses.
Introduction
Dabbling ducks (Anatidae family, Anatinae subfamily), which includes mallards (Anas platyrhynchos), are natural hosts for avian influenza virus (AIV) and it is not uncommon for them to carry low pathogenic (LP) AIV (Stallknecht & Brown, Citation2017). Among dabbling ducks, mallards are highly sampled and consistently have high AIV prevalence compared to other waterfowl (Olsen et al., Citation2006; Lauterbach et al., Citation2020). Since the mid-2000s the goose/Guangdong/1996 (gs/GD/96) H5 highly pathogenic (HP) AIV lineage has become endemic in wild waterfowl in Asia, Africa, and Europe (Lee et al., Citation2015; Kuiken, Citation2016; Lee et al., Citation2018). The current predominant gs/GD/96 H5 sub-lineage, clade 2.3.4.4b was introduced into North American wild waterfowl through bird migration in 2014 (Lee, et al., Citation2015) and 2021 (Bevins et al., Citation2022). It has been recognized for decades that AIV in wild waterfowl can be transmitted to poultry (Johnson et al., Citation1977; Deibel et al., Citation1985; Halvorson et al., Citation1985). Since its emergence in 2014 (Lee et al., Citation2014), the number of HPAIV outbreaks of the clade 2.3.4.4 lineage has substantially increased in poultry worldwide and has further evolved into subclades (WOAH, Citation2022a). The most prevalent subclade worldwide, which is also the subclade in the U.S, is 2.3.4.4b. Spread of clade 2.3.4.4b in North America is currently facilitated by a lack of immunity against this specific virus in the birds, based on lack of cross-reactivity between North American H5 AIVs and the clade 2.3.4.4b viruses (unpublished data).
Clade 2.3.4.4b H5 HPAIVs have not only been detected in wild waterfowl and poultry, but also in samples from sick and dead members of diverse families and orders of wild bird species not normally infected with AIV, and in mammals, often of predatory and scavenger species (FAO-UN, Citation2022). Because of this, infection and dissemination of this sub-lineage are a concern for domestic and wild bird health (Ramey et al., Citation2022).
In contrast to the severe disease seen in many bird species, mallards have not seemed to have been affected as severely and most detections have been from hunter-harvested birds. This may be due to immunity from prior infection with other AIV strains (Fereidouni et al., Citation2009; Costa et al., Citation2010a; Koethe et al., Citation2020) and/or, similar to their response to infection with other HPAIV strains, they may naturally be less susceptible to disease (Brown et al., Citation2006; Brochet et al., Citation2009; Pantin-Jackwood et al., Citation2016; Ducatez et al., Citation2017; Pantin-Jackwood, Costa-Hurtado, et al., Citation2017; van den Brand et al., Citation2018; Tanikawa et al., Citation2022).
As clade 2.3.4.4 H5 HPAIV continues to circulate in wild birds and to evolve, characterization is necessary to understand its ecology and potential to transmit and cause disease among wild and domestic bird species. Therefore, the objective of this study was to characterize the pathobiology and transmissibility of a recent North American clade 2.3.4.4b isolate, A/American Wigeon/SC/22-000345-001/2022 H5N1 HPAIV, in mallards using a similar study design to previous reports evaluating gs/GD/96 H5 HP AIV in mallards and Pekin ducks (Anas platyrhynchos) (DeJesus et al., Citation2016; Leyson et al., Citation2019; Pantin-Jackwood, et al., Citation2017).
Materials and methods
Virus
A clade 2.3.4.4b H5N1 HPAIV, A/American Wigeon/South Carolina/22-000345-001/2022 (AMWI/SC/22) (provided by the National Veterinary Services Laboratory, USDA-APHIS Ames, IA, USA) was used in this study. The virus was propagated by inoculation of 9–11-day-old embryonating chicken eggs by the chorio-allantoic route using standard methods (Spackman & Killian, Citation2020). Allantoic fluid was harvested, clarified by centrifugation, and stored at −80°C. The Reed and Muench method (Reed & Muench, Citation1938) was used to determine the virus titre in 9–11-day-old embryonating chicken eggs using established methods (Spackman & Killian, Citation2020). All procedures involving virus were performed in a biosafety level-3 enhanced facility in accordance with procedures approved by the US National Poultry Research Center (USNPRC) Institutional Biosafety Committee.
Pathogenesis and transmission study
All procedures involving birds were reviewed and approved by the USNPRC Institutional Animal Care and Use Committee. Mallard ducks were obtained 24 h post-hatch from a commercial mail-order hobby bird hatchery and were housed in isolation until the challenge was administered. The ducks came from flocks that were monitored for AIV infection. The experimental design was the same as in previous studies (Pantin-Jackwood, et al., Citation2017; Pantin-Jackwood, Stephens, et al., Citation2017; Spackman et al., Citation2016) to facilitate comparisons among species and isolates. Just before inoculation, blood was collected from 10 of the 44 ducks to screen for antibodies to influenza A and the challenge virus using both the haemagglutination inhibition (HI) assay as described below and a commercial type A influenza antibody specific blocking ELISA (MultisScreen AI Antibody IDEXX Inc., Westbrook, ME, USA). The ELISA was run in accordance with the manufacturer’s instructions.
