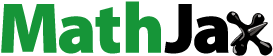
ABSTRACT
Autonomous and unmanned shipping are currently hot topics in the maritime industry. However, there are many different views on how the ultimate goal of an unmanned, autonomous ship will be reached. On any given ship, a large range of tasks is performed every day, each of which need to be replaced in such a way that no human presence is required on board. In this article, different possible combinations of tasks to be replaced are explored systematically, leading to an overview of the most beneficial combinations of tasks to replace together and a logical sequence in which to replace them. This leads to a plausible implementation path from low-manned ships towards fully unmanned autonomous ships.
1. Introduction
Over the last 10 years, there have been many companies, projects and consortia that have addressed unmanned autonomous shipping (Jokioinen et al. Citation2016; Tvete Citationn.d.; Skredderberget Citation2018; MUNIN Citation2016). However, currently no ships of this type are in operation. There are still several technical and economic challenges that need to be addressed, including an advanced automated navigation system and a propulsion system that requires no maintenance during the journey. There are several factors driving this research effort such as an increasing labour shortage in the maritime industry (BIMCO, and ICS Citation2015) and the competition between shipping companies. Additionally, it is believed that autonomous navigation will increase the safety of ships due to a decrease in the number of collisions (Porathe and Hoem Citation2018; Wróbel, Montewka, and Kujala Citation2017). It is still unknown when autonomous and unmanned ships will be able to enter service. The most optimistic predictions are that a first short sea cargo ship could commence commercial operation within 15 years (Levander Citation2017). However, one ship does not mean industry-wide implementation. This industry-wide implementation might take much longer or, as some believe, might never happen in certain areas of the maritime industry (Wienberg Citation2018).
In the last two years, several full scale trials have been completed. Rolls Royce has performed trials for autonomous navigation in the SVAN project (Rolls-Royce Citation2018). A consortium of Dutch companies performed trials for several navigation scenarios on the North Sea (Netherlands Maritime Technology Citation2019). These trials have shown that it is possible for a ship navigate without human intervention in normal situations. However, these tests are only the first steps on the road to reliable and safe unmanned autonomous ships. Many researchers, (e.g., Jokioinen et al. Citation2016; Batalden, Leikanger, and Wide Citation2017; Kobylinski Citation2018) have focussed on various parts of automated or autonomous navigation. Porathe (Citation2019) investigated some of the challenges that can arise in the communication between manned and unmanned ships. Investigations have also been performed into shore control stations for unmanned ships, e.g. (MacKinnon, Man, and Baldauf Citation2015; Levander Citation2017; Dybvik, Veitch, and Steinert Citation2020).
Autonomous navigation is not the only obstacle on the way to fully autonomous ships. In contrast to cars and aircraft, the operators on ships do much more than ‘just’ navigate the vehicle. For instance, the current propulsion units on ships are designed to rely on an engineering team on board to ensure reliable operation, cargo is checked and re-fastened underway and several crew members are required to moor the ship once it reaches port.
For maintenance of unmanned ships, the MUNIN project suggests that it must be performed while the ship is in port or during the period that the ship is in dry dock (Rødseth and Burmeister Citation2015). To decrease the possibility of failure while the ship is underway, some suggest increasing the redundancy of the main equipment or to change the maintenance policy (Rødseth and Burmeister Citation2015; Jalonen, Tuominen, and Whalström Citation2016). Additionally, others propose to step away from the traditional diesel engine as the main source of power (Rødseth and Burmeister Citation2015; Skredderberget Citation2018; Tvete Citationn.d.).
The journey of a ship consists of three distinct phases; arrival and departure, loading and unloading and sailing in open water. During these phases, varying levels of autonomy can be expected (Pietrzykowski and Hajduk Citation2019). This allows the transition between a manned vessel and an unmanned vessel to take place in parts as opposed to instantaneous. It is likely that there will be a transition phase in which changes are made to the ship which will allow it to operate with less human involvement. Much of the research effort is currently focussed on the end goal, i.e., a fully autonomous ship. However, much less is currently know about the logical transition path to reach this goal. Several tentative timelines have been developed; however, these do not consider the replacement of crew tasks in a detailed way (Rolls-Royce Citation2016; Lloyd’s Register, QineticQ, and University of Strathclyde Glasgow Citation2013). This article aims to remedy that shortcoming, thus providing a more well-founded transition path. To find this logical path, the focus of this research is on the technical and economic feasibility of implementing autonomous ships. It is often claimed that autonomous ships have other favourable qualities, such as improved safety and lower emissions. However, these aspects play a role in social and political acceptance of the concepts, which comes after technical and economic feasibility have been proven (in that order).
There are a large number of possible combinations of crew tasks that can be replaced on a ship to reduce the workload of the crew. Given the diversity of tasks that individual crew members perform, not all of these will actually result in a smaller crew or a cost reduction that will make the replacement option worthwhile. Thus, selecting the right set of tasks to replace is crucial.
