ABSTRACT
Budgerigar fledgling disease (BFD) is a contagious disease caused by avian polyomavirus (APV) in psittacine birds and causes high mortality rates. Here, eight APV-positive cases were confirmed from dead parrots or parrot tissue samples by polymerase chain reaction (PCR). Full-length genome sequencing showed high nucleotide identity (98.84–100%) between the APV strains. Phylogenetic analysis revealed that two genogroups were cocirculating in South Korea. The nucleotide sequences of five strains, collected from different parrot species, were identical; however, pathological lesions were observed in only two parrots, both aged 2 months. Pathology included necrotic spots in the liver, subcutaneous haemorrhage, hepatomegaly, ascites, intranuclear inclusion bodies, hepatocyte karyomegaly, hepatic necrosis, and bile duct proliferation. This suggests that the pathogenicity of APV might be host age-dependent regardless of the host species. This study improves our understanding of APV pathogenicity and provides a more detailed genetic characterization of APV strains.
RESEARCH HIGHLIGHTS
Eight APV strains were identified in South Korea from 2019 to 2021.
By phylogenetic analysis, South Korean APV strains were classified into two clades.
Introduction
Budgerigar fledgling disease (BFD), one of the most common viral diseases in psittacine birds, is caused by avian polyomavirus (APV). The disease causes abdominal distension, feather abnormalities, subcutaneous haemorrhage, and sudden death (Bernier et al., Citation1981; Bozeman et al., Citation1981; Padzil et al., Citation2017). The clinical signs are hepatitis, ascites, hydropericardium, and high mortality rates in fledglings. BFD may spread from parent to offspring (Magdalena et al., Citation2011), and infected birds may transfer the virus via contaminated faeces or feather dust (Altan et al., Citation2016). BFD has been detected in other birds, such as falcon, buzzard, chicken, and Gouldian finch, as well as in psittacine birds (Stoll et al., Citation1993; Johne & Muller, Citation1998; Heenemann et al., Citation2015). However, the degree of susceptibility to and severity of the disease seems to vary among avian species that are infected (Katoh et al., Citation2010).
APV belongs to the Gammapolyomavirus genus in the Polyomaviridae family (Moens et al., Citation2017). This virus has a nonenveloped icosahedral capsid with a diameter of 45–50 nm and contains a double-stranded circular DNA genome of approximately 5000 bp in length. The genome is divided into early and late regions and transcribed bidirectionally from the noncoding control region. The early regions encode open reading frames for two proteins: large and small tumour antigens. The late regions encode three structural proteins: VP1, VP2, and VP3 (Stoll et al., Citation1993). In addition, two proteins, VP4 and VP4 delta, are observed only in polyomavirus isolated from avian species (Johne et al., Citation2007; Padzil et al., Citation2017).
APV was first isolated from fledgling budgerigars in the USA (Bozeman et al., Citation1981; Davis et al., Citation1981) and Canada (Bernier et al., Citation1981) in 1981. Since then, it has been identified in Germany, Poland, Portugal, Turkey, Pakistan, New Zealand, Taiwan, Japan, and China (Stoll et al., Citation1993; Katoh et al., Citation2009; Dayaram et al., Citation2015; Henriques et al., Citation2018; Ma et al., Citation2019; Riaz et al., Citation2019; Adiguzel et al., Citation2020; Hu et al., Citation2022; Liu et al., Citation2022). The virus was first found in South Korea in an Alexandrine parakeet (Psittacula eupatria), in 2014 (Kim et al., Citation2014). A recent study reported the prevalence and genetic diversity of APV detected between 2016 and 2019 (Kim et al., Citation2022).
In this study, we performed full genome sequencing and genetic characterization of APV strains identified in South Korea between 2019 and 2021, and presented cases of dead parrots showing gross and histopathological changes caused by APV infection.