At 2 weeks of age, mallards exposed by direct inoculation were challenged with AMWI/SC/22 with doses of approximately 2 (low), 4 (mid), or 6 (high) log10 EID50 per bird in 0.1 ml by the intra-choanal (ICH) route as described in . Titration of the inoculum, as described above, confirmed the doses to be 1.83, 3.83, and 5.75 log10 EID50 for the low-, mid-, and high-dose groups respectively. Ten hatch-mates served as non-inoculated controls.
Table 1. Treatment group descriptions.
Oropharyngeal (OP) and cloacal (CL) swabs were collected in 2 ml brain heart infusion broth from all ducks at 2, 4, 7, 10, and 14 days post-challenge (DPC) for the inoculated ducks, and 1, 3, 6, 9, 13 days post-exposure (DPE) for the contact-exposed ducks. Birds that showed severe clinical signs were euthanized. At 2 and 4 DPC, two ducks inoculated with the high dose which had clinical signs, and one control duck were euthanized and necropsied. One set of tissues was collected in 10% neutral buffered formalin to evaluate microscopic lesions and to visualize influenza virus antigen in tissues by immunohistochemistry (IHC) staining (Pantin-Jackwood, Citation2020): nasal turbinates, trachea, lung, eyelid, heart, brain, proventriculus, duodenum, caecal tonsils, pancreas, liver, kidney, adrenal gland, spleen, thymus, cloacal bursa, skeletal muscle, eye, and gonads. A second set of tissues was collected from each duck for virus detection and quantification by quantitative real-time reverse transcription PCR (qRT-PCR): lung, spleen, heart, muscle, and brain. Identical tissue sets were collected from the two non-inoculated hatch-mates to serve as negative controls.
Mean virus shed titres were compared between: (1) ducks showing any clinical signs (neurological, corneal opacity, or lethargy) and ducks that appeared healthy; and (2) between ducks with neurological signs and apparently healthy ducks. Oral and CL shed titres were compared by swab type (OP or CL) and DPC (2 or 4) using the Mann–Whitney test (Prism 9.4.1 Graph Pad Software, Boston, MA, USA). Other timepoints and the contact exposure birds could not be included because there were too few birds for meaningful statistical analysis.
Infectious dose calculations
The 50% bird infectious dose was calculated with the Reed-Muench method (Reed & Muench, Citation1938). A duck was considered infected if it was either positive for virus shed at any time or had detectable antibodies at 14 DPC.
RNA extraction
Briefly, total RNA was isolated from OP and CL swab material with the MagMAX-96 AI/ND Viral RNA Isolation Kit (Thermo Fisher; Waltham, MA, USA) (Das et al., Citation2009). Tissues were cut into approximately 1 g portions and homogenized in 1 ml brain heart infusion (BHI). Total RNA was extracted from supernatants using Trizol LS reagent (Invitrogen/Thermo Fisher Scientific; Grand Island, NY, USA) in accordance with the manufacturer’s instructions. RNA was resuspended in 50 µl of nuclease-free water.
Quantitative real-time reverse transcription PCR to determine virus titre equivalents
Virus titre equivalents in swabs were determined by qRT-PCR as described previously (Spackman et al., Citation2002).
A type A influenza-specific one-step real-time qRT-PCR assay targeting the influenza A matrix gene (Spackman, et al., Citation2002) was run with a standard curve to calculate virus titre equivalents. The standard curve was generated from a 10-fold dilution series of the same titrated AW/SC/22 stock used as the challenge virus and was run in duplicate.
Serology
Serology was used to help confirm whether individual ducks were infected. Serum from ducks was tested prior to challenge (n = 10) and from all surviving ducks 14 DPC. Haemagglutination inhibition (HI) assay was used to quantify antibody to the challenge virus using established methods (Spackman & Sitaras, Citation2020). Endpoint titres are reported as the log2 dilution of the last HI-positive serum dilution. A titre of 16 or above was considered positive.
Results
Clinical disease
No differences in signs were observed between dose groups or exposure route (direct inoculation or contact exposure). However, presentation of signs generally started about 2 days later in the lowest dose group and the contact-exposed ducks compared to the mid- and high-dose groups and ICH-inoculated ducks (). A total of 14 ducks (41.2%) presented with clinical signs. One duck (2.9%) from the mid-dose directly inoculated group was visibly lethargic and had corneal opacity (CO). Neurological signs were observed in seven (20.5% of infected) ducks, which were euthanized when the sign was first observed (Supplementary Table 1). One contact transmission duck in the lowest dose group, which presented no clear clinical signs, died 7 DPE. Six ducks (three inoculated and three contact-exposed) developed CO (17.6% of infected ducks including the lethargic duck) and were first observed between 4 and 10 DPC/DPE in individual birds. CO was unilateral in five of the six affected ducks.