This article presents the following:
An investigation of the intermediate phases between the conventionally manned ships in use now and the unmanned autonomous ships of the future
A systematic method of exploring the most beneficial combinations of tasks to replace
A plausible implementation path for low-manned ships towards a fully unmanned autonomous ship on a system and functional level
2. Method
The first step in exploring task removal options is combining the large number of, often small, crew tasks into clusters of tasks that can be automated or otherwise be removed from the ship with a single solution. Field research and consultations with industry experts have resulted in 10 such clusters. Next, scenarios are created which represent different situations in which one or more clusters are replaced. The analyses performed in this article use the Crew Analysis Algorithm that is elaborately described in Kooij and Hekkenberg (Citation2019) while a more general explanation of algorithms and assignment problems in general is given in Kooij and Hekkenberg (Citation2020). Furthermore it builds on research performed in Kooij and Hekkenberg (Citation2019) and Kooij and Hekkenberg (Citation2020) in which the impact of replacing crew tasks for specific tasks related to navigation and mooring of the ship is demonstrated. This algorithm uses the remaining tasks to determine the cheapest crew that can perform these tasks, expressed in terms of cost for the ship operator. The results of the CAA are used to find a plausible path towards an unmanned autonomous ship.
This section first discusses how the task clusters are developed. From there, it discusses which methods are used to set up scenarios, and which one is most suited for the goals of this article. Finally, the CAA is explained shortly.
2.1. Setting up task clusters
The analysis in this article is built on prior research on the functional breakdown and task distribution on board of a short sea container vessel (Kooij et al. Citation2018). The functional breakdown is based on a field study performed on board of the MV Endurance sailing between two European ports. The findings from the field study were corroborated and complemented by several industry experts, i.e., seafarers and teachers at maritime academies. This has resulted in a generally applicable task list. The list can be found in .
Table 1. THE 10 CLUSTERS IDENTIFIED WITH THE HELP OF INDUSTRY EXPERTS AND THE TASKS INVOLVED.
This list of tasks is subdivided in three travel phases: arrival & departure, loading & unloading and normal sailing. The tasks encompass everything that happens on board of the ship and are often composed of smaller subtasks. Since most tasks are closely related to other tasks, multiple tasks can be clustered together and then solved with a single solution. A group of industry experts has been asked to identify clusters of tasks that can be replaced with one solution. This has resulted in 10 clusters. These clusters are discussed in section 3.
The technical solutions for the identified task clusters are only explored if they have a technological readiness level (TRL) of 6 or higher. This means that a prototype of the system has been demonstrated in the proper environment (European Union Citation2016). By only using such proven technologies it can be assumed that the scenarios are at least possible in the near future (i.e., within 25 years). This also helps enable the estimation of the cost of selected solutions, which is a crucial element in market uptake of unmanned ships.
Not all of the identified task clusters will be automated. In some cases a person on shore is more suited to perform the task. For example, it is not currently feasible to use a robot that is capable of performing all maintenance on board. Allowing several engineers on board when the ship is in port, combined with a system design that minimizes required maintenance, is a more plausible alternative.
2.2. Setting up scenarios
With the tasks and logical clusters defined, the next step is to set up the scenarios that are analysed in this article. With 10 clusters, there are different combinations of remaining and replaced tasks possible. These combinations are the scenarios referred to in this article. If different methods to replace tasks are taken into account, the number of potential scenarios grows even further. As a result, assessing various options is not straightforward and the approach should be selected with care.
There are three distinct methods to set up scenarios: deductive, inductive and normative (Shell International BV Citation2008). In the deductive method, two critical uncertainties are identified. These two uncertainties are put on a two-axis system which results in a four quadrant matrix, in which each of the four quadrants represents a scenario (Ogilvy and Schwartz Citation2004). The deductive method is most suited for cases where it is possible to define two dominant factors that are both highly uncertain (Shell International BV Citation2008; Heijden Citation2005). For inductive scenario building, a chain of events is set up that create a plausible storyline for the future. These chains are built around a ‘What if’ question (Ogilvy and Schwartz Citation2004). Together, this creates a chain of events that form a scenario (Shell International BV Citation2008). The normative method is similar to the inductive method. This method works with an ‘Official future‘, a final scenario somewhere in the future. The different paths towards this goal are investigated. This method is more systematic than the inductive method described above (Ogilvy and Schwartz Citation2004). This research uses the normative method because the final situation is known (completely unmanned autonomous ships).
To answer the ‘What if’ questions, the CAA (explained below in section 2.3) is used to calculate the reduction in crew for any given scenario. It is impossible to select scenarios based on only the crew reduction, as there are too many options that wield the same result. The following steps are taken to determine the order in which the clusters are replaced.
The clusters that are to be removed all take place in the normative phase of the voyage (i.e., the phase that requires the most crew members). Removing crew members from another phase will not lead to a reduction of the required crew.
The economic impact of the clusters is investigated. Scenarios will only be considered if there is potential for an economic benefit for the ship owner.
Of the remaining clusters, the replacement options with the highest TRL or the shortest time to maturity time are selected.
If there are still multiple options, the cluster with the highest impact on the size of the crew is selected.
2.3. Determining the required crew composition for the scenarios
For each of the scenarios, the required crew composition needs to be determined. To do this, a purpose-built Crew Analysis Algorithm (CAA) is used. The CAA is based on a greedy algorithm that assigns tasks to differently skilled crew members. The CAA is discussed in detail in Kooij and Hekkenberg (Citation2019), a short summary is given here.
shows a simplified version of the algorithm. The algorithm starts with a list of all the tasks, sorted from most expensive to perform to cheapest (1). Here, the most expensive task is selected. The algorithm first checks if a crew member that is capable of performing a task (both in skill and in time) is available (2). If one or more crew members are available, the most expensive (i.e., the highest trained) crew member that still has sufficient time available is selected to perform the task (3). This ensures that crew members perform tasks that are as close as possible to their level of training. If there are no crew members capable of performing the task, a new crew member is created (4). This crew member is the cheapest possible crew member that can perform the task. This is possible due to the fact that the tasks are sorted by their maximum cost. This means that there will not be another task later on in the process that requires a more expensive crew member. Once a crew member is selected, the task is assigned (5). The available working time of the crew member involved is updated and the task is assigned to their roster. From here, the algorithm moves on to the next task until all tasks are assigned and the final required crew composition is determined.