Materials and methods
Samples
Six dead parrots and two homogenized parrot samples (faeces and intestine), collected from 2019 to 2021, were confirmed as APV-positive based on PCR for the VP1 gene, as described previously (Phalen et al., Citation1991). Six bird carcasses were submitted to the Avian Disease Division of the Animal and Plant Quarantine Agency (APQA) for diagnosis, and the other samples (one faeces, one intestine) were obtained from Animal Disease Intervention Center, Kyungpook National University. Detailed information on parrots is listed in . At necropsy, organs of the dead birds, including the liver, intestine, and kidney, were obtained and homogenized in 10% (w/v) phosphate-buffered saline (PBS) containing 50 μg/ml gentamycin. The homogenates were centrifuged at 2095 × g for 10 min, and the supernatants were collected for total nucleic acid extraction. Additionally, a cloacal swab collected from A19-82 was resuspended in 1 ml PBS and stored at −80°C until use.
Table 1. Sources of the APV-positive samples analyzed in this study.
Histopathology
The samples collected were fixed in 10% (v/v) buffered formalin, and the tissues were embedded in paraffin. Sections of 4–5 μm were stained with haematoxylin and eosin and examined under the microscope.
DNA extraction and sequencing
Viral DNA was extracted from homogenates using a Viral Gene-Spin DNA/RNA Extraction Kit (Intron Biotechnology, Seongnam, South Korea) according to the manufacturer’s instructions. To analyze the complete genome of APV, PCR assays with varying annealing temperatures and cycle numbers were performed to optimize the PCR conditions with four sets of APV primers described in a previous study (Liu et al., Citation2022). PCR reactions were prepared in a total volume of 20 µl, each of which contained 4 µl of each viral DNA and 1 µl of each primer (10 pmoles/μl), using the Maxime™ PCR premix kit (i-StarTaq) (Intron Biotechnology). Amplified PCR fragments were analyzed under UV light after separation by 1.5% (w/v) agarose gel electrophoresis, and Sanger sequencing was carried out using the ABI 3730XL DNA analyzer at Bionics Co., Ltd. (Daejeon, South Korea).
Genetic analysis
The DNA sequences obtained were trimmed to remove the low-quality sequences and then assembled and aligned with APV reference sequences using the CLC Workbench version 20.0 (CLC bio, Aarhus, Denmark). The reference sequences were obtained from https://www.ncbi.nlm.nih.gov/genbank/ for genetic comparison. A phylogenetic tree was constructed by MEGA 11 (Tamura et al., Citation2021) using the neighbor-joining method with 1000 bootstrap replicates.
Nucleotide sequence accession numbers
Full-length sequences of eight newly identified APV strains have been submitted to the GenBank database under the accession numbers OQ230286-OQ230293.
Results
Gross lesions and histopathology
APV-positive samples were identified from eight different parrot species, including the African gray parrot, Black-headed parrot, Eclectus parrot, Senegal Parrot, Amazon parrot, and Quaker parrot/monk parakeet (). All APV-positive parrots were young birds aged between 2 and 7 months. Gross lesions were observed only in the birds infected with APV strains 20AD047 and 21AD001. The parrot infected with 20AD047 showed subcutaneous hemorrhage in the neck and abdomen, hepatomegaly, and pale-yellow necrotic spots. Ascites and yellow necrotic spots in the liver were observed in the parrot infected with 21AD001.
Microscopic examination of tissue from the parrot infected by 20AD047 revealed necrosis of hepatocytes scattered through the hepatic parenchyma ((a)) and bile duct proliferation around the portal vein ((b)). In addition, basophilic intranuclear inclusion bodies were observed in the liver ((c)). In the parrot infected with 21AD001, hepatocyte karyomegaly and basophilic intranuclear inclusion bodies were observed in the liver ((a)), and pale basophilic intranuclear inclusion bodies were detected in the tubular epithelium of the kidney ((b)). The six parrots infected with other strains were confirmed positive for APV by PCR, but no gross and histopathological lesions were detected in these birds.