Table 2. Number of mallards inoculated with A/AMWI/SC/345/22 H5N1 HPAIV shedding virus and mean titre equivalents based on real-time RT-PCR by dose and day post-challenge.
Gross and microscopic lesions
At 2 and 4 DPC ducks with neurological signs were selected for necropsy (two ducks each day). Two non-inoculated control ducks were necropsied at 4 DPC. Gross lesions in all infected ducks necropsied at either 2 or 4 DPC included empty intestinal tract (i.e. the ducks were anorexic). Both ducks necropsied at 2 DPC had pale livers and one of those ducks had a friable brain. Of the ducks necropsied at 4 DPC, one had a small thymus and pale pancreas. The other duck necropsied at 4 DPC had an enlarged heart, petechia in the thigh muscle, prominent vasculature in the brain, and a pale pancreas, thymus, and spleen. No gross lesions were observed in the non-inoculated ducks except for a pale thymus in one of the two ducks.
The microscopic lesions and distribution of viral antigen staining (an indication of virus replication) were similar between ducks euthanized and examined at 2 and 4 DPC; however, the lesions were more severe and the antigen staining stronger and more widespread within tissues in the ducks examined at 4 DPC. Microscopic lesions included moderate to severe necrotizing rhinitis and sinusitis, mild-to-moderate tracheitis and bronchitis with lumen cellular debris, and mild-to-moderate interstitial pneumonia. In the brain, randomly scattered foci of malacia with gliosis were observed. Focally extensive neuronal degeneration and necrosis and vacuolation of the neuropil were present in the ducks examined at 4 DPC. Mild multifocal cellular swelling and necrosis of the pancreatic acinar epithelium occurred in ducks sampled at 4 DPC. In one of the 4 DPC ducks, the corticotrophic cells of the adrenal gland had mild focal vacuolar degeneration. Minimal-to-moderate necrosis of hepatocytes was observed in the liver. Skeletal muscle and heart had degeneration to necrosis of individual myofibres. Mild to moderate lymphoid depletion was observed in all lymphoid organs.
Viral antigen staining was present in multiple tissues indicating systemic infection (, ) and patterns of viral staining were similar to those previously reported (DeJesus et al., Citation2016). No viral staining was found in the proventriculus, duodenum, caecal tonsils, kidney, and gonads in any of the ducks examined. Viral antigen was present in epithelial cells and macrophages in the nasal turbinates, trachea, salivary glands, and lung; neurones and glial cells of the brain; cardiac and skeletal myofibres; Kupffer cells in the liver; pancreatic acinar epithelium; epithelial cells and feather pulp in eyelid skin; iris muscle cells and in the pigmented and nonpigmented epithelial cells of the ciliary process in the eye; corticotrophic cells and infiltrating mononuclear cells in the adrenal gland; epithelial cells in the cloacal bursa; and in resident and infiltrating phagocytes of the thymus, bursa, and spleen.
Figure 1. Titre equivalents of A/American wigeon/SC/000345-01/2022 H5 HPAIV in oral or cloacal swabs by dose group, exposure route and day post-challenge or exposure as determined by quantitative real-time RT-PCR. Thick horizontal bars denote means, thin bars denote standard deviation. The horizontal dotted line is the assay limit of detection. Samples where no virus was detected were given a value of 50% of the assay limit of detection.
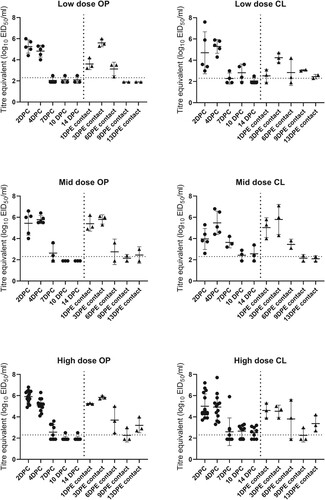
Table 3. Distribution of AIV antigen in tissues collected from mallards experimentally infected with A/American Wigeon/South Carolina/000345-001/2022 H5N1 HPAIV. Tissues were collected at 2 and 4 days post-challenge (DPC). The scoring is expressed in the form of bird 1/bird 2 for each DPC. No viral staining was found in the proventriculus, duodenum, caecal tonsils, kidney, and gonads.
Virus titres in tissues
Quantities of virus in tissues were determined by qRT-PCR for two inoculated ducks at 2 and 4 DPC each and two control ducks at 4 DPC (). No virus was detected in tissues from the control ducks. The spleen and thigh muscles were positive from all four inoculated ducks, but virus was not detected in the lung of one duck at 2 DPC and was also not detected in the heart and brain of one duck from each DPC. The titres were highest in the brain (7.7 and 9.3log10 EID50/ml equivalents).