The output of the CAA includes an overview of the required crew per travel phase as well as the total crew for the entire voyage, their workload per travel phase, and the cost of the crew. This process is repeated until all 1024 scenarios have been analysed.
2.3.1. Crew members involved
To determine the cost of the crew per travel phase, a monthly cost per crew member is used, based on information from MV Endurance’s operator. The cost can be found in . This cost is comparable to the cost used by Ghaderi (Citation2019) and is, therefore, deemed reasonable for other ships of similar type and size as well. The total crew cost for the ship owner is twice as high as presented in , since a ship is typically crewed by two complete crews, that each operate the ship for half the year. This means that the total crew cost is €97,800 per month.
Table 2. MONTHLY COST PER CREW MEMBER AND FOR THE TOTAL CREW IN A CONVENTIONAL SITUATION [CREW COST AND CREW COMPOSITION PROVIDED BY JR SHIPPING].
3. Elaboration of task clusters
As mentioned above, 10 clusters have been established through a field study and discussions with industry experts (see ). Below, each of the clusters and potential ways to replace them are discussed.
3.1. Cluster 1: mooring
The mooring task requires a significant number of crew members to be safe for all involved. In this cluster the tasks that take place on deck are covered, but the manoeuvring of the ship during mooring is not. That task is covered under the navigation cluster. Mooring is a dangerous procedure and is therefore tightly supervised by high ranking personnel. It is performed in three pairs to increase the safety of the crew involved.
There are several existing automatic mooring systems (Cavotec Citation2017; Mampaey Offshore Industries Citationn.d.). These systems are shore-based, which would require infrastructure adaptations in all ports that the ship visits. This would make implementation complex and expensive. Ideally, the ship would use an automated system that interfaces with the current infrastructure in ports (i.e., classic bollards). At this moment, no working prototype of such a system exists. However, one such solution has been suggested for use on the Yara Birkeland (MacGregor Citation2019). An alternative option for this is to take a shore crew on board to perform the mooring procedure.
3.2. Cluster 2: navigation
The task cluster navigation covers the tasks that are performed on the bridge during normal sailing and the arrival and departure phase. The most common solution suggested for navigation is to design a system that can perform the navigation (and all tasks connected with this) autonomously. In all cases, there is a human as back-up, in most cases on a shore control station.
However, a fully manned shore control station might not be required for a ship that is still manned. If some of the remaining crew members have basic navigation skills, they could bring the ship to safety should the autonomous system fail. Near ports, the ship could be sailed by experienced pilots, who are taken on board. This would significantly lower the requirements for the navigation system, both in terms of capabilities and in term of robustness, thus providing a workable solution until a more advanced system is available.
The requirements for the navigation system are the most strict during the arrival and departure phase, when the ship is sailing in narrow, shallow and busy waters. Out in open sea, during the normal sailing phase, there is more space for manoeuvring, and less traffic. It is likely that the navigation during the normal sailing phase is automated well before the navigation during the arrival and departure phase.
3.3. Cluster 3: engine room maintenance
The engine room maintenance cluster contains both the planned and unplanned maintenance that is performed in the engine room. In addition to the maintenance in the engine room, maintenance of other key components such as mooring and anchor winches is also included. Currently, maintenance of vital engine components is a non-stop process that starts almost as soon as the engine is started. The engine room can be left unattended for several hours. However, discussions with seafarers have revealed that the number of malfunctions is still far too high to enable a ship to sail reliably without engineers. While there are several solutions to improve reliability of the main machinery, e.g., several generator sets instead of one main engine or two main engines (Rødseth and Burmeister Citation2015) or a more steady state propulsion system such as a fuel cell or batteries (Kongsberg Citationn.d.; Tvete Citationn.d.), maintenance on these components is still required. This maintenance can be performed while the ship is in port.
During this time, a maintenance crew will come aboard to perform all required maintenance. The maintenance crew needs to be aware of any repairs that need to be performed in addition to the standard scheduled maintenance. For this reason, the technical equipment needs monitoring while the ship is in transit. It also means that on shore, someone needs to analyse this data to determine which repairs are required.
3.4. Cluster 4: maintenance on deck
The maintenance on deck consists of: cleaning and maintenance of the superstructure and the hull, maintenance of the hatch covers and the safety equipment, and any repairs required on this equipment. Normally, these tasks are performed during sailing; however, these works do not need to be done continuously. A similar solution as for the engine room maintenance can be set up for this type of maintenance. In port, a maintenance team can go aboard to clean the ship and to repair whatever is necessary.
3.5. Clusters 5: bunkering, 6: administration and 7: port supervision
Clusters 5, 6 and 7 have little in common with each other but can be solved using a similar solution. The bunkering process is performed mostly by staff of the bunkering company, while a crew member assists them with the bunkering and ensures that the fuel is loaded correctly. Both during loading and unloading and during the normal sailing phase of the ship several important administration tasks need to be performed. The loading plan for the new journey needs to be checked, bills of lading need to be signed, customs needs to be cleared and some crew administration, needs to be performed. Currently, many of these tasks are performed by either the captain or the chief officer.