Figure 1. Budgerigar fledgling disease in a 2-month-old Eclectus parrot (20AD047): histopathological lesion in the liver. (a) Hepatocellular necrosis (arrows) (×100). (b) Bile duct proliferation around the portal vein (arrows) (×200). (c) Basophilic intranuclear inclusion bodies (arrows) in hepatocytes (×400).
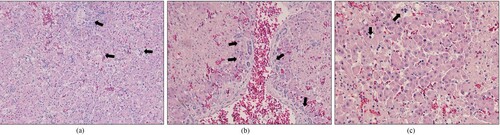
Figure 2. Budgerigar fledgling disease in a 2-month-old Amazon parrot (21AD001). (a) Histopathological lesions in the liver: hepatocyte karyomegaly (white arrows) and basophilic intranuclear inclusion bodies (black arrows) in hepatocytes (×400). (b) Histopathological lesions of the kidney: pale basophilic intranuclear inclusion bodies in the tubular epithelium (×400).
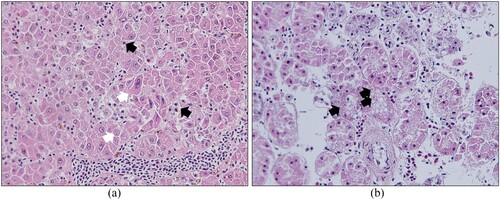
Phylogenetic tree
The sequences of the South Korean APV strains were compared with 33 APV sequences isolated from various regions and at different times (). A phylogenetic tree generated using the complete genome sequence of APV revealed that the eight South Korean strains were divided into two genogroups (I and II). Seven South Korean strains were clustered in genogroup I, together with strains from Europe, Asia, and the USA. 20AD031 belonged to genogroup II, along with strains identified in Japan, China, and South Korea. This showed that two APV genogroups were cocirculating in South Korea. Despite five of the seven South Korean strains in genogroup I clustereing on a single branch of the tree, only two of these stains caused pathological lesions in parrots.
Genetic analysis
The complete genomes of the eight South Korean strains were all 4981 bp in length. The nucleotide sequences of all 41 APV full genomes were very similar (98.84–100%) (). The genomic sequences of the five closely clustered Korean strains in genogroup I were 100% identical. The genome of strain 20AD031 was 99.56% similar to these five strains, whereas those of A19-48 and A19-82 showed 99.58% nucleotide identity. These differences represent a total of 22 nucleotides changes in strain 20AD031 and 21 nucleotide changes in strains A19-48 and A19-82.
Figure 4. Pairwise comparison analysis. Percent identity matrix generated from 41 APV complete genome sequences using CLC Workbench version 20.0.
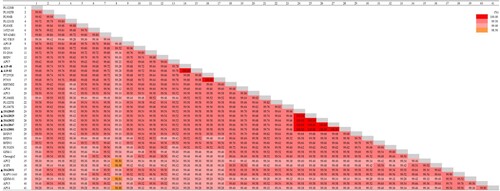
By performing multiple alignments, the amino acid sequences of the South Korean strains were compared with those of APV reference strains (). The amino acid sequences of APV strains were very similar. However, the APV strains from genogroup I and genogroup II harboured different amino acids in particular regions. The APV strains belonging to genogroup I encoded lysine at position 205 in viral protein 1 (VP1), arginine at position 234 in viral protein 2 (VP2), and leucine at position 221 in Large T Antigen (LT-Ag). By contrast, the APV strains of genogroup II encoded arginine at position 205 in VP1, histidine at position 234 in VP2, and proline at position 221 in LT-Ag. Compared with the other South Korean and reference stains, strain 20AD031 harboured S240 T and Q264E mutations in VP2. Compared with all of the other genogroup I and II strains, five of the seven South Korean strains in genogroup I (19AD045, 20AD029, 20AD032, 20AD047, and 21AD001) harboured amino acid substitutions in VP4 (Q94H), VP2 (K249R), and LT-Ag (A356S).