Table 4. Titre equivalents (50% egg infectious dose [EID50]) by rRT-PCR of virus in tissues from ducks inoculated with A/American Wigeon/South Carolina/22-000345-001/2022 2 and 4 days post-challenge (DPC).
Oral and cloacal virus shed
Oropharyngeal and CL swabs were collected from all ducks at 2, 4, 7, 10, and 14 DPC (1, 3, 6, 9, and 13 days post-exposure [DPE] for the contact-exposed ducks) (). Virus was not detected in swabs from control ducks at any time. Regardless of dose or route of exposure, all ducks shed virus in similar patterns. Detectable levels of virus were shed by 100% (34/34) of ducks by the oral route, and 33/34 (97.1%) by the cloacal route by 1-2 DPC. Oral shedding decreased substantially at 10 DPC/9 DPE (17%) and 14 DPC/13 DPE (26%). In contrast, the fewest ducks that were shedding virus by the cloacal route was 63% at 7 DPC/6 DPE; at 14 DPC/13 DPE 65% of the ducks were still shedding virus, although the mean titres were substantially lower than at earlier time points. At 2 DPC, inoculated birds shed more virus orally than cloacally, and at 10 and 14 DPC they shed more cloacally. Quantities of virus shed by ducks with any clinical signs were not significantly different than those shed by apparently healthy ducks (Online Supplementary Figure 1). Similarly, virus shed quantities were not significantly different between the ducks with neurological signs and apparently healthy ducks.
Figure 2. Immunohistochemical staining for AIV antigen in tissues of mallards infected with the A/American Wigeon/SC/000345-01/2022 H5N1 HPAI virus. Virus antigens are stained red. A, B, and C: tissues from ducks at 2 DPC. D-I: tissues collected from ducks at 4 DPC. A. Cerebellum. B. Lung. C. Nasal epithelium. D. Cerebrum. E. Eye ciliary process. F. Feather follicles. G. Thymus. H. Heart. I. Skeletal muscle. Magnification 20×.
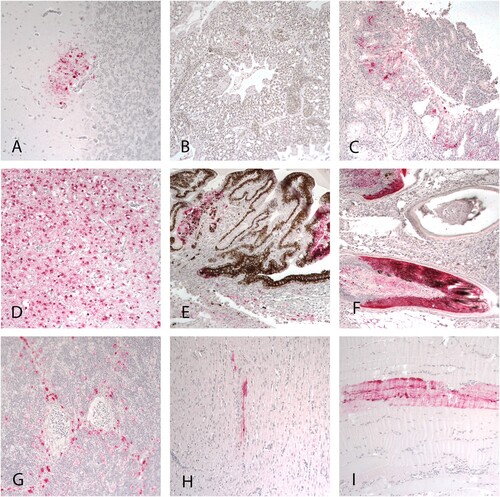
Transmission and infectious dose
Antibodies to neither influenza A nor the challenge virus were detected in serum collected prior to challenge (Online Supplementary Table 2). Antibodies to AIV were not detected in serum from non-inoculated control ducks at the termination of the experiment. Antibodies were detected in serum from all surviving ducks at 14 DPC or 13 DPE (contact-exposure birds) by HI assay (Online Supplementary Table 2). In addition, 100% of ducks shed virus after inoculation or contact exposure. Therefore, 100% of the ducks were infected regardless of exposure route. The lower endpoint for the infectious dose was not reached and the 50% bird infectious dose (BID50) was < 2 log10 EID50 per bird.
Discussion
A recent North American isolate of HPAIV belonging to clade 2.3.4.4b of the gs/GD/96 lineage (AMWI/SC/22) was evaluated for its pathogenesis and transmissibility in mallards. Numerous reports of natural infections and previous studies have reported on the pathogenesis of earlier sublineages of clade 2.3.4.4 in mallards and closely related species such as Pekin ducks (Anas platyrhynchos domesticus) and American black ducks (Anas rubripes). These studies showed variability in disease severity among isolates. Experimental infections of mallards and Pekin ducks with clade 2.3.4.4 isolates from 2014 resulted in relatively mild clinical disease (DeJesus, et al., Citation2016; Spackman et al., Citation2019). In contrast, viruses from 2016 caused severe disease and mortality (Leyson, et al., Citation2019; Usui et al., Citation2020). In naturally-infected domestic waterfowl, mostly Pekin ducks, clinical disease caused by the current clade 2.3.4.4b HPAIV lineage in the US is generally mild, but drops in egg production, and feed and water consumption may occur. (WOAH, Citation2022b).
The specific clinical signs, gross lesions, and microscopic lesions observed here are consistent with what has been reported previously with gs/GD/96 lineage H5 HPAIVs in mallards and related duck species. Neurological signs (i.e. ataxia, torticollis) are common in gs/GD/96 H5 HPAIV infections in ducks and are caused by virus replication in the brain (Pantin-Jackwood, et al., Citation2016; Usui, et al., Citation2020; Tanikawa, et al., Citation2022). Corneal opacity during HPAIV infection in ducks has been thoroughly described (Yamamoto et al., Citation2016) and is caused by an inflammatory response to virus replicating in the cornea. Virus replication was observed in eye tissues of 1 of the ducks examined for microscopic lesions.