Finally, there is a task cluster port supervision. This cluster encompasses the support to people coming onto the ship. This could for example be an external engineer assisting with repairs on the ship. Additionally, this task cluster entails ensuring that the ship is safe in port, supervision of the loading process and ensuring that the mooring lines stay secure and tight.
The most logical solution for all of these clusters is not a technical one. While many of the individual aspects of these tasks could be automated, it is highly likely that a person performing these tasks will be cheaper and equally effective.
Additionally, from expert interviews it was found that ship owners want to have control over who enters their ship. For most of the activities mentioned above, external personnel need access the ship. It would be an unprecedented leap of faith for commercial shipping to relinquish this control by the owner. At this point, it seems unlikely that this will be the case. Therefore, an agent to represent the ship and its owner is deemed a logical solution. Since it is already common to have an agent in destination ports, this is not considered a major challenge.
3.6. Cluster 8: responsibility
While on the ship, the captain has final responsibility for the ship. This means that they make the final decisions and can ultimately be held responsible for things that go wrong. On an unmanned autonomous ship, there is no ultimate responsibility on board. This is currently not allowed by law (International Maritime Organization Citation2000) but, if a person on shore can be provided with sufficient situational awareness and capability to intervene, there is no fundamental reason why this responsibility cannot be taken on shore. The same goes for the responsibility of the chief engineer in the engine room.
It is possible that the captain is no longer required on board, except to hold responsibility over the ship. By using a so-called marof, an officer trained as an engineer and a nautical officer, the responsibility can be moved to this crew member. That way it is possible to combine two roles, making it more likely that the crew size can be reduced.
3.7. Cluster 9: cargo conditioning
The movement and vibrations of the ship can cause problems, such as causing the lashings of containers to loosen. To prevent stacks of containers from falling over, the lashings need to be refastened during the voyage. This is done by the deck crew, who check them at least once per day. By equipping the ship with above-deck cell guides, as opposed connecting the containers with twistlocks and lashings, the containers will remain stable on the ship.
3.8. Cluster 10: life support
This cluster encompasses two tasks that are vital for the survival of persons on the ship; preparation of food and provision of medical care. Automating medical care is not possible. While there are possibilities for automation within the food production industry, these options to not seem feasible on board of a ship. As long as there are humans on board of the ship, both these tasks need to be performed. However, it is to be expected that at some point, the ship will, no longer have a dedicated cook. The medical care will be required as long as there are crew members on board. This means that a crew member always requires some medical training.
3.9. Summary of solutions
In , the solutions mentioned in this section are summarised. One of the elements that is used to determine which cluster to automate is the implementation time. Therefore, an indicative time frame is given in which it is expected that this solution will be available. This timeframe is an indication and is based either on the TRL of the technical solution, or the number and type of changes suggested for an organisational solution.
Table 3. SUMMARY OF THE PRESENTED SOLUTIONS AND THE EXPECTED IMPLEMENTATION TIME OF THE SOLUTIONS.
3.10. Required crew members per cluster
shows how many crew members from each department (i.e., bridge, engine room and deck) are involved in performing the tasks that make up each of the clusters. The table shows that in many cases crew members from different departments are involved. Additionally it shows that a number of clusters occur in multiple travel phases. The information presented in plays a key role in determining logical paths towards unmanned autonomous ships. The information is used to select subsequent clusters to automate, based on the order defined in section 2.
Table 4. REQUIRED CREW MEMBERS PER CLUSTER, SORTED BY TRAVEL PHASE PER DEPARTMENT [OUTPUT FROM AUTHOR’S PUSPOSE BUILT CREW ANALYSIS ALGORITHM].
4. Results
By calculating the required crew in all scenarios, it is possible to get a complete overview of the effects of the removal of all combinations of task clusters. The results are presented in a heat map. After a short introduction of the heat map, this analysis focusses on a plausible path to an unmanned autonomous ship using the normative method explained in section 2.1.1.
4.1. Preliminary analysis of task cluster replacement
shows the heat map for the required crew. The horizontal axis shows the crew that is required for a given scenario, while the vertical axis shows the number of clusters that are replaced. The numbers inside the heat map show how many different combinations of clusters there are that result in the same combination of number of replaced clusters and remaining number of crew members. For example, the column titled ‘8ʹ shows that there are 5 scenarios (out of a total of 346) that require 8 crew members when 2 clusters are replaced. On the top right of the figure the base-case scenario can be found, which has no automated clusters and 11 required crew members. On the bottom left, the final scenario can be found where all 10 clusters have been automated and no crew is required. The columns in between illustrate how many scenarios there are that require a specific number of crew members at a specific number of replaced clusters. In this figure, the maximum crew required for the trip (i.e., the crew required in the normative phase) is taken as the input. In some cases, this is the normal sailing phase, while in others it is the arrival and departure or the loading and unloading phase.
The heat map shows that there are many different ways to reduce the crew by a specific number of crew members. For example, there are 346 combinations of replaced task clusters to create a ship that requires 9 crew members. As another example, it is possible to find a scenario that requires 7 crew members by automating anywhere between 4 and 9 clusters. This also means that it is very easy to pick a ‘wrong’ scenario that does not have the ideal balance between investing in solutions and the decreasing the crew cost.