Table 2. Amino acid mutations in individual proteins of South Korean and reference APV strains.
Discussion
This study analyzed the complete genome sequences of APV strains collected between 2019 and 2021 in South Korea. The nucleotide identities of APV complete genome sequences ranged from 98.84% to 100%. This high level of genome conservation may be a result of slow evolution over time. According to phylogenetic analysis based on complete genome sequences, eight South Korean APV strains were classified into two genogroups, similar to those suggested in previous studies (Ma et al., Citation2019; Kim et al., Citation2022). Psittacine birds are not native to South Korea, but since imports of parrots have increased significantly since the 2000s (Kim et al., Citation2021), we speculate that these APV strains originated in birds imported through international trade.
Five APV strains clustered in the same genogroup showed identical nucleotide sequences but were collected from different parrot species. This was similar to two Portuguese APV isolates (PT25528 and PT919), which had identical nucleotide sequences despite being isolated from a green-winged macaw and an Eclectus parrot, respectively (Henriques et al., Citation2018). In contrast, in this study, the two strains (20AD031 and 20AD047) obtained from the same parrot species had different nucleotide sequences and belonged to different genogroups. These results imply that the strains might share a common origin regardless of the parrot species.
Gross and microscopic lesions were observed in only two of the five parrots infected by genetically identical South Korean strains; both birds were 2 months of age. Pathological lesions observed in these birds included hepatomegaly, yellow necrotic spots in the liver, subcutaneous haemorrhage in the neck and abdomen, and ascites within the peritoneal cavity. Intranuclear inclusion bodies, one of the typical lesions found in APV-infected parrots (Magdalena et al., Citation2011; Alley et al., Citation2013), were also observed in the liver and the kidney. Microscopic examination showed enlarged nuclei in the hepatocytes, hepatic necrosis, and bile duct proliferation in the liver. The findings are consistent with the pathology studies of APV infection described in previous papers (Bernier et al., Citation1981; Magdalena et al., Citation2011). Young parrots are more susceptible to APV infection than older parrots, suggesting that the appearance of pathological lesions might be associated with the age of infected parrots.
South Korean strains demonstrated high homology, regardless of host species or the ability to cause pathological lesions, although there were nucleotide differences that resulted in amino acid changes. The VP4 and VP4d proteins of APV have been proposed as pathogenic factors because they are involved in the induction of apoptosis and inhibition of IFN- β promoter activation. Thus, variations in these proteins might be associated with acute APV disease (Johne et al., Citation2000; Citation2007; Ma et al., Citation2019). However, the present study found that not all five genetically identical strains cause similar pathological lesions. VP1 interacts with VP2/VP3, which is involved in nuclear localization for viral assembly (An et al., Citation1999). Glycine at position 221 in VP2 may affect the nuclear localization signal or the role of VP1 transferring to the nucleus (Stoll et al., Citation1994). All eight South Korean strains characterized in this study had glycine at this position. Strains belonging to genogroup I are predominant among currently circulating APV. We found amino acid substitutions in several proteins from APV strains within this genogroup. These variations reveal that the virus continues to evolve genetically and suggest the possible emergence of new APV lineages, which may affect the magnitude and severity of new epidemics.
In conclusion, we confirmed that APV strains with identical sequences collected from different parrot species showed differences in pathogenicity, which were not related to the host species but did appear to be host age-dependent. The genetic similarity among the APV strains analyzed was very high, but some variations were found. Phylogenetic analysis indicated that two APV genogroups are prevalent in psittacine birds in South Korea. The high mortality rate of APV infection is likely to pose a threat to the field of companion birds, including parrots. Appropriate measures such as compliance with the customs clearance process and maintenance of clean breeding environments are needed to reduce the risk of virus transmission. Moreover, to prevent the spread of uncontrolled pathogen in the vulnerable sector of pet birds, training of operators of the ornamental bird sector is needed, as well as an improvement of the diagnostic services.
Disclosure statement
No potential conflict of interest was reported by the authors.