About 41% of the ducks presented with clinical signs during the study (because all ducks, inoculated and contacts, were infected, discussion of the clinical outcome will treat them as one group). Neurological signs and corneal opacity were the primary clinical signs observed with the exception of one duck that was both lethargic and had CO, and one duck died without showing any clear clinical signs. Because the ducks with neurological signs were euthanized for humane reasons, it is unclear whether they would have developed additional signs. Both CO and neurological impairment would likely impact survival in the wild, even if these birds were to recover from the virus infection. In the wild, lethargy and CO would likely be missed by observers unless the ducks were observed at a close distance, therefore they may be underreported. However, there is some uncertainty about how the severity of clinical disease observed here would translate to mallards in the wild.
The severity of disease caused by HPAIV in ducks is affected by age. Younger birds, such as these, would likely present with more severe disease than older birds (Pantin-Jackwood et al., Citation2007) and 2-week-old ducks, the age of exposure here, would only be exposed in breeding grounds. In addition, these ducks were naïve to influenza A. In the wild, prior infection with AIV, even other subtypes, might provide some immunity which would mitigate disease (Fereidouni, et al., Citation2009; Costa, et al., Citation2010a; Segovia et al., Citation2017; Koethe, et al., Citation2020). Regardless of age, most of the ducks did not present with disease, and effects on survival and movement could be short-term and minimal. Finally, another unknown is the impact of other physiological stressors, e.g. other diseases, nutrition, migration, etc., on disease severity and progression.
Similar to these previous studies, the mallards were highly susceptible to infection with the virus; all dose groups and all contact-exposed ducks became infected and were shedding virus 24 h post-exposure. The lower endpoint for the virus was not achieved so the BID50 was < 2log10 EID50. The BID50 for clade 2.3.4.4 isolates have consistently been reported to be < 3log10 EID50 in mallards, and the closely related Pekin and American black ducks (Pantin-Jackwood, et al., 2017; Spackman, et al., Citation2019).
Understanding virus shed patterns from mallards is important for virus transmission and informing sampling strategies for wild and domestic ducks. Here, 100% of the mallards shed virus and the mean quantities at the peak of shed 1-4 DPC were high both orally (mean 5.4log10 EID50/ml) and cloacally (mean 4.8log10 EID50/m1). Oral and cloacal shedding were highest (highest quantities and highest proportion of ducks shedding) from 1 through 4 DPC regardless of exposure route. Oral shed decreased rapidly by 7 DPC; however, 65% of the ducks continued to shed cloacally through 13/14 DPC.
The high quantities of virus shed orally and cloacally, and long duration of cloacal shed observed here, suggest that this isolate would spread by infected ducks efficiently contributing to environmental contamination. Notably, the quantities of virus shed cloacally were generally higher than those observed with the 2014 clade 2.3.4.4 isolates from the US (A/NOPI/WA/40964/2014 and A/gyrfalcon/40188/2014) (DeJesus, et al., Citation2016), which suggests the AMWI/SC/22 may spread more easily. Further, it has been reported that the quantity of LPAIV shed by mallards increases with age from 2 weeks to 4 months (Costa et al., Citation2010b). If there is a similar pattern with HPAIV, older ducks may contribute to transmission more than younger ducks by contributing more to shed. Practically, because titres were high early after infection and shed persisted longer cloacally, cloacal swabs remain a good sample type for mallards and probably should still be used for Pekin ducks and wild dabbling ducks.
Results here are consistent with previous studies using the same experimental design with gs/GD/96 lineage isolates in mallards. However, there is some variability in clinical outcome. Two clade 2.3.4.4 H5N2 HP AIV isolates from gallinaceous poultry from the 2015 outbreak in the US were examined in mallards and compared to the original US 2014 index H5N2, A/northern pintail/40964/2014 and A/gyrfalcon/WA/41088/2014 H5N8 HP AIV and a clade 2.2 GS/GD H5 HP AIV A/whooper Swan/Mongolia/244/2005 (DeJesus, et al., Citation2016). All five viruses had similar high infectivity for mallards (infectious doses of less than 3log10 and transmitted to co-housed naïve mallards). Low mortality occurred with the clade 2.2 H5N1 isolate A/whooper Swan/Mongolia/244/2005 (100%) and with A/turkey/MN/12582/2015 (7.5%). Shed profiles by both the OP and CL routes varied among all five isolates, the shed profile with A/AMWI/SC/2022 was most similar to the turkey-origin isolate that caused low mortality (DeJesus, et al., Citation2016). In contrast, high mortality (79.1%) was observed in mallards inoculated with A/tufted duck/Denmark/11740_LWPL/2016, a gs/GD/96 H5N8 clade 2.3.4.4b HP AIV (Leyson, et al., Citation2019). That virus had an infectious dose of 3log10 and transmitted to ducks in the two highest dose groups (Leyson, et al., Citation2019).