Currently, the crew of a ship is split up into three departments: the bridge department, the engine room department and the deck department. During the field study it was found that performing tasks from another department, or even from a crew member within the same department with a different rank, is not customary, even if a crew member has the required skill. A previous case study by Kooij and Hekkenberg (Citation2019) has shown that having the traditional task assignment inhibits the removal of crew members from the ship as it leads to a relatively large crew, often with a low workload for several crew members.
In the base scenario, there is no difference between the requirements for the traditional or the relaxed task assignment. However, for other scenarios, there is a difference. shows the difference in crew requirement between the strict and relaxed task assignment. This figure shows that the relaxed assignment is consistently more to the right, indicating that, on average, fewer crew members are required for each scenario and that there are more options to achieve any given crew reduction. This relaxed task assignment is therefore used in this article.
Figure 3. DIFFERENCE IN SCENARIO DISTRIBUTION (CUMULATIVE) SHOWING A SIGNIFICANT DIFFERENCE BETWEEN THE REQUIRED CREW FOR THE RELAXED ASSIGNMENT AND THE TRADITIONAL ASSIGNMENT.
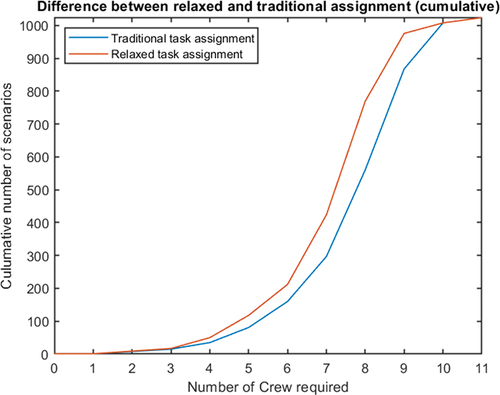
Instinctively, the topmost solution of each column of (as much crew reduced with as little replacement effort as possible) may seem like the best situation. However, this conclusion cannot be drawn from this map, as the cost of the replacement solution is not taken into account and it is not specified which crew members are removed (e.g., removing the captain saves more money than replacing two deck boys). Therefore, further analysis is required.
4.1.1. Crew cost
From the algorithm, it is also possible to determine the crew cost for each of the scenarios. In the base case, the cost for the total crew is €100,600 per month. For the scenarios to be economically feasible, the cost savings from a smaller crew must be equal to or larger than the investment and operating cost of the replacement solutions. shows the crew cost as a function of the number of clusters that are automated and the required crew respectively. The top row of the figure shows that there is a significant number of scenarios (124) that reduce the crew cost by a maximum of just over €10,000 per month, even if up to 6 clusters are replaced. This does not provide a significant budget to implement alternative solutions for the task clusters that are to be replaced. The figure also shows that each of the 10 options in which only one cluster is automated falls within this category. It is therefore very likely that only automating one cluster does not allow for enough reduction in crew cost to be feasible.
Figure 4. HEAT MAP FOR MONTHLY CREW COST PER SCENARIO AS A FUNCTION OF THE NUMBER OF CLUSTERS AUTOMATED.
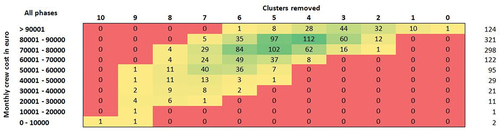
Again, there are many different ways that a specific cost range can be reached. In line with the findings above, very few conclusions regarding which scenario is best can be drawn from , apart from the fact that automating a single task cluster will not lead to major cost savings and that there is no clear single best way forward. For such insight, it is necessary to analyse the effect of replacing individual task clusters in more detail.
4.2. Reducing the crew size
As discussed in the previous section, it is not straightforward to determine which scenario is the best option. Even if all of the investment and operational costs required to replace the crew’s role in a given task cluster are known, there are other factors to consider, such as time until technical maturity of a solution and regulations. Therefore, the normative method, as introduced in section 2, is used to find a plausible path towards an unmanned and autonomous ship. As a reminder, to determine which cluster to remove, the following steps are taken:
Find the normative phase
Find clusters that have an economic impact
Find the cluster with highest TRL or shortest implementation time
Find the cluster with the highest impact on crew size
The cluster that emerges is replaced and the process is repeated. To do this, the information provided in and is used. Following these steps leads to the results presented in .
Table 5. COMPREHENSIVE SUMMARY OF THE REQUIRED NUMBER OF CREW MEMBERS PER REDUCTION STEP [OUTPUT FROM AUTHOR’S PURPOSE BUILT CREW ANALYSIS ALGORITHM].
The required crew in the conventional situation is: 11 crew members in the normal sailing phase, and 9 for the arrival and departure phase and the loading and unloading phase. This means that the first replacement of a task cluster will need to take place in the normal sailing phase. The normal sailing phase encompasses; navigation, maintenance in the engine room, maintenance on deck, administration, responsibility, cargo conditioning and life support. Maintenance on deck, administration and cargo conditioning could all be replaced without further technological development; however, it has not been done. This is attributed to the lack of an economic benefit to moving these tasks ashore. Along the same lines, there is no economic benefit to moving responsibility ashore, while the captain and chief engineer remain on board. Life support cannot be replaced while a crew remains. This leaves navigation and maintenance in the engine room. Of these two, the navigation cluster has a higher TRL and shorter implementation time. Therefore, the navigation cluster will be removed first. In section 3, two different solutions for automating the navigation cluster were suggested. In this case, the first, simpler, solution is used where only the navigation during the normal sailing phase is automated.