Additional information
Funding
References
- Adiguzel, M.C., Timurkan, M.O. & Cengiz, S. (2020). Investigation and sequence analysis of avian polyomavirus and psittacine beak and feather disease virus from companion birds in eastern Turkey. Journal of Veterinary Research, 64, 495–501.
- Alley, M.R., Rasiah, I., Lee, E.A., Howe, L. & Gartrell, B.D. (2013). Avian polyomavirus identified in a nestling Gouldian finch (Erythrura gouldiae) in New Zealand. New Zealand Veterinary Journal, 61, 359–361.
- Altan, E., Eravci, E., Cizmecigil, U.Y., Yildar, E., Aydin, O., Turan, N., Ozsoy, S., Tekelioglu, K.B., Kurt, T., Gerbaga Ozsemir, K., Altan, E. & Yilmaz, H. (2016). Detection and phylogeny of beak and feather disease virus and avian polyomavirus in psittacine pet birds in Turkey. Journal of Exotic Pet Medicine, 25, 280–287.
- An, K., Smiley, S.A., Gillock, E.T., Reeves, W.M. & Consigli, R.A. (1999). Avian polyomavirus major capsid protein VP1 interacts with the minor capsid proteins and is transported into the cell nucleus but does not assemble into capsid-like particles when expressed in the baculovirus system. Virus Research, 64, 173–185.
- Bernier, G., Morin, M. & Marsolais, G. (1981). A generalized inclusion body disease in the budgerigar (Melopsittacus undulatus) caused by a papovavirus-like agent. Avian Diseases, 25, 1083–1092.
- Bozeman, L.H., Davis, R.B., Gaudry, D., Lukert, P.D., Fletcher, O.J. & Dykstra, M.J. (1981). Characterization of a papovavirus isolated from fledgling budgerigars. Avian Diseases, 25, 972–980.
- Davis, R.B., Bozeman, L.H., Gaudry, D., Fletcher, O.J., Lukert, P.D. & Dykstra, M.J. (1981). A viral disease of fledgling budgerigars. Avian Diseases, 25, 179–183.
- Dayaram, A., Piasecki, T., Chrzastek, K., White, R., Julian, L., van Bysterveldt, K. & Varsani, A. (2015). Avian polyomavirus genome sequences recovered from parrots in captive breeding facilities in Poland. Genome Announcements, 3.
- Heenemann, K., Sieg, M., Rueckner, A. & Vahlenkamp, T.W. (2015). Complete genome sequence of a novel avian polyomavirus isolated from Gouldian finch. Genome Announcements, 3.
- Henriques, A.M., Fagulha, T., Barros, S.C., Ramos, F., Duarte, M.D., Luís, T., Carvalho, P., Mendonça, P., Monteiro, M., Craveiro, H. & Fevereiro, M. (2018). Aves polyomavirus 1 in Ara chloroptera and Eclectus roratus with disclosure of full genomic sequences. Journal of Exotic Pet Medicine, 27, 4–10.
- Hu, X., Cai, D., Liu, S., Li, Y., Chen, L., Luo, G., Pu, H., He, Y., Liu, X., Zhao, L., Cao, H., Yang, T. & Tian, Z. (2022). Molecular characterization of a novel budgerigar fledgling disease virus strain from budgerigars in China. Frontiers in Veterinary Science, 8, 813397.
- Johne, R., Jungmann, A. & Muller, H. (2000). Agnoprotein 1a and agnoprotein 1b of avian polyomavirus are apoptotic inducers. Journal of General Virology, 81, 1183–1190.
- Johne, R. & Muller, H. (1998). Avian polyomavirus in wild birds: genome analysis of isolates from Falconiformes and Psittaciformes. Archives of Virology, 143, 1501–1512.
- Johne, R., Paul, G., Enderlein, D., Stahl, T., Grund, C. & Müller, H. (2007). Avian polyomavirus mutants with deletions in the VP4-encoding region show deficiencies in capsid assembly and virus release, and have reduced infectivity in chicken. Journal of General Virology, 88, 823–830.