In closely related Pekin ducks exposed to A/northern pintail/40964/2014 H5N2 and A/gyrfalcon/WA/41088/2014 H5N8, the infectious dose was also around 3log10 but did not transmit well to contacts (Pantin-Jackwood, et al., Citation2017). Virus titres shed through the OP route were higher than the CL route which may account for less efficient transmission (Pantin-Jackwood, et al., Citation2017), which is also in contrast to what was observed here. No mortality was observed with the Pekin ducks (Pantin-Jackwood, et al., Citation2017).
The clinical outcome of mallards being infected with clade 2.3.4.4 HPAIVs has implications for both wild birds and poultry. During 2022 through 28 October, 11 domestic duck flocks had been infected in the US. If ducks are easily infected and don’t experience clinical disease but shed high quantities of virus for a long duration, they are likely to be productive virus spreaders. As clade 2.3.4.4 H5 HPAIV continues to circulate in wild ducks, it will continue to evolve. This current variant in North America seems to have adapted to the niche at least in mallards, where it is highly infectious, causes subclinical disease in most birds, and is shed in high quantities.
Supplemental Material
Download MS Word (190 KB)Acknowledgements
We gratefully acknowledge Linda Moon, Anne Hurley-Bacon, Emily Newton, Tarrah Bigler, and Kaitlin Dozier for technical assistance.
Mention of trade names or commercial products does not imply recommendation or endorsement by the US Department of Agriculture. Any use of trade, firm, or product names is for descriptive purposes only and does not imply endorsement by the US Government. All opinions expressed in this paper are the authors’ and do not necessarily reflect the policies and views of US Department of Agriculture, Agriculture Research Service. The US Department of Agriculture is an equal opportunity provider and employer.
Disclosure statement
No potential conflict of interest was reported by the authors.
Additional information
Funding
References
- Bevins, S.N., Shriner, S.A., Cumbee, J.C., Dilione, K.E., Douglass, K.E., Ellis, J.W., Killian, M.L., Torchetti, M.K. & Lenoch, J.B. (2022). Intercontinental movement of highly pathogenic avian influenza A(H5N1) clade 2.3.4.4 virus to the United States, 2021. Emerging Infectious Diseases, 28, 1006–1011.
- Brochet, A.L., Guillemain, M., Lebarbenchon, C., Simon, G., Fritz, H., Green, A.J., Renaud, F., Thomas, F. & Gauthier-Clerc, M. (2009). The potential distance of highly pathogenic avian influenza virus dispersal by mallard, common teal and Eurasian pochard. Ecohealth, 6, 449–457.
- Brown, J.D., Stallknecht, D.E., Beck, J.R., Suarez, D.L. & Swayne, D.E. (2006). Susceptibility of North American ducks and gulls to H5N1 highly pathogenic avian influenza viruses. Emerging Infectious Diseases, 12, 1663–1670.
- Cohen, A.C. (1959). Simplified estimators for the normal distribution when samples are singly censored or truncated. Technometrics, 1, 217–237.
- Costa, T.P., Brown, J.D., Howerth, E.W. & Stallknecht, D.E. (2010a). Effect of a prior exposure to a low pathogenic avian influenza virus in the outcome of a heterosubtypic low pathogenic avian influenza infection in mallards (Anas platyrhynchos). Avian Diseases, 54, 1286–1291.
- Costa, T.P., Brown, J.D., Howerth, E.W. & Stallknecht, D.E. (2010b). The effect of age on avian influenza viral shedding in mallards (Anas platyrhynchos). Avian Diseases, 54, 581–585.
- Das, A., Spackman, E., Pantin-Jackwood, M.J. & Suarez, D.L. (2009). Removal of real-time reverse transcription polymerase chain reaction (RT-PCR) inhibitors associated with cloacal swab samples and tissues for improved diagnosis of avian influenza virus by RT-PCR. Journal of Veterinary Diagnostic Investigation, 21, 771–778.
- Deibel, R., Emord, D.E., Dukelow, W., Hinshaw, V.S. & Wood, J.M. (1985). Influenza viruses and paramyxoviruses in ducks in the Atlantic flyway, 1977-1983, including an H5N2 isolate related to the virulent chicken virus. Avian Diseases, 29, 970–985.
- DeJesus, E., Costa-Hurtado, M., Smith, D., Lee, D.H., Spackman, E., Kapczynski, D.R., Torchetti, M.K., Killian, M.L., Suarez, D.L., Swayne, D.E. & Pantin-Jackwood., M.J. (2016). Changes in adaptation of H5N2 highly pathogenic avian influenza H5 clade 2.3.4.4 viruses in chickens and mallards. Virology, 499, 52–64.