This first reduction leads to two new normative phases, loading and unloading and arrival and departure, as can been seen in . For the loading and unloading phase, port supervision is selected to be replaced. This cluster is easy and relatively cheap to implement. For arrival and departure, the mooring cluster is replaced, as it has the highest TRL. As discussed in section 3, mooring can be replaced by either a shore crew, or a fully automatic system. As a shore crew is likely to be a cheaper and simpler option, this solution is selected.
As per , the normative phase is now normal sailing again, which requires 8 crew members. Out of the remaining clusters, maintenance on deck, administration and cargo conditioning have the shortest time to availability. These solutions can all be implemented with relative ease by a shore crew. While aiming to reduce the required crew during the normal sailing phase, the crew in the loading and unloading phase is also decreased, as administration and maintenance on deck also take place in this phase.
The crew has now been reduced to a maximum of 4 crew members. (left) shows the crew members that remain. The first thing to note is the fact that the ship still has a full time cook on board. This seems excessive for such a small crew. The right side of shows that when it is assumed that all crew members have the skill to perform the task prepare food and drink and the required time for this task is reduced to 3 hours, a cook is no longer required.
Figure 5. LEFT: WORKLOAD FOR THE REMAINING 4 CREW MEMBERS FOR EACH OF THE TRAVEL PHASES, RIGHT: WORKLOAD WITH OTHER CREW MEMBERS HAVING THE CAPABILITY TO PREPARE FOOD.
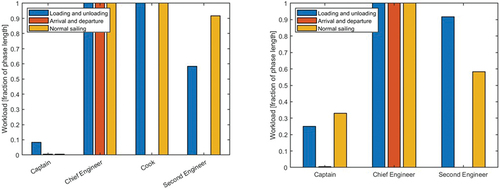
At this point, only a few clusters of tasks remain. These are; maintenance in the engine room, responsibility, bunkering, life support and the near shore sailing part of the navigation cluster. There are two normative phases, loading and unloading and normal sailing, each of which require 3 crew members. The cluster in the loading and unloading phase that is easiest and most likely the cheapest to replace is the bunkering, especially since several other clusters have already been moved to shore. Therefore, this cluster is selected to be replaced. To reduce the size of the crew in the normal sailing phase, the remaining part of the navigation cluster is automated. It is believed that the technology to do this will be available sooner than a propulsion system that will not require human interaction. In order to fully remove the captain from the ship, the responsibility task is replaced as this is the captain’s one remaining task on board. This also ensures that, should the navigation system break down, the marof is capable of navigating the ship.
This means that a very small crew of 2 engineers remains. To make the final step in the crew reduction process, the maintenance in the engine room cluster needs to be replaced. Additionally, the regulations should allow someone to be responsible for a ship from a distance. If these two challenges are solved, the final remaining cluster, life support, is also no longer required. It should be noted that this last step is very expensive, since it requires the implementation of an elaborate shore control station as well as a new, expensive, power plant on board the ship.
5. Discussion
In this article, a logical path towards unmanned autonomous ships has been determined. However, is not the only possible solution. However, since the order of removal of the clusters is dictated by the moment the solutions are available for replacement, there are a limited number of other logical paths which are not likely to differ significantly from the one suggested in this article. One possible difference might be not to start with automating the navigation tasks in the normal sailing phase but to elect to start with replacing cargo conditioning, maintenance on deck and administration because they do expect economic benefits from replacing these clusters, which is possible today.
The required number of crew members is reduced to two, before the final step towards unmanned ships is taken. However, it should be investigated if this is a desirable situation with regards to the crew’s mental and physical safety. It is very possible that the final two steps (i.e., replacing near shore navigation, bunkering, and maintenance in the engine room) may be taken together.
The method described in this article is capable of analysing a large number of scenarios. In this paper, the method has been used only to analyse the size of the crew and the number of clusters automated. While valid conclusions can be drawn from this analysis, it does not paint the full picture. A ship will only be built if it is economically viable and at least costs the same, or less, than a conventional ship in operation. This article uses the estimated economic benefit of solutions, based on logical reasoning. However, a more detailed economic analysis will shed further light on how and when reduction steps are possible.
In that case, not only the crew cost need to be taken into account, but also cost savings due to the fact that the crew is no longer on board. On a ship without a crew, several systems, such as heating, ventilation and air conditioning (HVAC) systems, plumbing and the entire superstructure, are no longer required, which could decrease the building cost. The on-board crew cost will reduce by an estimated €97.800 per month. Frijters (Citation2017) has estimated that no longer having a crew on board would save between €1.5 and €2 million in building cost. That would mean that this money is also available for replacement solutions.
To be able to make a good estimation of the cost, further investigation of the costs of all the solutions is required. The exact number of personnel required to form the shore crew, as well as the investment cost (CAPEX) for the technical replacements needs to be determined. With these numbers it is possible to identify which of the intermediate steps described in this article are economically feasible, and which ones will be skipped because they are not. Only with a full investigation of the economics is it possible to determine if unmanned autonomous ships are economically feasible. .
6. Conclusions
In this article, a purpose-built crew analysis algorithm is used in combination with the normative scenario method to find a likely path towards unmanned and autonomous ships. From this research, a number of conclusions can be drawn.