- Katoh, H., Ogawa, H., Ohya, K. & Fukushi, H. (2010). A review of DNA viral infections in psittacine birds. Journal of Veterinary Medical Science, 72, 1099–1106.
- Katoh, H., Ohya, K., Une, Y., Yamaguchi, T. & Fukushi, H. (2009). Molecular characterization of avian polyomavirus isolated from psittacine birds based on the whole genome sequence analysis. Veterinary Microbiology, 138, 69–77.
- Kim, H.J., Lee, S.R. & Park, C.K. (2014). First detection of avian polyomavirus by PCR from Alexandrine Parakeet (Psittacula eupatria) in Korea. Korean Journal of Veterinary Service, 37, 213–218.
- Kim, S., Kim, S.J. & Na, K.J. (2022). Molecular characteristics of budgerigar fledgling disease polyomavirus detected from parrots in South Korea. Journal of Veterinary Science, 23, e67.
- Kim, S.H., Kwon, Y.K., Park, C.K. & Kim, H.R. (2021). Identification of Campylobacter jejuni and Chlamydia psittaci from cockatiel (Nymphicus hollandicus) using metagenomics. BMC Genomics, 22, 797.
- Liu, F.L., Chang, S.P., Liu, H.J., Liu, P.C. & Wang, C.Y. (2022). Genomic and phylogenetic analysis of avian polyomaviruses isolated from parrots in Taiwan. Virus Research, 308, 198634.
- Ma, J., Wu, R., Tian, Y., Zhang, M., Wang, W., Li, Y., Tian, F., Cheng, Y., Yan, Y. & Sun, J. (2019). Isolation and characterization of an Aves polyomavirus 1 from diseased budgerigars in China. Veterinary Microbiology, 237, 108397.
- Magdalena, S., Koodziejska, A., Szarek, J., Babinska, I., Szweda, M., Kołodziejska, A., Szarek, J. & Babiñska, I. (2011). Avian polyomavirus infections in Amazon parrots. Medycyna Weterynaryjna, 67, 147–150.
- Moens, U., Calvignac-Spencer, S., Lauber, C., Ramqvist, T., Feltkamp, M.C.W., Daugherty, M.D., Verschoor, E.J., Ehlers, B. & ICTV Report, Consortium (2017). ICTV virus taxonomy profile: polyomaviridae. Journal of General Virology, 98, 1159–1160.
- Padzil, F., Mariatulqabtiah, A.R. & Abu, J. (2017). Avian polyomavirus: a recent update. Jurnal Veterinar Malaysia, 29, 9–13.
- Phalen, D.N., Wilson, V.G. & Graham, D.L. (1991). Polymerase chain reaction assay for avian polyomavirus. Journal of Clinical Microbiology, 29, 1030–1037.
- Riaz, A., Yousaf, A., Moaeen-Ud-Din, M., Shah, M.A.A., Zainab, T., Masood, S., Akhter, N. & Ali, A. (2019). First detection and molecular characterization of avian polyomavirus in young parrots in Pakistan. Veterinary Research Communications, 43, 197–202.
- Stoll, R., Hobom, G. & Muller, H. (1994). Host restriction in the productive cycle of avian polyomavirus budgerigar fledgling disease virus type 3 depends on a single amino acid change in the common region of structural proteins VP2/VP3. Journal of General Virology, 75, 2261–2269.
- Stoll, R., Luo, D., Kouwenhoven, B., Hobom, G. & Müller, H. (1993). Molecular and biological characteristics of avian polyomaviruses: isolates from different species of birds indicate that avian polyomaviruses form a distinct subgenus within the polyomavirus genus. Journal of General Virology, 74, 229–237.
- Tamura, K., Stecher, G. & Kumar, S. (2021). Mega11: molecular evolutionary genetics analysis version 11. Molecular Biology and Evolution, 38, 3022–3027.