- Ducatez, M., Sonnberg, S., Crumpton, J.C., Rubrum, A., Phommachanh, P., Douangngeun, B., Peiris, M., Guan, Y., Webster, R. & Webby, R. (2017). Highly pathogenic avian influenza H5N1 clade 2.3.2.1 and clade 2.3.4 viruses do not induce a clade-specific phenotype in mallard ducks. Journal of General Virology, 98, 1232–1244.
- FAO-UN. (2022, Aug. 24, 2022). Global Avian Influenza Viruses with Zoonotic Potential situation update Retrieved Sept. 22, 2022, 2022, from https://www.fao.org/animal-health/situation-updates/.
- Fereidouni, S.R., Starick, E., Beer, M., Wilking, H., Kalthoff, D., Grund, C., Häuslaigner, R., Breithaupt, A., Lange, E. & Harder, T.C. (2009). Highly pathogenic avian influenza virus infection of mallards with homo- and heterosubtypic immunity induced by low pathogenic avian influenza viruses. PLoS One, 4, e6706.
- Halvorson, D.A., Kelleher, C.J. & Senne, D.A. (1985). Epizootiology of avian influenza: effect of season on incidence in sentinel ducks and domestic turkeys in Minnesota. Applied and Environmental Microbiology, 49, 914–919.
- Johnson, D.C., Maxfield, B.G. & Moulthrop, J.I. (1977). Epidemiologic studies of the 1975 avian influenza outbreak in chickens in Alabama. Avian Diseases, 21, 167–177.
- Koethe, S., Ulrich, L., Ulrich, R., Amler, S., Graaf, A., Harder, T.C., Grund, C., Mettenleiter, T.C., Conraths, F.J., Beer, M. & Globig, A. (2020). Modulation of lethal HPAIV H5N8 clade 2.3.4.4B infection in AIV pre-exposed mallards. Emerging Microbes & Infections, 9, 180–193.
- Kuiken, T. (2016). Role for migratory wild birds in the global spread of avian influenza H5N8. Science, 354, 213–217.
- Lauterbach, S.E., McBride, D.S., Shirkey, B.T., Nolting, J.M. & Bowman, A.S. (2020). Yearround influenza A virus surveillance in mallards (Anas platyrhynchos) reveals genetic persistence during the undersampled spring season. Viruses, 12, 632.
- Lee, D.H., Torchetti, M.K., Hicks, J., Killian, M.L., Bahl, J., Pantin-Jackwood, M. & Swayne, D.E. (2018). Transmission dynamics of highly pathogenic avian influenza virus A (H5Nx) clade 2.3.4.4, North America, 2014-2015. Emerging Infectious Diseases, 24, 1840–1848.
- Lee, D.H., Torchetti, M.K., Winker, K., Ip, H.S., Song, C.S. & Swayne, D.E. (2015). Intercontinental spread of Asian-origin H5N8 to North America through Beringia by migratory birds. Journal of Virology, 89, 6521–6524.
- Lee, Y.J., Kang, H.M., Lee, E.K., Song, B.M., Jeong, J., Kwon, Y.K., Kim, H.R., Lee, K.J., Hong, M.S., Jang, I. & Choi, K.S. (2014). Novel reassortant influenza A (H5N8) viruses, South Korea, 2014. Emerging Infectious Diseases, 20, 1087–1089.
- Leyson, C., Youk, S.S., Smith, D., Dimitrov, K., Lee, D.H., Larsen, L.E., Swayne, D.E. & Pantin-Jackwood, M.J. (2019). Pathogenicity and genomic changes of a 2016 European H5N8 highly pathogenic avian influenza virus (clade 2.3.4.4) in experimentally infected mallards and chickens. Virology, 537, 172–185.
- Olsen, B., Munster, V.J., Wallensten, A., Waldenstrom, J., Osterhaus, A.D. & Fouchier, R.A. (2006). Global patterns of influenza A virus in wild birds. Science, 312, 384–388.
- Pantin-Jackwood, M.J., Costa-Hurtado, M., Bertran, K., DeJesus, E., Smith, D. & Swayne, D.E. (2017). Infectivity, transmission and pathogenicity of H5 highly pathogenic avian influenza clade 2.3.4.4 (H5N8 and H5N2) United States index viruses in Pekin ducks and Chinese geese. Veterinary Research, 48, 33.
- Pantin-Jackwood, M.J., Costa-Hurtado, M., Shepherd, E., DeJesus, E., Smith, D., Spackman, E., Kapczynski, D.R., Suarez, D.L., Stallknecht, D.E. & Swayne, D.E. (2016). Pathogenicity and transmission of H5 and H7 highly pathogenic avian influenza viruses in mallards. Journal of Virology, 90, 9967–9982.
- Pantin-Jackwood, M.J., Stephens, C.B., Bertran, K., Swayne, D.E. & Spackman, E. (2017). The pathogenesis of H7N8 low and highly pathogenic avian influenza viruses from the United States 2016 outbreak in chickens, turkeys and mallards. PLoS One, 12, e0177265.