The first conclusion is that with the number of scenarios and the number of scenarios that result in the same number of required crew, it is very important to choose tasks to replace with care. Replacing the wrong combination of clusters can result either in a very low reduction of the required crew or in significantly more costly solution than might be required. Therefore, selecting the correct order of clusters to automate requires careful consideration of the affected travel phase, the economic benefit to the ship owner, the TRL of the suggested solution, and finally the effect of the solution on the size of the crew. Making the wrong choice can have significant effects as it is possible to automate up to six clusters without removing a single crew member (see ).
The second conclusion that can be drawn is that letting go of the traditional task assignment, in which crew only perform tasks within their own department, reduces the required crew overall. Over the whole solution space the required number of crew members is lower if a more relaxed task assignment is used. By allowing crew members to perform tasks in other departments that they have the skill for, their occupation rate for a travel phase goes up, resulting in a lower crew requirement. However, this might also mean that a highly skilled crew member such as a captain or a chief engineer ends up performing duties such as cargo conditioning or maintenance of the superstructure. These tasks are well below their skill level which could negatively impact their work enjoyment.
The path presented here shows that there are only a few distinct steps on the path to unmanned autonomous sailing. The first step is taken when autonomous navigation outside of the busy waterways (i.e., during normal sailing) is possible. This allows for a small reduction in crew, saving €5,600 monthly. While other clusters can be replaced earlier, it is unlikely that this will happen, as it is not done today, implying that ship owners do not see any benefit in replacing these tasks. As soon as partially replacing navigation becomes possible, a large number of other clusters can be replaced as well, to significantly reduce the number of crew members required. Replacing mooring, deck maintenance, bunkering, administration and port supervision decreases the required crew to 4 crew members. Reworking the food preparation task to better suit such a small crew can further cut the required number of crew to 3 people. These three crew members are highly skilled and therefore expensive. Reducing the crew by 8 crew members only cuts the crew cost in half. Next, the near shore navigation, as well as engine room maintenance, responsibility and life support can be replaced.
There is a large technology gap between having 3 crew members on board and having no crew members on board. Keeping a captain, a chief engineer and a second engineer on board means that the navigation system can be less complex and redundant as these crew members can step in if required. The difficult task of building an autonomous navigation system that can handle sailing in ports can be postponed and a complex and expensive shore control station is not required yet. The same goes for the maintenance in the engine room. Having two engineers on board means that the ship can continue to sail with the current drive train, which significantly decreases development cost compared to more solid-state, robust and low maintenance solutions. Finally, the ship will continue to meet the manning regulations which state that a captain must be on board and hold responsibility.
The most challenging cluster to replace is maintenance in the engine room. Conventional diesel engines are not designed to function without maintenance for more than a few hours. Further increasing the performance of the diesel engine will be costly. New propulsion systems, such as fuel cells, have fewer moving parts and therefore less maintenance. Additionally, these propulsion systems have the added benefit of emitting far fewer greenhouse gasses than a diesel engine of the same power. It is likely that the development of the autonomous ship will go hand in hand with the development of the green ship.
Disclosure statement
No potential conflict of interest was reported by the author(s).
Additional information
Funding
References
- Batalden, B., P. Leikanger, and P. Wide. 2017. “Towards Autonomous Maritime Operations.” In International Conference on Computational Intelligence and Virtual Environments for Measurement Systems and Applications (CIVEMSA), 1–6. Annecy, France. https://doi.org/10.1109/CIVEMSA.2017.7995339.
- BIMCO, and ICS. 2015. “Manpower Report Executive Summary 2015.” http://www.ics-shipping.org/docs/default-source/resources/safety-security-and-operations/manpower-report-2015-executive-summary.pdf?sfvrsn=16.
- C. Kooij and R. Hekkenberg, 2020. The effect of autonomous systems on the crew size of ships – a case study. Maritime Policy & Management. doi:10.1080/03088839.2020.1805645
- Cavotec. 2017. MoorMaster TM Automated Mooring Systems. 2017. Lugano, Switzerland: Cavotec.
- Dybvik, H., E. Veitch, and M. Steinert. 2020. “Exploring Challenges with Designing and Developing Shore Control Centers (Scc) for Autonomous Ships.” In Proceedings of the Design Society: DESIGN Conference, 1:847–856. https://doi.org/10.1017/dsd.2020.131.
- European Union. 2016. Horizon 2020 Workprogram Annex G - Technology Readiness Levels.
- Frijters, T. 2017. Future Ships - Design and Cost Analysis of Unmanned Ships. Delft, The Netherlands: Delft University of Technology.
- Ghaderi, H. 2019. “Autonomous Technologies in Short Sea Shipping: Trends, Feasibility and Implications.” Transport Reviews 39 (1): 152–173. doi:10.1080/01441647.2018.1502834.
- Heijden, K, van der. 2005. Scenarios - The Art of Strategic Conversation. 2nd ed. John Wiley & Sons, Ltd
- International Maritime Organization. 2000. “Principles of Safe Manning - IMO Resolution A.890 (21).” https://doi.org/10.1126/science.202.4366.409.
- Jalonen, R., R. Tuominen, and M. Whalström. 2016. Safety and Security in Autonomous Shipping - Challanges for Research and Development. London, England: AAWA.
- Jokioinen, E., J. Poikonen, A. Kolu, T. Jokela, J. Tissari, A. Paasio, H. Ringbom, et al. 2016. “Remote and Autonomous Ships: The Next Steps.” Ship Intelligence: Marine. http://www.rolls-royce.com/~/media/Files/R/Rolls-Royce/documents/customers/marine/ship-intel/aawa-whitepaper-210616.pdf.