- Pantin-Jackwood, M.J., Suarez, D.L., Spackman, E. & Swayne, D.E. (2007). Age at infection affects the pathogenicity of Asian highly pathogenic avian influenza H5N1 viruses in ducks. Virus Research, 130, 151–161.
- Pantin-Jackwood, M. J. (2020). Immunohistochemical Staining of Influenza Virus in Tissues. Methods Molecular Biology, 2123, 29–36.
- Ramey, A.M., Hill, N.J., DeLiberto, T.J., Gibbs, S.E.J., Camille Hopkins, M., Lang, A.S., Poulson, R.L., Prosser, D.J., Sleeman, J.M., Stallknecht, D.E. & Wan, X. (2022). Highly pathogenic avian influenza is an emerging disease threat to wild birds in North America. The Journal of Wildlife Management, 86, e22171.
- Reed, L.J. & Muench, H. (1938). A simple method for estimating fifty per cent endpoints. American Journal of Epidemiology, 27, 493–497.
- Segovia, K.M., Stallknecht, D.E., Kapczynski, D.R., Stabler, L., Berghaus, R.D., Fotjik, A., Latorre-Margalef, N. & França, M.S. (2017). Adaptive heterosubtypic immunity to low pathogenic avian influenza viruses in experimentally infected mallards. PLoS One, 12, e0170335.
- Spackman, E. & Killian, M.L. (2020). Avian influenza virus isolation, propagation, and titration in embryonated chicken eggs. Methods in Molecular Biology, 2123, 149–164.
- Spackman, E., Pantin-Jackwood, M.J., Kapczynski, D.R., Swayne, D.E. & Suarez, D.L. (2016). H5N2 highly pathogenic avian influenza viruses from the US 2014-2015 outbreak have an unusually long pre-clinical period in turkeys. BMC Veterinary Research, 12, 260.
- Spackman, E., Prosser, D.J., Pantin-Jackwood, M., Stephens, C.B. & Berlin, A.M. (2019). Clade 2.3.4.4 H5 North American highly pathogenic avian influenza viruses infect, but do not cause clinical signs in, American black ducks (Anas rubripes). Avian Diseases, 63, 366–370.
- Spackman, E., Senne, D.A., Myers, T.J., Bulaga, L.L., Garber, L.P., Perdue, M.L., Lohman, K., Daum, L.T. & Suarez, D.L. (2002). Development of a real-time reverse transcriptase PCR assay for type A influenza virus and the avian H5 and H7 hemagglutinin subtypes. Journal of Clinical Microbiology, 40, 3256–3260.
- Spackman, E. & Sitaras, I. (2020). Hemagglutination inhibition assay. Methods in Molecular Biology, 2123, 11–28.
- Stallknecht, D. & Brown, J. (2017). Wild bird infections and the ecology of avian infuenza viruses. In D. Swayne (Ed.), Animal Influenza 2nd edn (pp. 153–176). Ames, IA: Wiley-Blackwell.
- Tanikawa, T., Fujii, K., Sugie, Y., Tsunekuni, R., Nakayama, M. & Kobayashi, S. (2022). Comparative susceptibility of mallard (Anas platyrhynchos) to infection with high pathogenicity avian influenza virus strains (Gs/Gd lineage) isolated in Japan in 2004-2017. Veterinary Microbiology, 272, 109496.
- Usui, T., Soda, K., Sumi, K., Ozaki, H., Tomioka, Y., Ito, H., Murase, T., Kawamoto, T., Miura, M., Komatsu, M., Imanishi, T., Kurobe, M., Ito, T. & Yamaguchi, T. (2020). Outbreaks of highly pathogenic avian influenza in zoo birds caused by HA clade 2.3.4.4 H5N6 subtype viruses in Japan in winter 2016. Transboundary and Emerging Diseases, 67, 686–697.
- van den Brand, J.M.A., Verhagen, J.H., Veldhuis Kroeze, E.J.B., van de Bildt, M.W.G., Bodewes, R., Herfst, S., Richard, M., Lexmond, P., Bestebroer, T.M., Fouchier, R.A. & Kuiken, T. (2018). Wild ducks excrete highly pathogenic avian influenza virus H5N8 (2014-2015) without clinical or pathological evidence of disease. Emerging Microbes & infections, 7, 67.
- WOAH. (2022a, Sept. 22, 2022). Global animal disease information system. Retrieved Sept. 22, 2022, 2022, from https://empres-i.apps.fao.org/
- WOAH. (2022b, Oct. 25, 2022). World Animal Health Information System. Retrieved Oct. 31, 2022, 2022, from https://wahis.woah.org/#/home
- Yamamoto, Y., Nakamura, K., Yamada, M. & Mase, M. (2016). Corneal opacity in domestic ducks experimentally infected With H5N1 highly pathogenic avian influenza virus. Veterinary Pathology, 53, 65–76.