- Kobylinski, L. 2018. “Smart Ships - Autonomous or Remote Controlled?.” Scientific Journals of the Maritime University of Szczecin-Zeszyty Naukowe Akademii Morskiej W Szczecinie 53 (125): 28–34. doi:10.17402/262.
- Kongsberg. n.d. “Autonomous Ship Project, Key Facts about YARA Birkeland - Kongsberg Maritime.” Accessed 16 October 2017. https://www.km.kongsberg.com/ks/web/nokbg0240.nsf/AllWeb/4B8113B707A50A4FC125811D00407045?OpenDocument.
- Kooij, C., M. Loonstijn, R. G. Hekkenberg, and K. Visser. 2018. “Towards Autonomous Shipping: Operational Challenges of Unmanned Short Sea Cargo Vessels.” In International Maritime Design Conference 2018, Helsinki, Finland.
- Kooij, C., and R. G. Hekkenberg. 2019. “Towards Unmanned Cargo-Ships : The Effects of Automating Navigational Tasks on Crewing Levels.” In COMPIT 2019 - Tullamore, Ireland, 104–117. Hamburg: Technische Universität Hamburg-Harburg.
- Levander, O. 2017. “Autonomous Ships on the High Seas.” IEEE Spectrum (3): 2017. doi:10.1109/MSPEC.2017.7833502.
- Lloyd’s Register, QineticQ, and University of Strathclyde Glasgow. 2013. Global Marine Trends Global Marine Trends.
- MacGregor. 2019. “Yara Birkeland.” Movie. 2019. https://www.youtube.com/watch?v=Co211gU_J5w.
- MacKinnon, S., Y. Man, and M. Baldauf. 2015. “D8. 8 : Final Report : Shore Control Centre.”
- Mampaey Offshore Industries. n.d. Intelligent Dock Locking System. Dordrecht: Mampaey offshore industries.
- MUNIN. 2016. “MUNIN | MUNIN– Maritime Unmanned Navigation through Intelligence in Networks.” 2016. http://www.unmanned-ship.org/munin/.
- Netherlands Maritime Technology. 2019. “First Autonomous Manoeuvring Vessel Trials Held on North Sea - Autonomous Shipping.” 2019. https://autonomousshipping.nl/2019/03/27/first-autonomous-manoeuvring-vessel-trials-held-on-north-sea/.
- Ogilvy, J., and P. Schwartz. 2004. “Plotting Your Scenarios.” Learning from the Future: Competitive Foresight Scenarios, 5–8. Emeryville, California, USA: Global Business Network. papers2://publication/uuid/DC3E008E-C279-4EA9-9179-BA131B10FF71.
- Pietrzykowski, Z., and J. Hajduk. 2019. “Operations of Maritime Autonomous Surface Ships.” TransNav 13 (4): 725–733. doi:10.12716/1001.13.04.04.
- Porathe, T., and Å. Hoem. 2018. “At Least As Safe As Manned Shipping? Autonomous Shipping, Safety And ‘ Human Error.” In Safety and Reliability – Safe Societies in a Changing World - ESREL 2018, edited by S. Haugen, A. Barros, C. Van Gulijk, T. Kongsvik, and J. E. Vinnem, 417–425. London, England: Taylor and Francis. https://doi.org/10.1201/9781351174664-52.
- Porathe, T. 2019. “Autonomous Ships and the COLREGS: Automation Transparancy and Interaction with Manned Ships.” In Compit 2019, edited by V. Bertram, 352–358. Hamburg: Technische Universität Hamburg-Harburg.
- Rødseth, Ø., and H. C. Burmeister. 2015. “D10.2 : New Ship Designs for Autonomous Vessels.” http://www.unmanned-ship.org/munin/wp-content/uploads/2015/10/MUNIN-D10-2-New-Ship-Designs-for-Autonomous-Vessels-MRTK-final.pdf.
- Rolls-Royce. 2016. “Autonomous Ships: The Next Step.” In AAWA: Advanced Autonomous Waterborne Applications, 7.
- Rolls-Royce. 2018. “Press Releases - Rolls-Royce and Finferries Demonstrate World’s First Fully Autonomous Ferry–rolls-royce.” 2018. https://www.rolls-royce.com/media/press-releases/2018/03-12-2018-rr-and-finferries-demonstrate-worlds-first-fully-autonomous-ferry.aspx.
- Shell International BV. 2008. Scenarios : An Explorer ’ s Guide. The Hague, The Netherlands: Shell Intenational BV.
- Skredderberget, A. 2018. “Yara Birkeland | The First Zero Emission, Autonomous Ship | Yara International.” Webpage. 2018. https://www.yara.com/knowledge-grows/game-changer-for-the-environment/.
- Tvete, H. A. n.d. “The ReVolt - A New Inspirational Ship Concept.” Accessed 16 October 2017. https://www.dnvgl.com/technology-innovation/revolt/index.html.
- Wienberg, C. 2018. “Maersk’s CEO Can’t Imagine Self-Sailing Box Ships in His Lifetime - Bloomberg.” Article. 2018. https://www.bloomberg.com/news/articles/2018-02-15/maersk-ceo-can-t-imagine-self-sailing-box-ships-in-his-lifetime.
- Wróbel, K., J. Montewka, and P. Kujala. 2017. “Towards the Assessment of Potential Impact of Unmanned Vessels on Maritime Transportation Safety.” Reliability Engineering and System Safety 165 (March): 155–169. doi:10.1016/j.ress.2017.03.029.